Biol 341 Ecology Exam 2 PDF
Document Details
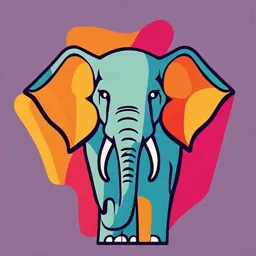
Uploaded by ProvenAlexandrite9303
Tags
Summary
This document appears to be an outline or chapter of an ecology textbook, examining population genetics, and natural selection.
Full Transcript
1 Biol 341 Ecology Exam 2 Chapter 4: Population genetic and natural selection Charles Darwin ○ 1835 Charles Darwin visited Galapagos Islands and become convinces various populations evolved from ancestral forms...
1 Biol 341 Ecology Exam 2 Chapter 4: Population genetic and natural selection Charles Darwin ○ 1835 Charles Darwin visited Galapagos Islands and become convinces various populations evolved from ancestral forms ○ 1838 After reading an essay by Thomas Malthus Theorized some individuals would have a competitive advantage conferred by favorable characteristics The key: Darwin’s Theory of natural selection ○ Natural selection acts on individuals ○ Evolution changes population ○ Organisms produce like organisms Heritable variation among individuals ○ Evolution mergent from this process More offspring produced than can survive → natural selection → non-random survival and reproduction Some traits confer higher survival than others in the same population → natural selection → Altered trait frequencies ○ Individuals do not evolve They are selected ○ Evolution drives changes in population Gregor Mendal ○ Augustinian Monk Studied garden pea Discovered characteristics passed from parent to offspring inform of discrete packets called genes Exist in alternative forms called alleles ○ Dominant and recessive 2 Variation within populations ○ Phenotypic variation results from combined effects of genes and environment ○ Many plant species differ dramatically in form from one elevation to another Distinctive ecotypes →locally adapted Ecotypes: ○ Locally adapted and genetically distinctive populations of a species ○ phenotypic differences (growth/flower production) of clones grown at 3 elevations are the result of environmental differences Phenotypic plasticity ○ Variation as result of environmental influences 3 What might cause variation among populations? ○ Genetic variation in Alpine Fish Movement of cold adapted whitefish into the headwaters of glacial valley in Alps created clusters of geographically isolated populations Isolation drives differentiation Hardy Weinberg: a null model ○ Hardy Weinberg principles help identify evolutionary forces that can change gene frequencies in populations ○ States that in a population mating at random in the absence of evolutionary forces, allele frequencies will remain constant ○ Lady beetles: 4 If SS-81% SA 18% AA 1%. What is the frequency of S? ○ By using p^2 + 2pq + q^2 = 1 Probability equations P(A∩B) = P(A) x P(B) 5 ○ Conditions necessary for Hardy Weinberg: Random mating No mutations Large population size No immigration Equitable fitness between all genotypes Likely at least one of these will not be met and allele frequencies will change Potential for evolutionary change in natural populations is very great Genetic Drift ○ Change in allele frequencies due to chance or random events ○ Reduces genetic diversity over time by increasing some alleles and reducing or eliminating others ○ More problematic in small populations ○ I.e endangered species ○ Founder effects → reduced genetic population ○ Bottlenecks → usually disease 6 Natural Selection ○ Different survival and reproduction among phenotypes ○ Some individuals in a population, because of their phenotypic characteristics, will have higher survival and produce more offspring Fitness of an individual is the relative contribution of offspring, or genes, to future generations Natural selection can favor, disfavor, or conserve the genetic make-up of populations Stabilizing Selection ○ Stabilizing selection acts to impede changes in population by acting against extreme phenotypes and favoring average phenotypes ○ Under stabilizing selection extreme phenotypes have lower rules of reproduction and survival As a consequence, the average phenotype remains the most common from one generation to the next Directional selection ○ Directional selection leads to changes in phenotypes by favoring an extreme phenotype over other phenotypes in the population ○ Under directional selection am exceptional phenotype has higher reproduction and survival As a result, the population average changes in a particular direction over time Disruptive selection ○ Disruptive selection creates bimodal distribution by favoring two or more extreme phenotypes over the average phenotype in population ○ Under disruptive selection average phenotypes have lower reproduction and survival compared to the extremes Over time, average phenotypes become less common and the population becomes phenotypically more diverse 7 Heritability is a key condition of evolution ○ Natural selection which changes genotypic and phenotypic frequencies in populations can result in adaptation to the environment ○ Depends on heritability of traits: h^2 = G/G+E G= Genetic variance E = Environmental