Adaptation and Traits - Differentiation of Populations PDF
Document Details
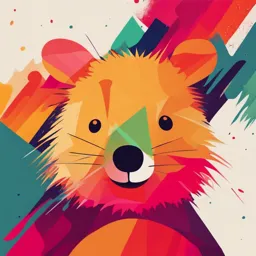
Uploaded by SophisticatedConstructivism1378
2025
Tags
Summary
These lecture notes cover the topic of adaptation and traits, focusing on the differentiation of populations. Key concepts discussed include gene flow, genetic drift, and the impact of environmental factors on population variation. The notes also present examples of genetic and genomic analysis used to understand population dynamics and adaptation.
Full Transcript
Adaptation and Traits 6. Differentiation of Populations, - 03/11/2025 Our last time together, we were learning about quantitative traits and kind of how these states can respond to selection. 4:01 We thought about how, you know, traits that are heritable and influence fitness in the parent, um, 4:...
Adaptation and Traits 6. Differentiation of Populations, - 03/11/2025 Our last time together, we were learning about quantitative traits and kind of how these states can respond to selection. 4:01 We thought about how, you know, traits that are heritable and influence fitness in the parent, um, 4:07 generation and how, um, that can influence the variation in trait values in offspring population. 4:14 But everything that we've kind of looked at before was like, okay, 4:24 there's all these traits that we're interested in all then adaptation or not going to adaptation. 4:28 But at the end of the day, those states, um, do they have an underlying genetic basis? 4:33 Right. But what we don't often think about is that most of the DNA in genomes is non-coding. 4:38 So selection is not necessarily acting on a large proportion of the genome. 4:46 So what can we use that information for when we look at variation in what we say neutral markers. 4:54 So selection is not playing at all. 5:01 This is when we can get a really strong idea of the impact of non um neutral processes, unusual processes like gene flow and genetic drift. 5:03 So whenever we're looking at neutral markers this is where we're going to be like okay how is 5:16 gene flow interrupt actually shaping the variation within and between populations that we see. 5:21 And that's kind of the point um, of today's um class. 5:27 So these are the learning outcomes. 5:32 Essentially we should be able to describe in general terms how does population size and distance between populations, 5:34 like actual physical distance between populations influence patterns of variation? 5:42 Um, when is especially when we're looking at neutral um loci, we're going to revisit ideas about if esteem, 5:47 which measures levels of differentiation among populations and how we can interpret low or high if esteem values. 5:57 You should be able to describe how you can compare neutral markers with the patterns that we see with neutral markers, 6:07 with the patterns that we see with, um, adaptive loci. 6:13 So loci that are under the influence of selection. And then in general terms we want to be able to think about what are the positive 6:18 and negative potential const um consequences of gene flow between populations. 6:25 And when we are done with this section, we'll move on to a very brief introduction to the genetics of adaptation. 6:33 So this first part is going to look at, you know, neutral loci. 6:40 And the second part is going to look at um loci that are actually under selection. 6:44 So let's start with our grounding. Um, example. 6:50 Does anybody recognize this iconic conifer? 6:55 Any ideas? It's a conifer, so it should say something. 7:06 I feel like you want to take a guess. No. 7:13 Oh, I suppose I should have taken that out every time I forget to. 7:20 Like. I'm asking you a question. I shouldn't be the answer anyway. 7:23 So this is a Sitka spruce that's on a tree of life. 7:26 This is where it occurs. And it's a really cool, um, species because it has a very unique distribution, um, along the coast, um, of North America. 7:32 So, um, the distribution is really interesting because it covers this really broad latitudinal range, 7:44 and populations all along its range will therefore experience very different, um, climatic conditions. 7:53 So what are some of these differences between, for example, 8:02 the population at the extreme southern end of its distribution in California versus the population? 8:07 At the extreme northern end of his distribution in Alaska. 8:13 And it turns out that they have very different environments, as expected. 8:18 So at the population in Alaska, winter daylight hours are about only 5.5 hours, whereas in California the average daylight hours is 9.5 hours. 8:22 There's also significant differences in temperature. So average January temperatures range from -7 to -2 in Alaska, but four and 11 in California. 8:34 So intuitively you could think like okay, these are three different environments. 8:49 So selection can play at all. Selection is probably going to influence a whole lot of traits associated with growth in the species. 8:53 But yeah selection probably is. But what about those other evolutionary processes. 9:02 What about drift. What about gene flow. So some things that we should never forget to think about, right. 9:06 We can't always just have this adaptation approach like thinking that everything is an adaptation. 9:14 So you should always keep in mind that the environment is not constant over space or time. 9:21 Right. 9:27 Intuitively, we know this, but we kind of don't think about how these changing environments can influence the patterns of variation that we see. 9:28 Also, most populations are not fully isolated from one another. 9:37 Right. Even though those populations that we saw of the spruce that's in California and Alaska, 9:41 they seem pretty isolated to another because they are so far apart, but they are actually connected. 9:48 Right. They connected between all the populations along um, that distribution. 9:53 So not all populations are completely isolated from others. 9:59 Also keep in mind that loci traits and the strong selection is probably going to show 10:05 you different patterns over space and time when you look at patterns of neutral loci. 10:10 And that is because selection is going to create some kind of variation in some pattern. 10:17 And then those other processes drift and gene flow can give you a different pattern. 10:22 So the big question that we essentially going to think about today is how do populations vary over space in their neutral and selected loci. 10:29 And then also how can we tell the difference between patterns that result from 10:40 drift in gene flow alone and those that are under the influence of selection? 10:46 So the first thing that we're going to do is we're going to review our expectations or how drift can act on neutral assign isolated populations. 