BIOL 408 Exam Study Guide PDF
Document Details
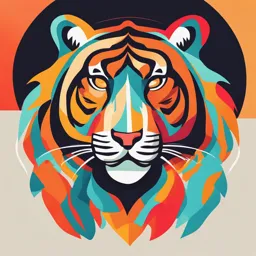
Uploaded by BelievableVigor1436
University of Hawaii at Mānoa
Tags
Summary
This document is a study guide for a biology course (BIOL 408) and covers topics such as biomembranes, transmembrane transport, cellular energetics, and photosynthesis.
Full Transcript
Chapter 10: Biomembrane Structure I. The Fluid Mosaic Model of Biomembranes Plasma Membrane (PM): ○ Composed of lipid bilayer, proteins, and carbohydrates. ○ Functions as a semi-permeable barrier, regulating the movement of substances. ○ Exhibits fluidity due...
Chapter 10: Biomembrane Structure I. The Fluid Mosaic Model of Biomembranes Plasma Membrane (PM): ○ Composed of lipid bilayer, proteins, and carbohydrates. ○ Functions as a semi-permeable barrier, regulating the movement of substances. ○ Exhibits fluidity due to lateral movement of lipids and proteins. Types of Membrane Proteins: ○ Integral (Transmembrane) Proteins: Span the bilayer. ○ Peripheral Proteins: Attach via non-covalent interactions. ○ Lipid-Anchored Proteins: Covalently bonded to lipids (e.g., GPI anchors). II. Composition of Biomembranes Lipid Bilayer: ○ Hydrophilic head groups face outward, hydrophobic tails face inward. ○ Cholesterol modulates fluidity and stability. Membrane Lipids: ○ Phospholipids: Glycerophospholipids (e.g., phosphatidylcholine, phosphatidylserine). ○ Sphingolipids: Sphingomyelin, glycolipids, ceramides. ○ Sterols: Cholesterol in animals, phytosterols in plants. Membrane Asymmetry: ○ Different lipid compositions in cytosolic vs. extracellular leaflets. ○ Flipases, Floppases, and Scramblases regulate lipid distribution. III. Membrane Properties and Dynamics Self-sealing and fusion properties: Enables vesicle formation, endocytosis, and exocytosis. Lateral diffusion: Movement of lipids/proteins within the membrane. Phase transition (gel → fluid state): Temperature-dependent changes in membrane fluidity. Lipid Rafts: ○ Rich in cholesterol and sphingolipids. ○ Specialized for signaling and trafficking. IV. Transport Across the Membrane Passive Transport: ○ Simple diffusion (O₂, CO₂, small lipophilic molecules). ○ Facilitated diffusion (via channels or transporters). Active Transport: ○ Requires ATP (e.g., Na+/K+ ATPase, Ca²+ ATPase). Endocytosis & Exocytosis: ○ Endocytosis: Phagocytosis (large particles), pinocytosis (fluids), receptor-mediated. ○ Exocytosis: Secretion of substances (e.g., neurotransmitters). Chapter 11: Transmembrane Transport of Ions and Small Molecules I. Overview of Membrane Transport Mechanisms Pumps (Primary Active Transport): ATP-powered, move molecules against gradients. Channels: Allow specific ion passage down electrochemical gradients. Transporters (Carriers): Conformational change-mediated transport. II. Types of Transporters Uniporters: Transport a single molecule down its gradient (e.g., GLUT1 for glucose). Symporters: Move two molecules in the same direction (e.g., Na+/glucose symporter). Antiporters: Move two molecules in opposite directions (e.g., Na+/H+ exchanger). III. ATP-Powered Transport Proteins P-Class Pumps: ○ Na+/K+ ATPase (pumps 3 Na+ out and 2 K+ in per cycle). ○ Ca²+ ATPase (sarcoplasmic reticulum, muscle contraction). V-Class Pumps: ○ Acidify organelles (lysosomes, endosomes) by pumping H+ inside. Opposite of F-Class. F-Class Pumps: ○ ATP synthase in mitochondria and chloroplasts (proton gradient-driven ATP production). ABC Transporters: ○ Multiple ATP binding domains to open channel ○ Multidrug resistance (MDR1), CFTR (cystic fibrosis chloride channel). IV. Ion Channels & Action Potentials Resting membrane potential (~ -70 mV): Set by Na+/K+ ATPase and K+ leak channels. Voltage-Gated Ion Channels: 1. Open/close in response to voltage changes (e.g., Na+, K+, Ca²+ channels). Action Potential: 1. Depolarization: Na+ channels open, Na+ influx. 2. Repolarization: K+ channels open, K+ efflux. 