variance Vp= Phenotypic variance is a function of environment and genetics Heritability of a trait ○ h^2 (heritability) is 0.6 for egg size in ural owls ○ Example of stabilizing selection 8 Rapid adaptation by Soapberry Bugs ○ Bug feeds on seeds from family Sapindaceae Has slender beaks to pierce fruit walls Beak length should be under selection Close relationship between fruit radius and beak length Comparison of radius of fruits produced by native and exotic species Assume evolutionary change ○ Native → introduced plants → decrease of survival ○ Introduced → native -> decrease of survival ○ Undergo natural selection and example of directional selection ○ Darwin’s Finches ○ One species of finch 2 different beak sizes ○ Few intermediate individuals → disruptive selection Those with medium size beaks had disruptive selection Small / large size beaks thrived Evidence of genetic drift in Chihuahua Spruce ○ Larger population had more genetic diversity 9 Genetic Variation in Island Populations ○ Genetic variation is lower in island populations ○ I.e the non singing crickets and non pickiness females Genetic Diversity and Butterfly Extinctions ○ Inbreeding may drive extinction in small populations Reduced fecundity, juvenile survival, life span ○ Experiment on Glanville fritillary butterflies Higher inbreeding and high extinction rates Evolution and Agriculture ○ “Artificial selection” Breeding of domesticated organisms for desirable traits ○ “Genetic engineering” Introduction and deletion of genes GMOs Unintended Evolutionary Consequences ○ The use of chemicals in agriculture can have evolutionary consequences ○ Plant and animal pests may evolve resistance ○ MRSA antibiotic resistance Antibiotics, herbicides, insecticides Selection events that drive rapid evolution of populations Chapter 9: Population Distribution and abundance Populations ○ All individuals of the same species occupying a specific area at the same time Individuals having a high potential to interact ○ Species generally consist of a group of actually or potentially interbreeding populations Why study distributions? ○ Endangered species, pest populations, commercial/game species ○ Ecological variability ○ Distributions may be seasonal Population ○ Ecologists define this as a group of a single species in a specific area ○ We can describe it by: Number of individuals Density Distribution Abundance 10 Age structure, growth rates, etc Environmental Constraints ○ Physical environment plus biotic factors limits geographic distribution of a species Organisms can only compensate so much for environmental variation Remember previous chapters on temp, water relations ○ Niche: Summarizes biophysical environmental factors that influence growth survival and reproduction of a species Niche ○ Fundamental niche Organisms theoretically could live ○ Realized niche Includes biotic interactions such as competition/ predation/diseases/ etc that may restrict where's a species may live I.e Kangaroo distribution and climate Climate influences species distribution by ○ Food production ○ Water supply ○ Habitat ○ Incidence of parasites, pathogens and competitors I.e Distribution of plants along a moisture temperature gradient ○ Different Encelia species distribution correspond to variations in temperature and precipitation E. farinosa and E frutescens overlap and have similar distribution Partition range among microclimates to which they are adapted E.far grows in shallow soils limited water E.fru grows deep soils Same range but completely different environments Distribution of individuals on small scales ○ 3 categories 1. Random An individual has an equal probability of occurring anywhere in an area Not really interacting Neutral Equal chance of being anywhere 11 Uniform distribution of resource, random dispersal 2. Regular Individuals are uniformly spaced through the environment Overdispersed Big gaps means there are some negative interactions Competing resources Uniformly or systematically spaced Exclusive use of areas with little overlap Individuals avoid one another 3. Clumped Individuals live in areas of high local abundance separated by areas of low abundance Individuals have positive interactions Facilitation of each other or maybe concentrated resources Unequal chance of being anywhere Mutual attraction between individuals Patchy resource distribution Dispersal clumped Distribution of Desert shrubs ○ Traditional theory suggests that desert shrubs are regularly spaced due to competition ○ Phillips and MacMahon found distribution of shrubs changed from clumped to regular shrubs grow ○ Clumped for 3 reasons: 1. Seeds germinate at safe sites “nurse plants” 2. Weak seed dispersal 12 3. Clonal Reproduction ○ Phillips and MacMahon Proposed as plants grow, some clumps die reducing clumping Competition among remaining plants produced higher mortality Eventually creates regular distributions ○ Brisson and Reynolds found competitive interactions with neighboring shrubs appear to influence distribution of creosote roots Organism size and population density ○ Average