10:53 And then we're going to introduce the concepts of gene flow. And we're going to revisit FSD. 11:02 I know that Sally already introduced that a little bit. So we'll just go into um some more detail over there. 11:07 And then we're going to put all of this together and kind of think about how can we tell, um, when drift um, drift is acting on it. 11:16 Um, on a drift is the course of particular pattern versus selection. 11:25 Okay, so let's review what we know about drift. 11:31 So imagine that these three little circles are three different, um, populations at a particular point in time. 11:36 All of them have identical, um, allele frequencies at neutral polymorphic at at a neutral polymorphic locus. 11:44 Right. So this means and there's no exchange of genes between them. 11:53 There's no gene flow. Remember when we talk about a neutral locus we are saying that the there's no real impact of selection. 11:57 So there's no detectable effect on fitness. 12:05 And a polymorphic locus is essentially when there are two distinct alleles, um, in a particular population or sample. 12:08 Okay. So. 12:18 Let's start with a first. 12:25 I click a question. 12:29 If you know what happens over time to the allele frequencies of that particular locus in one population, just imagine this is population one. 12:33 Does that help you predict what you can observe in example population C. 12:43 So you have two options A or b. 12:49 So let me know what you think. 12:54 Okay, so responses are tapering off of it. I'll just give it a couple more seconds. 13:42 Okay. So I'm going to. Close the poll and 54321. 13:53 Okay. Right. So the vast majority of you, um, were correct and chose option B that. 14:09 No, because each population experiences independently. 14:19 Right. So, um, the first one was saying that. 14:24 Yes, because all populations of the same species experienced the same strength of. 14:28 Um, and we know that that is not true. 14:33 Right. So this could have been even like a true or false question. Um, for for a came. 14:36 So if we go to the next slide, um, if you know the outcome of one polymorphic locus, 14:47 does it allow you to predict what you might observe in another locus in the same population? 14:57 So now we're not comparing between populations. We kind of want to know what's happening in one population. 15:03 If you have one locus, um, and, um, another in the same population. 15:08 So this isn't a cyclical question. I want you to chat with each other, um, and then share what you think. 15:15 Essentially, if you know what's happening in one locus, can you predict what's happening in another locus and the same population? 15:23 Okay. Yeah. Let someone be brave enough to share the ideas. 16:22 Don't make me pick someone. Yes, I think you thinking the combination size the. 16:29 Yes. So you've identified a very important factor. 16:44 Right. And that is population size. So, um, population size is going to influence how the strength of drift in a particular population. 16:47 So if you the population size is, I don't know, whatever value, kind of knowing what's happening in one locus can help you to some extent, 16:58 think about what's happening in the other locus because they have the same population size, right? 17:10 Um, and that's really great. So not specifically all the polymorphic loci within a population at the same population size. 17:19 So now instead of, um, you know, kind of just leaving it the way I want to illustrate this by using a really cool example, um, of the wooly mammoth. 17:27 Right. This is like an iconic species. I'm sure we've all we've all heard about it. 17:36 Um, so mammoths were all extinct mammals, and they're actually closely related to Asian elephants. 17:42 And they lived in Asia and North America throughout the Pleistocene. 17:48 So they only, um, went extinct relatively recently. 17:52 Right? The last population that we know of, um, existed on the little island, um, off the coast of, um, Siberia, called at Angle Island. 17:57 And the earliest fossils that we have, um, only dates back to about 4000 years ago. 18:11 So, um, they went extinct very recently. 18:17 What's also really cool is that, um, for many mammals, fossils, 18:24 they were so well preserved that scientists were actually able to extract really good quality DNA, 18:29 allowing them to do some genetic, um, and genomic analysis, um, based on that. 18:36 And it allowed scientists to kind of get a really good understanding of what was happening in those populations as they approached extinction. 18:43 Right. So. 18:53 Oh, I really should have, like, animated this one to just show up as it comes up, 19:03 but this is essentially showing you the results of one, um, particular study that did, um, a genome, um, analysis. 19:09 So what they did, um, in this study was they compared the genomes between two individuals. 19:19 The first individual was one from Wrangel Island. 19:27 Um, and this fossil is only about 4300 years old. 19:30 And they compared that to, um, another individual from a mainland population. 19:35 So this individual, um, died about 44,000 years ago. 19:41 So this is kind of giving you the snapshot about what the populations were experiencing at the time. 19:48 And you are essentially comparing a population that's part of the mainland and probably connected to other populations. 19:55 And then you're comparing that to a very isolated population. 20:04 Um, on Wrangel Island, they probably had a very small, um, population size. 20:08 So how do we interpret this figure? Um, so these are the results of two different chromosomes. 20:15 So it was one in chromosome three. 20:23 And the red lines the height of the red lines measures the divergence between two alleles at that particular position. 20:26 So the higher the line the greater the difference right at those particular positions. 20:35 This, um, gray bars is what's really interesting. 20:42 They represent what we call runs of homozygous city. 20:48 So it's parts of the genome in which there was no, um, differentiation. 20:52 There was no variation. They were only, um, zygotes, um, like homozygous. 20:57 Right. 21:04 So when we look at the, at Angle Island, um, individual at both chromosome one and chromosome three, large parts of the genome wasn't very different. 21:05 And this could be indicative of inbreeding. 21:18 So, um, it indicates interbreeding between closely related individuals. 21:23 So not only, um, did the Dangle Island, um, individual show more evidence, um, of inbreeding, 21:34 but if they also had, um, much longer runs of homozygous city than the mainland population. 21:43 So it suggests both inbreeding and small population size, probably, um, contributed to the extinction of mammoths. 21:55 So the idea is that if you are looking, um, for these kinds of signals in the genome, it can tell you about the influence, um, of drift. 22:07 Right. 