3. Refractory period: Na+ channels temporarily inactivated. Chapter 12: Cellular Energetics I. Overview of Cellular Respiration Aerobic Respiration: O₂ is final electron acceptor in the electron transport chain. Anaerobic Respiration: Alternative electron acceptors (e.g., sulfate, nitrate). Overall Equation: C₆H₁₂O₆ + 6 O₂ → 6 CO₂ + 6 H₂O + 30-32 ATP II. Stages of Glucose Oxidation 1. Glycolysis (Cytoplasm): ○ Glucose → 2 Pyruvate + 2 ATP + 2 NADH 2. Pyruvate Oxidation (Mitochondrial Matrix): ○ Pyruvate → Acetyl-CoA + NADH + CO₂ 3. Citric Acid Cycle (TCA Cycle): ○ Produces NADH, FADH₂, and ATP/GTP. 4. Electron Transport Chain & Oxidative Phosphorylation: ○ Generates proton gradient, driving ATP synthesis via ATP Synthase. III. Lipid and Protein Metabolism Fatty Acid Oxidation (Beta-Oxidation): ○ Acyl-CoA → Acetyl-CoA + NADH + FADH₂ Protein Catabolism: ○ Amino acids are deaminated, entering TCA cycle. IV. Photosynthesis 1. Light-Dependent Reactions (Thylakoid Membrane): ○ Photosystem II (P680) splits H₂O, producing O₂ and protons. ○ Photosystem I (P700) reduces NADP+ to NADPH. 2. Calvin Cycle (Stroma of Chloroplasts): ○ Fixes CO₂ and Ribulose 1,5-bisphosphate into glyceraldehyde-3-phosphate (G3P). i. Ribulose 1,5-bisphosphate to 3-phosphoglycerate 1. Enzyme: Rubisco ii. 3-phosphoglycerate to 1,3-bisphosphoglycerate iii. 1,3-bisphosphoglycerate to G3P ○ G3P can exit the chloroplast and enter the cytoplasm to make i. 1 molecule of glucose/fructose ○ 6 CO2 = 1 SIX CARBON SUGAR ○ REGENERATION OF 6 RIBULOSE 1,5-BISPHOSPHATE ○ USES 12 NADPH AND 18 ATP Chlorophyll a Structure & Function Core Structure: Porphyrin ring with a Mg²⁺ ion (instead of Fe²⁺ in heme) + hydrocarbon phytol tail for membrane anchoring. Chlorophyll a vs. b: Chl b has a –CHO (formyl) group instead of a –CH₃ group. Function: Major light-absorbing pigment in thylakoid membrane, bound to proteins in photosystems. Photosynthetic Pigments & Light Absorption Chlorophyll a (Chl a) absorbs red (680 nm) & blue light, driving photosynthesis. Chlorophyll b (Chl b) absorbs 650 nm light, extending the range. Carotenoids absorb shorter wavelengths & protect chlorophyll from damage. Phytochromes detect far-red light (705–740 nm) for photoreception. Photoelectron Transport (Primary Event in Photosynthesis) Light excites Chl a in the reaction center, donating an electron to a quinone (Q/PQ). Creates an irreversible charge separation across the thylakoid membrane. Plastoquinol (PQH₂) = reduced form, passes electrons to cytochrome b₆f complex. Chlorophyll a⁺ is neutralized by: ○ PSII: Water oxidation (H₂O → O₂ + 4H⁺ + 4e⁻). ○ PSI: Electron transfer from plastocyanin (PC). Light-Harvesting Complex (LHC) in Cyanobacteria Contains 90 chlorophyll molecules in an optimal geometric arrangement. Resonance energy transfer funnels absorbed light to special-pair Chl a in the reaction center. Redox Potential in Photosynthesis H₂O oxidation (O₂ evolution) requires 1.14 V but cells use >2 V due to non-standard conditions. Photon energy at 680 nm = 1.8 V, enough to reduce NADP⁺ to NADPH via electron transport. PSII & Water Splitting (Photolysis) P680 (reaction center of PSII) is the strongest biological oxidant. Splitting H₂O (O₂ evolution): ○ 2 H₂O → O₂ + 4H⁺ (lumen) + 4e⁻ (P680 oxidizes H₂O). ○ Requires 4 photons (one per electron). ○ Mn/Ca cluster in PSII accumulates 4 "+" charges before O₂ release. Electron Transport Pathway & Proton Gradient Electrons flow: H₂O → PSII → PQ → Cyt b₆f → PC → PSI → NADP⁺ → NADPH. Proton gradient (ΔpH): ○ H⁺ released in lumen during photolysis. ○ Cytochrome b₆f pumps protons, increasing proton-motive force (PMF) for ATP synthesis. PSI: Accepts electrons from PC & transfers to ferredoxin (Fd) → FNR → NADPH. Emerson Effect & Photosystems I & II Emerson Effect: Photosynthesis is more efficient when plants absorb light at two different wavelengths (PSI = 700 nm, PSII = 680 nm). Z-Scheme: Linear electron flow from H₂O to NADP⁺, involving PSII & PSI.