population density decreases with increased body size, across a wide spectrum of animal groups ○ Plants Plant population density decreases with increasing plant size Tree seedling can live at high densities but as the tree grows density declines progressively until mature trees are at low densities 13 ○ Behavior is distribution of tropical bee colonies Hubbell and Johnson predicted aggressive bee colonies would show regular distributions while non aggressive species would show random or clumped distributions 4 species with regular distribution were highly aggressive 5th was non-aggressive and randomly distributed Distribution of individuals on Large Scales ○ Bird populations across North America Root found at continental scale bird populations showed clumped disruptions in Christmas Bird Counts Clumped patterns occur in species with widespread distributions Brown found a relatively small proportion of study sites yielded most of records for each bird species in breeding bird survey Plant Distribution along moisture gradients ○ Whittaker examined distributions of woody plants along moisture gradients in several North AMerican mountain ranges Documented gradient from moist canyon bottoms up to the dry southwest-facing slopes Tree species showed a highly clumped distribution along moisture gradients with densities decreasing substantially toward the edges of their distribution Clumping on moisture ○ Abundance of 3 tee species on a moisture gradient Organism size and population density ○ In general, population density declines with increasing organism size Damuth found the population density of herbivorous mammals decreased with increased body size Peters and Wassenberg fund aquatic invertebrates tend to have higher population densities than terrestrial invertebrates of similar size Mammals tend to have higher population densities than birds of similar size Commonness and rarity ○ Rabinowitz devised commonness classification based on 3 factors 1. Geographic range of species 2. Habitat tolerance 3. Low population size ○ Populations that are least threatened by extinction have extensive geographic ranges broad habitat tolerances and some large local populations 14 ○ Rarity Rarity I Extensive range Broad habitat tolerance Small local populations I.e peregrine falcon Rarity II Extensive range Large population Narrow habitat tolerance I.e passenger pigeon ○ Grows in undisturbed forests, which we cut down Rarity III Restricted Range Narrow Habitat tolerance Small populations ○ I.e CA condor The most abundant species and those least threatened by extinction have : Extensive geographic ranges Broad tolerances Large populations Tiger Beetle of Cold Climates ○ High lats and elevations Metabolic rates are higher and prefer lower temperatures ○ This supports generalization that the physical environment limits species distribution Distribution of barnacles along an intertidal exposure gradient ○ Organisms living in a intertidal zone have evolved to different degrees of resistance to drying Barnacles Takeaways ○ Environment limits distributions ○ Small-scale patterns may be random, regular, or clumped ○ Large-scale patterns exhibit clumpiness ○ Population density decreases with organism size Chapter 10 Population Dynamics Questions from last class ○ What are some differences between a fundamental vs realized niche? 15 ○ What conditions might cause organisms to be regularly distributed across a landscape? Competition Negative interacting Clumping ○ Generally, population density increase with body size (T/F) F ○ Why might the distribution of organisms within their range exhibit clumped patterns? availability of resources ○ What are three factors which would cause a species to be rare? Broad habitat tolerance Broad geographic distribution Population status Population Dynamics ○ Study of factors influencing the expansion decline and maintenance of populations Dispersal ○ Movement of organisms or their propagules Dispersal can change the distribution of species and increase or decrease local population densities Immigration and emigration populations I.e dandelion ○ Quantifying dispersal Dispersal can be described using measures such as maxim or average distances or as rates The average dispersal rate may conceal some complexity Outliers may drive more rapid population spread than expected ○ Historical dispersal (Paleoecology) Techniques such as pollen and fossil analysis (radiocarbon dated) can help reconstruct past dispersal patterns ○ I.e seed dispersal measurement Seed movement via wind, water, soil movement, or fauna Seed dispersal by small and big animals and humans Meta-populations ○ Group of sub-populations in habitat patches connected by exchange of individuals among populations ○ I.e rocky mountain parnassian butterfly ○ What factors could affect the metapopulation of butterflies in meadows of this forest landscape? 