22:18 So because there wasn't a lot of variation and because there was a lot of inbreeding, the effect of drift on that small population size in that angle, 22:19 population probably, um, significantly influenced to speed up the extinction of mammals in that population. 22:27 The takeaway message of this is that population size is such a big impact on the strength of drift. 22:39 So the rate and loss of genetic variation and the data and also genetic variation, 22:47 which means that it is going to impact a large portion of the genome or most loci across the genome. 22:52 So there is still this very strong evolutionary force. 23:00 So what are the consequences of these long term effects of drift on isolated populations? 23:10 And the idea is that genetic diversity can be lost over time in isolated populations. 23:17 And this is made even more pronounced when the population sizes are really small. 23:23 And always keep in mind that even though you know, when we're talking about the draft, 23:30 most of the time it's it's results in this loss of genetic variation. 23:34 You know, sometimes they could be, um, mutations that can actually be beneficial. 23:39 But then because selection is not necessarily acting or because population size is so small, the draft can actually take that away. 23:45 So just can both increase and decrease variation. And it can actually um, expose the little recessive alleles, um, as homozygous. 23:52 So don't think about drift as like this other evolutionary force that's having the small impact. 24:03 Right. That's not true. Especially when there are differences in population size. 24:09 So why should we care about studying wooly mammoth extinction? 24:15 And that is because many of the species that we have identified, um, as, um, 24:20 very vulnerable to extinction, so show similar patterns in the, um, genomes. 24:26 Right. So they show some similarity. Similarity, low levels, um, of heterozygosity. 24:31 Um, so yes, the Wrangel Island, um, wooly mammoth, there's the one from the mainland, 24:38 and we can see that many different, um, species have, um, even lower levels of heterozygosity. 24:44 So just to be conscious of time, I can actually play this little video, but you are welcome to do that. 24:54 Um, at home. Um, it gives you a really nice snapshot of this study done with Wrangel Island and some subsequent studies. 25:01 And I actually do want you to watch this video. Right. Um, 25:11 because it will allow you to make connections between extinction and kind of how we 25:14 can learn about extinction with what we're going to learn about later in the course. 25:19 Um, around extinction and macroevolution. So that's something that you can kind of do at your own time. 25:25 But I would suggest that you do that. Okay, so any questions? 25:31 No. Okay. So now we're going to think about another, um, really strong force, um, evolutionary force, which is gene flow. 25:43 And we kind of use gene flow and migration, um, interchangeably. 25:55 Um, so the wooly mammoth was kind of an example that illustrated what happens when you have really isolated and small populations. 26:00 But what about populations that are not isolated from one another, right. 26:08 Like the mainland population of mammoths? 26:13 When populations are not isolated from one another, from one another, the influence of gene flow, um, becomes very important. 26:16 Gene flow can be defined as the movement of individuals. Um, proper duals like seeds for gametes from one population to another. 26:24 But the important distinction is that it results in the incorporation of the genetic material from one population to another. 26:34 So it's not like seasonal migration. 26:40 You know how the wildebeest always migrate from one part of the Serengeti, like, to another part, another not significant, but another part of Africa? 26:42 That's seasonal migration. It's migration together with interbreeding visits. 26:50 Exchange of genetic material. So if two populations differ in the frequency of presence or absence of different alleles, 26:54 if there's gene flow between them, that's actually going to change the allele frequencies. 27:07 If you imagine you have subpopulation one and subpopulation two in each, um, different colored circle represents like a particular allele. 27:13 So let's do another. I think a. 27:25 I'm gonna, uh, start it. 27:31 Um. Sorry. 27:39 I'm gonna restart it. 27:42 Okay. So it's now question three. 27:49 Sorry, I will fix that. And I clicker afterwards. 27:54 So imagine that you have some people I like on sitting, and I don't even know if you've read everything properly. 28:00 Okay. Imagine you have four different populations. 28:05 The sizes of the circles indicates the population size, and the distance between them can be indicative of the geographic distance. 28:08 So each letter is not what you would expect about the pattern of gene flow. 28:17 Okay. Number of responses. 28:43 Uh, slowing down similarly to the numbers from the previous question. 28:46 Okay. 54321. 28:59 Let's close that. Okay. 29:06 So again, the vast majority of you answered, be small populations will have highest numbers of migrants leaving them. 29:11 With someone like to share why they did not choose a nearby populations will share more gene flow. 29:22 Does that make intuitive sense? 29:36 Just because they are new to one another, it might just be easier for um individuals to move and therefore, um, interbreed with number. 29:38 Yes, large populations will generally have the highest number of migrants leaving them. 29:48 And for the last one, nearby populations will be more likely to have similar allele frequencies at the neutral loci than distant populations. 29:56 Simply because of that first one number, a nearby populations will likely share more gene flow. 30:04 What this should tell you. Is that how far they move? 30:12 Could have a significant impact right on gene flow. So dispersal distances. 30:24 This is the idea about how far organisms actually disperse from where they were born or hatched or grew, 30:31 um, to where they would actually, um, reproduce. So most dispersal events actually occur over short distances. 30:40 It's very rare that individuals can travel, um, over very long distances. 30:49 So this is just a graph to illustrate. Um. Dispersal distance in the scrub J. 30:55 So we showing differences between male and female dispersal. 31:03 Um so we have distance on the x axis, um, and the proportion of males and females that are dispersing. 31:08 So if we just look at females, you'll see the um, most females um dispersed out of the shorter distances, 31:15 but they are quite a few that will disperse over much, um, much greater distances compared to males. 31:23 Most males kind of stay very close to, um, their natal data choose like where they were actually raised. 31:31 And even though this distance seems relatively small, right. 