Genetics Study Guide PDF
Document Details
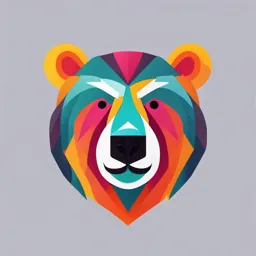
Uploaded by HaleImpressionism8557
Xavier University of Louisiana
Tags
Related
- Human Genetics and Molecular Biology Notes PDF
- Molecular Biology and Genetics - Explorations: An Open Invitation to Biological Anthropology (2nd Edition) PDF
- Lesson 2: Central Dogma of Molecular Biology: Replication PDF
- Molecular Biology Final Exam 2021-2022 KING SALMAN Univ PDF
- BIOL10221 Molecular Biology DNA Replication I 2024 PDF
- Molecular Biology Summary PDF - DNA, RNA, Genetics
Summary
This document provides a comprehensive study guide for genetics, covering key concepts such as DNA structure, gene expression, and various types of mutations. It also explores the fundamental processes of transcription and translation, including the roles of RNA and proteins. Practice questions are included to help students prepare for exams.
Full Transcript
Overview of Genetics Genetics, the scientific study of heredity and variation, forms the cornerstone of understanding how traits and characteristics are transmitted from one generation to another. At the heart of genetics is the concept of the gene, traditionally defined as the 'unit' of heredity wh...
Overview of Genetics Genetics, the scientific study of heredity and variation, forms the cornerstone of understanding how traits and characteristics are transmitted from one generation to another. At the heart of genetics is the concept of the gene, traditionally defined as the 'unit' of heredity which encodes specific traits through its functional product, usually a polypeptide. Fields of Genetics Genetics is broadly categorized into three primary fields: Transmission Genetics Molecular Genetics Population Genetics Transmission Genetics Transmission genetics, the oldest field, concentrates on how traits are inherited from parents to offspring. Gregor Mendel's pioneering work in the 1860s laid the foundation for this field, introducing key principles such as the law of segregation and the law of independent assortment. These principles elucidate how genes, passed down as discrete units (now known as genes), follow specific inheritance patterns, which can be empirically tracked and analyzed through genetic crosses over multiple generations. Key Concepts in Transmission Genetics: Law of Segregation: Each individual has two alleles for each gene, which segregate during gamete formation such that each gamete carries only one allele for each gene. Law of Independent Assortment: Genes for different traits are inherited independently of each other. Molecular Genetics Molecular genetics delves into the biochemical mechanisms underlying genetic material, primarily focusing on DNA's structure, function, and regulation. This field encompasses detailed analyses of DNA, RNA, and proteins, elucidating how genes are organized, expressed, and regulated at the molecular level. Fundamental Processes: Gene Expression: The two-step process where DNA is first transcribed into RNA, which is then translated into proteins. These protein products are crucial for cellular function and, ultimately, the phenotypic traits observed in organisms. DNA Structure: DNA is composed of nucleotide sequences, where each nucleotide contains one of four nitrogenous bases: adenine (A), thymine (T), cytosine (C), and guanine (G). The specific sequence of these bases encodes the genetic information necessary for protein synthesis. Population Genetics Population genetics addresses the genetic composition of populations and how it varies over time and space. It bridges Mendelian genetics and Darwinian evolution, focusing on gene pools and the impact of evolutionary forces such as mutation, selection, genetic drift, and gene flow. Key Focus Areas: Genetic Variation: Differences in inherited traits among individuals, stemming from mutations, changes in chromosome structure, or variations in chromosome number. Role in Evolution: Genetic variation provides the raw material for evolutionary change, allowing populations to adapt to changing environments. Genetic Variation and Interaction of Genes Genetic diversity is vital for adaptation and survival. Variations in genes and alleles lead to different traits among individuals, influenced by: Gene Mutations: Small changes in gene sequences create different alleles. Chromosomal Alterations: Large segments of chromosomes may be lost or duplicated, impacting gene function and expression. Chromosome Number Variations: Loss or gain of entire chromosomes can result in significant phenotypic changes. Genes and Environment Interplay Traits are not solely determined by genes but also influenced by environmental factors. For instance, phenylketonuria (PKU) is a condition where individuals cannot metabolize phenylalanine due to defective genes. However, by controlling dietary intake, the detrimental effects of this genetic condition can be mitigated, showcasing the interplay between genes and environment. This comprehensive exploration of genetics, from the transmission of traits and molecular underpinnings to the broad-scale population dynamics, offers a detailed understanding suited for in-depth study and analysis. Identification of DNA as Genetic Material Genetic material must: Information: It must contain essential information to make an entire organism. Transmission: It must be transmissible from parent to offspring. Replication: It must be able to replicate so it can be passed on during cell division. Variation: It must be capable of variation to account for the diversity within and between species. The identification of DNA as the genetic material was a result of several critical experiments. Griffith's Transformation Experiment Frederick Griffith studied Streptococcus pneumoniae, which comes in two strains: S (Smooth): Secretes