16 ○ Butterflies are more likely to emigrate from small populations Demographic study of populations ○ Study of balance of births and deaths ○ Calculate essential info about populations ○ Life table: Summarizes survivorship and deaths (mortality) “Cohort” Group born during the same time period of interest such as a year ○ Survivorship curve: How many individuals survive past each age? ○ 3 main ways to estimate survival: 1. Track individuals from birth to death 2. Record age of all deaths and backtrack 3. Estimate survival/mortality based on current age structure 17 ○ Survivorship curve Type 1 High survival for young-med age Low survival of old Type 2 Constant survival across ages Type 3 Extremely low survival of juveniles Old have high survival Age distribution ○ Can measure all at once by aging individuals in a population such as using tree rings ○ This population structure suggests population is stable or growing Old can be replaced by many young trees 18 ○ Unstable population No youngins Most trees are leftover from past recruitment Gaps Gaps caused by droughts Peaks caused by pluvials (rainfall) 19 l x m = lm Who lives/dies x reproduction = sum Life tables ○ (age)(prop.survival)(repro)/ net repro rate ○ log(net repro rate) / generation time 20 Takeaways ○ Dispersal rate drive population expansion ○ Concept of a meta-population ○ Survivorship curves Type 1: young dies Type 2: doesn't matter Type 3: old dies ○ Age distributions ○ Life tables Chapter 11 Population Growth Questions for last lecture ○ Why might rates of dispersal be misleading? ○ A stable meta-population implies no sub-population are declining (T/F)F ○ What type of survivorship curve best describes a typical plant? Type 3 ○ What might result in a different survivorship curve? Drought rain ○ What might we infer from an age distribution that is almost entirely very young? Grows in the future More review : an equation for population size ○ Nt= Nt-1 + B + I -D - E Nt = population size Nt-1 = population size at previous time step B = birth rate I = immigration rate D = death rate E = emigration rate Exponential growth often feel like an on/off switch 21 22 Geometric population growth ○ With abundant resources and few limiting factors, populations can grow geometrically (successive generations differ in size by a constant ratio) 23 Exponential growth ○ When generations are not discrete ○ Can model unlimited growth as exponential ○ Change in time (dN/dt) as function of per capita increase and number of individuals Notice dN/dt increases with N ○ Population size (Nt) as function of initial size, per capita increase and time Paleo-ecological example of exponential growth ○ Receding glaciers exposed new habitat ○ No competition unlimited resources Contemporary exponential growth ○ Protection → grew exponentially When exponential growth slows ○ Exponential growth can’t continue forever ○ Populations hit resources, space, competition, predation, disease, or other limits imposed by the environment ○ This logistic growth 24 Logistic Population Growth ○ As resources are depleted, predators are attracted, diseases spread Population growth (r) slows stops s-shaped/sigmoidal (k) carrying capacity/ environmental resistance ○ Max population that environment can support ○ From this example, what is the value of K? About 250 25 Logistic population growth cont. ○ N/K → environmental resistance ○ As N gets larger, resistance to growth increases Tends to reduce population growth Visualizing impact of environmental resitance ○ Growing N reduces per capita growth r ○ N=K growth is 0 ○ 26 ○ I.e Petri dish Artificially set up populations Populations initiated N>K ha populations decline Negative growth rate Why is the rate of logistic population growth at low population densities (N) almost the same as exponential growth? ○ N/K value Low population low environmental resistance Density dependence Fundamental characteristic of many ecological phenomena How does population density influence population growth? Limit on high populations Biotic or abiotic? Biotic and abiotic factors matter ○ Modifies birth/death rates ○ Mods to immigration, emigration, dispersal possible Galapagos finches ○ Wet years and dry years result in boom/bust population cycles Food availability also linked to demographic rate (birth) ○ Wet years reduce death rates (starvation) and increase birth rates ○ Outcome of more food Higher egg laying rates May be individual physiology mechanism 27 Youngmay also be more successful Combination of biotic and abiotic drivers of populations ○ The extreme wet year that was good for some plants was bad for cacti ○ Abiotic effect: too much water, tor, fell over ○ Biotic effect: vines smoother plants ○ Specialist finches eat cacti pollen, nectar, seeds, and insects ○ What are the impacts of these birds on cacti reproduction in 1983? Density dependent flower damage Abiotic damage from water biotic damage from vines reduced caucus density after 183 At lower cactus densities, birds damage more flowers ○ Fewer to et Caused lagged recovery of cactus reproduction for multiple years after 1983 wet event Populations are “clumped” in space ○ Coasts ○ Valleys/drainages of large river systems ○ Estuaries and deltas Singapore 8k/ km^2 Nevada 10/ km^2 Alaska