31:40 The same kind of pattern you can see across many different, um, species, we, um, most dispersal distances, 31:45 well, most organisms will dispersal short distances and few will disperse of a large distances. 31:54 And this is going to really impact how gene flow, um, impacts populations and changes in allele frequency. 32:00 Who say that example. You have again, these four populations. 32:10 Right. And the space between them is indicative of the geographic distance in large populations. 32:13 There'll be a lot of gene flow. So most individuals will leave large populations. 32:21 And then from the smaller populations for part they could still be some level of gene flow. 32:26 This population is nearer. So again, more individuals are living, um, migrating to um the large to the smaller population. 32:33 But there's still this asymmetry, right. Gene flow from this small population to the large one is, um, still smaller than the other way around, 32:41 but it's a little bit bigger than from this population over there. 32:50 The idea is that there's always going to be asymmetry in gene flow between any population. 32:54 Because of dispersal distances. And that could also just be due to the geographic distance between them. 33:01 So how can we model? Um, migration. So don't worry. 33:11 This is not something that you're actually going to have to know or calculate. 33:17 I've only included it here because I wanted to show you how we can actually, um, model mutation, um, migration over here. 33:22 And if you just compared it very superficially to how a model mutation. 33:31 You can see that the equations are relatively similar, right. 33:36 So like mutation, um, migration can be measured as a fraction of individuals moving from one population to another. 33:40 Um per generation. So even though these equations are quite similar, there are some key differences that you should take into account. 33:48 The first is that, you know, um, with mutation, 33:59 these are things that happen randomly in different parts of the genome when migrants move from 34:03 one population to the next and then actually interbreed with individuals in that population. 34:09 They are actually taking all of that levels. So all of the loci, um, involved. 34:13 And that will change the little frequencies of everything. 34:18 Typically when you think about migration, they are orders of magnitude larger than values for mutation rates. 34:22 So we know that mutation is actually something that's very rare right. 34:30 Competitively. But migration um, is very common. 34:34 And migration happens all the time. So the effect of migration and gene flow is actually a very powerful force. 34:39 And that's going to impact not just how, um, neutral loci or changes in a little frequency neutral loci, but also in loci. 34:48 That's important for adaptation. Is that. 34:57 Is that okay? So this is kind of the next step is just a review, um, of what of what Sally introduced before about FSD. 35:04 And we say that FSD is a measure of differentiation between pairs, um, of populations. 35:20 The reason why FSD, such a um, well used metric is because it can really give us a sound idea of how drift and gene flow can act across populations, 35:26 um, in a landscape, across the landscape. So, um, when you think about FSD, it's always a comparison between two populations, right? 35:38 So we have cell population one and cell population two. 35:51 And again a little circles um, indicate uh different alleles. 35:55 So the subpopulations are actually part of the total population of the species. 36:02 We essentially think about subpopulations as populations all the time. 36:10 But when you're trying to figure out if these T values, um, we can think about subpopulations. 36:14 The total population is all of the cell populations together. 36:20 So say, for example, in your study you've collected data from ten different populations across the species distribution. 36:24 Each one of that will be considered a cell population. And the total population is everything in your sample. 36:32 So the total is all of the individuals pooled together across all populations. 36:39 So now let's think about how can we actually measure if s t. 36:47 So this equation does occur on your formula sheet. 36:52 But we will not ask you to actually use it. Write something that you can refer back to if you need a reminder. 36:56 So that's essentially the equation. And we want to measure the difference between um populations using FSD. 37:03 So the two terms that we're going to think about is the expected um total heterozygosity right. 37:14 So it's based on assuming the whole set of individuals. 37:20 So it's like the total capacity of all the cell populations in your analysis. 37:24 Hers is the expected hitches like a city in each sub population. 37:30 With its unique set of alleles. So if you remember from Sally with, um, she told you that the FSD ranges from 0 to 1 zero, 37:36 meaning that there is no genetic differentiation between populations and one meaning that they are completely different. 37:48 So when FSD is zero between populations, it means that they have identical allele frequencies. 37:58 So in these two sub populations they have the exact same allele frequencies. 38:07 So when you take um the total population um. 38:13 Into account it's also the same allele frequencies. 38:20 Right. So in this case um TT minus the average um of the city between the cell populations is equal to zero. 38:25 And your FSD is then also equal to zero. 38:36 The idea is that if FSD is zero, there's no genetic variation, no differences in allele frequencies between these two populations. 38:39 At the other extreme, when f.a.s.t. is one, it means that between the two populations different alleles are fixed. 38:50 So you have a scenario like this in one sub population. 38:59 Say for example this is a level A1 is fixed and in a population to the um A2 is fixed. 39:04 So they are completely different. And that means that in the end your FSD is equal to one. 39:13 So what does this tell us about drift and migration? 39:23 Um, in these two populations? If f s t um is zero, what does that tell you? 39:27 Mhm. Yeah. 39:38 Yes. Yeah. So I'm not going to ask you to, like, go through all the equations of how to calculate total aces and stuff like that. 39:57 So just think about the fact that if the alleles in the sub populations are exactly the same, 40:04 if you then take everything together, it's still going to be the same. 40:09 I. What do I mean by. 40:14 A little. In that particular population. 40:24 Yeah. So yeah, I think I think if you if you want to go more into it, you can kind of like review expected heterozygosity. 40:33 We're using hardy-weinberg, that kind of thing. 40:42 But the main thing that that you should be able to do now for, for these kinds of interpretations and questions, 40:45 is to think about what patterns do we expect when FST is zero versus what patterns do we expect when FST is one? 40:52 Right. Either no genetic variation so the levels are exactly the same or complete difference between them where different alleles are fixed. 41:01 So the idea is it ranges from zero with no differentiation to one with fixed differences. 41:14 But most of the time we're not going to get either 0 or 1. 41:22 There's going to be some value in between. There's going to be some form of some level of genetic, um, variation. 41:26 So FSD is used to get an overall estimate of population differentiation, right? 41:35 And that means that to get a really good kind of to be confident in your FSD calculations, 41:41 you have to include information from many loci and from many populations because it kind of averages everything together. 41:47 In many of these kinds of studies if history is reported as a pairwise table. 41:57 So it's literally comparing um, population one with population two, um, 42:02 and then population one as population three, and just creating a really long table with all those comparisons. 42:06 And it also includes this includes an average um, if SD. 42:13 Other things to think about with FSD, um, is that we always interested in looking at differences among populations within a species, 42:20 and therefore it can give us some idea of the level of gene flow between populations. 42:29 On average, FSD is going to be lower. 42:38 When you comparing populations of the same species versus populations, um, or versus comparing different species, which is almost never done any more. 42:42 If his team will also be higher in species that have, um, very low dispersal. 42:54 Right. So, um, if you have low dispersal, it's going to decrease gene flow potential gene flow between populations. 43:01 And therefore it's going to increase FSD with a higher genetic, um, variation between them. 43:09 Um, and kind of like I said before, um, FSD is actually hard to compete among unrelated groups of species. 43:17 So FSD is really like a population level analysis within species. 43:25 So if you ever see a paper where they're talking about FSD and they're comparing very different, 43:29 um, species, um, you have to be very careful about how they've interpreted that. 43:34 So let's kind of look, um, at our spruce example or revisit our spruce example. 43:40 So in this paper they use neutral microsatellite markers. 43:47 And in their analysis they found FSC between populations ranged from 0.09 to 0.11. 43:52 So even though there was this very, um, distinct distribution across a um, latitudinal range, the FSD values was relatively low. 44:01 So how can we interpret this? Why do we know it's low. So 0.09. 44:14 It's telling us that about 90% of the total genetic variation that they detected was shared across all populations in the range. 44:19 So they were actually very genetically similar. 44:29 Remember it's 0.09, so it's closer to zero than one of these to one. 44:34 And this result is not really surprising, right? 44:42 And because these teas are very long lived and they are wind pollinated, so that facilitates dispersal and therefore gene flow among populations. 44:45 So again it could be that, you know, the California population, there's no direct gene flow from day to day to Alaska from Alaska today. 44:57 But they could be gene flow all the way along. And in that way the alleles are being shared across, um, 90% of the populations. 45:06 Does that make sense? Right. To interpolate from 0 to 1. 45:19 Close to zero. Three. Genetically different. Um. 45:23 Close to one. Um. No. Sorry. All the way down. 45:26 Close to zero. Not an entirely different. Close to one. Um. 45:30 Very different. So other ways in which you can probably, um, come across how differences between populations, 45:33 um, can be illustrated is this example below, which is a structure plot. 45:43 Have you seen that before? And you probably will see it in an upcoming, um, tutorial as well. 45:49 Um, and essentially what it does is you'll have your data set, you'll have your, um, 45:54 neutral markers for all the individuals and the populations that you are interested in. 46:00 And you would say like, okay, how are these populations, um, grouped, how are they subdivided according to how similar they are genetically? 46:04 And then you could get, um, a plot like this. In this case, there's two different alleles, um, purple and green. 46:15 And each bar is showing you that variation in a particular individual. 46:23 Um, so these kinds of plots are very common when we're looking at genomic data. 46:29 And if we just look at the y axis, this is going to show you the proportion of variation assigned to each of the two groups. 46:37 So I'm just going to take um an individual. Most um of the variation um in this individual is assigned to green and the rest to purple. 46:46 So you don't need to know the details of this particular example. 47:00 You should just know that in this analysis they were interested in looking at, um, these different groups, 47:04 populations in the North Coast and south and looking at the genetic variation between them. 47:09 Okay. So when we thinking about, um, measuring f.a.s.t. between populations, it's going to tell you how different the populations are. 47:16 Um, across one or more loci. 47:31 And because we have this asymmetry of gene flow that we spoke about before, you could think about how gene flow can pull alleles, right. 47:35 Um, together. So if there's a lot of gene flow, it's, um, creating that bridge between populations. 47:46 So the alleles will be shared. And then you have something like drift also acting on the same populations that can pull them apart again. 47:54 Right. So this is how things can become complicated fast is when you interested in a particular group of organisms. 48:04 And you want to see how selection or adaptation, um, influences a trait, 48:12 but you cannot discount the influence of drift and selection, um, drift and gene flow. 48:19 So in any study, 48:25 most of the time the best thing to do is to look at both loci that are under selection and look at how is drift actually acting on this population. 48:27 And to do that, you then also look at data from neutral loci. 48:37 And that can tell you about drift and gene flow. 48:42 Okay, so one thing that we often also don't think about is just this influence of distance, physical distance between populations. 48:51 If we were to measure genetic distance using neutral markers and then also plot that against geographic distance, what do you think we will find? 49:02 Do you think that populations closer together are going to have a, um, greater or lower efficiency value? 49:15 Yeah. So if, for example, we were just looking at if it's between these two populations and these two and these two, 49:28 they have lower, lower genetic distance and lower FSD because they are close together. 49:38 If we compare populations that are further apart, if IST values are going to be a little bit higher. 49:45 And if you see is going to be highest amongst the populations that are furthest apart from one another, 49:53 and this results in a pattern that we call isolation by distance. 49:59 We see something where the greater the geographic distance between them, the greater the genetic distance between populations as well. 50:05 And what I want you to do in your notes is I want you to actually draw a line, 50:14 draw a straight line that would estimate where it would fit, um, across these points. 50:19 Because that's going to drive home the fact that there's this positive correlation. 50:29 So isolation by distance is a very common pattern. 50:38 And we see it all the time. It shows with is increasing genetic distance with geographic distance. 50:42 And isolation by distance is so important. It's, you know, it seems so intuitive. 50:52 It seems really easy to kind of understand. But what you need to remember is that it represents the outcome of two forces gene flow and genetic drift. 50:55 And even though isolation isolation by distance is actually very common, it's not always we don't always find it. 51:11 And the reason why is because sometimes gene flow can just be so high, right? 51:20 Um, so if there's very high gene flow, then, you know, 51:27 maybe the geographic distance between populations doesn't have any it doesn't impede gene flow, essentially. 51:31 Also, um, you might not get an isolation by distance pattern when you have something like a founder event. 51:40 When there's frequent losses of populations. Um, that can kind of obscure this kind of pattern. 51:48 But isolation by distance might not be the pattern that we observe. 51:58 Also, because of the reason that selection could be playing a role. 52:02 And this is why in these kinds of studies, you have to look at, 52:07 it's almost like you need to use different data sets to piece together the entire 52:11 picture of how evolution is acting on a particular system that you're interested in. 52:16 So let's go back to our spruce example. We say that it um the distribution follows this latitudinal gradient. 52:23 Um, and it ranges from very cold temperatures to higher temperatures. 52:32 Right. So it would make sense. 52:38 Like we could think that they could be loci. Um, that's under selection due to this differences environment. 52:42 And that could also change um allele frequencies. 52:50 So we could think that, um, you know, going from cold to warmer temperatures. 52:55 This is the two populations that experienced the greatest, um, climatic difference. 53:03 But not only does this, um, or rather this geographic distance. 53:11 Is also reflected, um, in the difference in temperature. 53:19 So in this graph of air, we have to distance from the center of the range and in mean annual temperature. 53:25 Essentially it's showing that the further populations are apart from one another the lower the mean annual temperature. 53:32 So what this means is that, you know, we could expect and the isolation by distance, this kind of pattern with neutral loci. 53:42 But if we looked at loci that may be important for temperature adaptation. 53:50 We could get a similar pattern. Right. 53:54 We can get a similar pattern from individuals that are adapted to colder environments, to individuals that are adapted to warmer environments. 53:57 So now because of this climate variation in climate, this clonal variation matters. 54:05 The variant, the geographic distance. If we look at loci that should be under adaptation and loci um that's neutral. 54:11 They could be giving us the same pattern. So how can we actually then determine if drift is important. 54:19 I mean, if selection is important and there are two ways in which we can do it. 54:28 The first kind of traditional way, it's an oldie but a goodie, is looking at something called a QST and QST. 54:32 Um is essentially an analog of FSD, and it measures differentiation in quantitative trait values. 54:41 So, I mean, we've spent, um, a whole two lectures looking at quantitative traits, and I'm not going to ask you to calculate QST. 54:52 I would if I were to ask you a question, I'd actually just give you the quiz two values. 55:00 And in the same way that you would interpret a 50, that is how you interpret the question. 55:05 So the quantitative traits that were measured in this study was maybe something like height at age three, but set growth period and growth rate. 55:11 So um, QST was then calculated and then we see this range of values. 55:21 So for high QST was 0.79 for bad set to 0.89. 55:29 So what does that tell you if you are interpreting it in the exact same way as you would interpret FSD, what is x t value of 0.89 tell you? 55:35 More variation. So they are very different in the quantitative states over there in these particular quantitative traits. 55:49 And you can compare it to this one. Growth rate um, is relatively low. 55:57 So that means that the growth rate is not very different across populations. 56:03 So the idea is that you would then look at the traits as well and how those traits vary, um, across populations. 56:07 Uh, more common way of doing things today is using a method called genome wide association studies. 56:17 And this is where, um, you essentially, um, get the genome of your organization of interest, 56:25 and then you're going to compare traits and see if, um, differences in the traits map onto positions in the genome. 56:34 That kind of has been flagged as important for variation. 56:42 So it's a um, quantitative trait loci mapping. 56:48 Well, this genome wide association kind of study is linking two types of information. 56:52 It's linking the phenotype. Um so normally things that we can measure with the genotype. 56:59 So in this case the authors were looking at the trait of height. 57:06 Um, the bands over the uh indicate loci or um regions of interest in the genome. 57:13 Um, so it's just showing the position on a particular chromosome. 57:21 And here three different loci, um, has just been identified. 57:25 And once this has been identified and then we can compare, okay, what's happening with a trait. 57:30 And we can see that for um, position one and position three there's actually no variation in the state. 57:35 Whereas at position two. Um, individuals that have these mutations at position two. 57:43 Um, they have a much they are much taller. 57:52 Right. So we can associate the trait with a position, um, on the genome. 57:56 And the blue curve over here is simply a measure of likelihood. 58:02 The likelihood that that trait that has been identified is actually associated with the phenotype. 58:07 Came. So what I want you guys to do now is just spend a few minutes, um, discussing these two points. 58:17 Right. I want you to think about under what scenarios. 58:29 When will gene flow kind of be a good thing, and when will it be a bad thing? 58:32 In other words, can gene flow actually help or hinder adaptation, um, in different populations. 58:38 So spend a couple of minutes and then I'll get back to you. Student. 58:45 So I guess for a little bit of that. Yeah. Okay, so it's kind of like a very open ended question. 59:59 So, you know, I like to share the ideas for how gene flow can influence adaptation. 1:00:14 Yes. Mhm. 1:00:21 That. But it's also a bad thing, especially like let's say, at. 1:00:37 Mhm. That's right. Yeah, I like that. 1:00:44 Yeah. So it could be a good or bad thing because, um, you know, if a population is struggling. 1:00:50 So I'm assuming, like, maybe something that's close to extinction or whatever. 1:00:56 Um, introducing, um, new alleles that could be beneficial, um, could actually rescue a population. 1:01:00 Um, but it could also be a bad thing if history between populations are very low. 1:01:08 Um, it means that they're very similar. 1:01:13 But then, you know, there could be a big selection event like a new disease that's introduced because they have one similar, 1:01:14 um, is that a lot of variation for natural selection to be acting on? 1:01:21 Um, and then it could actually cause extinction. Any other ideas? 1:01:26 Like that didn't really cover, like everything. It was good. What is this? 1:01:34 More. Anyone like to share. 1:01:37 Okay, so I'm going to leave that up to you guys. Um, I have included some, some ideas, like in the next couple of slides. 1:01:45 Um, and I think that that's okay for you to review, because I want you to also start making, like, 1:01:55 those connections and thinking about how gene flow can actually influence all these different aspects, 1:02:03 um, that we've been introduced to, but essentially they are some positive effects and definitely some negative effects as well. 1:02:08 Um, and I'll just go through one example of here, like when populations that are well suited to the local environments, 1:02:16 they can be negatively impacted by gene flow from populations experiencing different conditions. 1:02:23 So say for example, you had, you know, populations like the spruce that is in warmer climates. 1:02:29 And suddenly there's gene flow from populations that are better adapted to, 1:02:37 um, you know, colder environments, then that's not really going to help them. 1:02:41 Right. By gene flow coding and interbreeding happening, it could kind of mess up a good thing, um, in that particular population. 1:02:45 Okay. Another thing that I want you to think about. 1:02:55 Um, and of course, you know, if you want to come to office hours or we can even do it, 1:03:01 like as a discussion board on Piazza or something, I think I'll just create a post that people can and share the ideas. 1:03:07 I want you to also think about the idea that, okay, most of the time, um, 1:03:15 we know gene flow is asymmetrical and it's usually from larger populations towards smaller populations. 1:03:20 And this is kind of exactly what they found in this, um, study with the spruce. 1:03:26 So, um, populations in the northern, northern and southern edges, um, of the species range receive more gene flow from the center. 1:03:31 So there's more gene, gene flow towards the edge of the species range and towards the center. 1:03:45 And yeah, I want you to think about what are the potential consequences of that and think about what does it mean about a species range. 1:03:52 Right. What's happening at the extremes of the range? 1:04:01 What kind of environments are they? Do they represent the limit to where the species can actually, um, um, survive and persist? 1:04:05 So think about that asymmetry of gene flow. Um, and then yeah, I'll open a discussion thing on um, Piazza and then we can share ideas about that. 1:04:17 Okay. Is there any questions? It's. 1:04:30 Could that movement be a response to movement of what? Off gene flow. 1:04:38 Yeah. Definitely. So climate change is just kind of missing everything up, right? 1:04:44 So usually at the end, um, species have a limit to the kinds of environments that they can sustain. 1:04:50 They can adapt. Right. And like we saw with, uh, um, the earth, with climate change, it meant that they've shifted their ranges, 1:04:59 they've shifted where they can actually assist, but they might also be a limit to that. 1:05:08 So yeah, that's one of the things that you can maybe bring up in the in the Piazza post and get some get some discussion going. 1:05:13 Okay. So in the next 15 minutes I'm going to try to get through. 1:05:21 I'm pretty sure I can. Um, thinking about the genetics of adaptation. 1:05:28 So we've looked at new loci and kind of how we can tease apart the impact of drift in gene flow and what patterns we expect. 1:05:33 And then we also said like, okay, you know, sometimes the patterns that we, um, 1:05:41 see in neutral loci is the exact same as patterns that we can see in um, um, loci and selection. 1:05:46 So we have to like, perform extra analysis or do more kinds of things to then determine if the pattern 1:05:55 that we see in selected loci is due to selection versus only due to drift, 1:06:02 um, and gene flow. But in this section we kind of want to think about, you know, where does what is selection actually act on. 1:06:07 Right. Is adaptation um, mostly from what we call standing genetic variation. 1:06:17 Or do adaptations mostly happen because of novel mutations? 1:06:24 And then you should also have a basic understanding of some analyzes used to determine association between traits genes. 1:06:30 And I think for that one I think we we covered we covered most of that um, in the previous class actually. 1:06:36 Um, and let's in the interest of time, let's just scratch out number three. 1:06:43 Okay. So I won't I won't get to those last three, three slides. 1:06:49 So you can just cut that one out. Let's focus on the first and second one. 1:06:53 So the big questions that we're going to think about is where do new adaptations come from? 1:06:59 So do adaptations arise from areas that are already present in populations, or do they always only arise from new mutations? 1:07:05 Um. And can we can you make predictions about that? So just a quick review. 1:07:15 Do you remember that when you went to think about whether adaptation is involved? 1:07:21 These are three things that need to happen. There should be, um, an association between the taste values and fitness values. 1:07:25 They should be a genetic basis for the trade. 1:07:36 Like we should know that they are some genes involved. 1:07:39 And we also need to understand the mechanism of selection. 1:07:44 We need to show that there's something happening in the environment that's going to influence, um, evolution on natural selection, on those traits. 1:07:47 So where do adaptive states come from? They can either come from new mutations. 1:07:59 So they arise in species or populations. 1:08:05 Um, but allows them to adapt. Or adaptive alleles can come from existing variation. 1:08:09 So alleles are already present in the species, but they could be neutral or even deleterious. 1:08:17 Right. Um, the idea is that when something changes in the environment, 1:08:25 when there's a new selective pressure or selective context, the levels are already there. 1:08:31 And now they can be favored because now selection can actually act on them. 1:08:36 So this is called standing genetic variation. Variation that's already there that selection can act on when the selective environment changes. 1:08:41 So always remember that for complex states, new phenotypes can also emerge when different um leaders at different loci can come together 1:08:55 in new combinations that we are going to focus on today is just looking at mutations. 1:09:04 And in the second part, um, adaptation by standing genetic variation. 1:09:09 So a lot of the examples that we've actually looked at, um, so far involved selection acting on variation that's already been in the population. 1:09:15 Right. But you might then wonder, like, how often do novel mutations actually prove to be beneficial? 1:09:25 Um, so I'm going to describe a really cool, um, experiment that was done using Drosophila. 1:09:33 And yeah, the researchers were trying to determine, okay, if you were to expose, um, these organisms to, um, a particular environment. 1:09:42 Um, what would would they essentially survive? 1:09:54 So in this experiment, they used as close to genetic clones as they could get. 1:09:59 So they had inbred homozygous lions. So essentially clones. 1:10:06 And the flies in the experiment were reared on food with different salt levels. 1:10:12 So some flies were reared on food with very high salt levels and others, um, with very low salt levels or. 1:10:18 No. So what they then did was, um, different populations. 1:10:25 They then exposed those populations to environments with very high salt concentration, and all flies died. 1:10:31 So they did this because they wanted to kind of get an idea of how, at what point is salt concentration lethal? 1:10:39 Um, for these different lines. So with this first kind of celebrity, um. 1:10:48 Um, experiment, they could then conclude that populations in these cloned clyne's lack alleles for tolerance to high salt. 1:10:57 So now that they know that there's this, um, basis of, um, 1:11:09 this degree in which all concentration is lethal, no matter how, um, how these different lines operate. 1:11:14 They wanted to then ask, can salt tolerance evolve? 1:11:22 So they did this with the experiment. They had a control and they had a treatment and the control. 1:11:27 The flies were raised without salt in their food, and they decided to do this for 30 generations. 1:11:34 After the two generations, the flies were introduced to, um, a food source with a very high salt concentration, and all of them died. 1:11:41 So they were still unable to survive on high salt food. 1:11:52 So it meant that no mutations for high salt color tolerance was actually present after 13 generations. 1:11:58 And then for their treatment. They also had flies that were raised in a patchy environment. 1:12:11 Right. So in this patchy environment, flies had access to both low concentration salt food and high salt food. 1:12:17 But they were also tricky in that they didn't provide enough food for salts. 1:12:27 Um, for the flies, um, in the little vials, which meant that there was some competition between flies. 1:12:32 Right. And they also did this for 30 generations. 1:12:39 After this experiment, they found that when they introduced the flies that were raised in a patchy 1:12:48 environment to only other food sources with a very high salt concentration, 1:12:57 many of them actually survived. So there was a mutation, um, for the ability to consume high salt concentration food. 1:13:03 So for the control lines, the flies were raised without salting the food, 1:13:14 and then by the time they were exposed to the new environment, it was no novel mutation. 1:13:19 But that doesn't mean that mutations didn't happen within those 30 generations, right? 1:13:27 Mutations are random, but because there was no need, 1:13:32 there was no selection for high salt tolerance because they were eating food with no salt all along. 1:13:36 Even if there was a beneficial mutation for salt tolerance, 1:13:43 it didn't matter because there was no fitness advantage for them at that time without selection. 1:13:46 That mutation could have just been lost. So the rates of mutation between the control lines and the treatment lines would have been very similar. 1:13:54 Right. Because mutations are random. 1:14:06 So it's a random change in DNA. So there shouldn't have been differences between the two treatments. 1:14:11 But what the results does tell you is that the thickness of novel high salt, um, tolerant mutation was different in the two types of lines. 1:14:18 Right. Flies that had mutations for high salt tolerance in the patchy environment would have had an advantage, 1:14:30 because then they could consume food with both high salt concentration and low salt concentration. 1:14:38 And that means that when they were exposed to an environment that only had high salt concentration, they could survive. 1:14:45 They already had those mutations. 1:14:53 The takeaway from this is that we know from a pub chain module that neutral mutations are likely to be lost by drift, 1:14:57 and even beneficial mutations can be lost by drift, but they'll have a better chance of spreading if they provide some kind of fitness benefit, right? 1:15:08 So in this experiment, it shows that context matters. 1:15:22 The high salt concentration environment was so, um, harsh that it required a mutation that would, 1:15:26 um, that would infer high salt tolerance very, very quickly. 1:15:37 But that didn't happen, right? The individuals couldn't escape the conditions because mutations, they are random. 1:15:44 They don't appear when you need them. Right. So mutations could have happened, but it was likely lost in the control lines. 1:15:53 Does that make sense? Right. 1:16:03 So mutations can be beneficial, but it's not necessarily always the when you need it. 1:16:06 And that is why adaptations most of the time occurs from standing genetic variation. 1:16:12 Variation that already exists um, in the population. 1:16:17 So it looks like I won't actually be able to finish, um, the evolution from standing genetic variation. 1:16:24 So I think that even though we've got a few minutes, this is where I'm going to stop. 1:16:31 Um, and if you want, we can actually have that discussion. Or do you want to go? 1:16:35 We can actually then have that discussion about gene flow. Um, in the day and age, if you're like, no. 1:16:41 I think people are backing up. Okay. So I'll put it on PSA. 1:16:49 Um, and we can just have a discussion based on that.