a protective polysaccharide capsule, producing smooth colonies. R (Rough): Lacks a capsule and produces rough colonies. Griffith demonstrated that heat-killed S cells could transform live R cells into virulent S cells in mice, suggesting a "transforming principle" that could transfer virulence. Avery, MacLeod, and McCarty's Experiment Avery, MacLeod, and McCarty identified DNA as the transforming principle by demonstrating that only DNA extracts from S cells could transform R cells into S cells. Treatment with DNase (which degrades DNA) eliminated transformation, while RNase and protease did not. Hershey-Chase Experiment Hershey and Chase used bacteriophage T2 to show that DNA is the genetic material. They labeled phage DNA with phosphorus-32 (32P) and protein with sulfur-35 (35S). After infection of Escherichia coli, they found that the 32P-labeled DNA, not the 35S-labeled protein, entered the bacterial cells, confirming that DNA carries genetic information. RNA as Genetic Material in Some Viruses A. Gierer and G. Schramm isolated RNA from tobacco mosaic virus (TMV) and demonstrated that purified RNA could infect plants, confirming RNA as the genetic material in RNA viruses. Structure of DNA General Structural Features DNA consists of two strands twisted into a double helix. Each strand is composed of nucleotides, which contain: A phosphate group A deoxyribose sugar A nitrogenous base (adenine, thymine, cytosine, or guanine) The double helix structure is stabilized by: Hydrogen bonding between complementary bases (A-T and G-C). Base stacking interactions among the planar surfaces of bases. Chargaff's Rules Erwin Chargaff formulated several critical biochemical observations: Adenine (A) equals Thymine (T) Guanine (G) equals Cytosine (C)These rules provided key evidence for the base-pairing mechanism in the DNA structure. The Double Helix Model by Watson and Crick Watson and Crick, utilizing Rosalind Franklin’s X-ray diffraction data and Chargaff's rules, proposed the double helical structure of DNA. They noted: Antiparallel orientation: The two strands run in opposite directions (5’ to 3’ and 3’ to 5’). Right-handed helix: The helix spirals clockwise away from the observer. Alternative DNA Structures DNA can adopt various secondary structures: B-DNA: The common physiological form. A-DNA: A right-handed helix that forms under low humidity. Z-DNA: A left-handed helix that may be involved in transcription and recombination. RNA Structure RNA is typically single-stranded but can form short double-stranded regions through complementary base pairing. RNA differs from DNA in that it: Uses uracil (U) instead of thymine (T). Contains ribose sugar instead of deoxyribose. Practice questions: 1. What does "base stacking" refer to? →Base stacking is the non-covalent interactions between adjacent nitrogenous bases in DNA. These interactions stabilize the DNA structure by minimizing exposure to water and maximizing van der Waals forces, contributing to the overall stability of the double helix. 2. Explain the significance of the transformation principle discovered by Griffith. → The transformation principle discovered by Griffith showed that genetic material can be transferred between bacteria. This demonstrated that DNA carries genetic information 3. How do the antiparallel strands of DNA contribute to its overall structure? → Antiparallel strands of DNA run in opposite directions, allowing for complementary base pairing. This arrangement stabilizes the double helix structure, enabling proper replication and transcription, and ensures accurate genetic information transfer. 4. Why is it important that DNA has directionality (5' to 3')? → DNA directionality (5' to 3') is crucial because: It determines how DNA is replicated and transcribed Enzymes like DNA polymerase can only add nucleotides in this direction It ensures proper base pairing and genetic information flow during processes like replication and protein synthesis. 5. What are the structural features of the DNA double helix? →Two strands twisted into a helix Sugar-phosphate backbone Nitrogenous bases (A, T, C, G) paired in the center Antiparallel strands (one runs 5' to 3', the other 3' to 5') Complementary base pairing (A with T, C with G) Major and minor grooves along the helix 6. What does the term "transformation" refer to in Griffith's experiments? → The uptake of genetic material leading to a change in phenotype 7. How does the presence of hydrophobic interactions influence the stability of the DNA double helix? → Hydrophobic interactions stabilize the DNA double helix by causing the hydrophobic bases to stack together in the interior, away from water. This stacking reduces exposure to the aqueous environment, enhancing stability and maintaining the helical structure. 8. What is the primary difference between A-DNA and Z-DNA? → A-DNA is a right-handed helix, while Z-DNA is a left-handed helix 9. What is the significance of the major and minor grooves in the DNA double helix? → They serve as binding sites for proteins that regulate gene expression 10.What is the role of DNase in the transformation of bacteria? → To degrade DNA DNA Replication Complementarity of DNA strands: Adheres to the AT/GC rule (Chargaff’s rule). ○ Parental Strands: Original DNA strands. ○ Daughter Strands: Newly synthesized DNA strands from the parental templates. Three models proposed for DNA replication: ○ Conservative Model: Parental strands remain together post-replication. ○ Semiconservative Model: Each daughter DNA contains one parental and one daughter strand. ○ Dispersive Model: Parental and daughter DNA interspersed in both strands post-replication. Bacterial DNA Replication Meselson and Stahl Experiment Objective: To validate the correct model of DNA replication. ○ Used E. coli cultures in mediums with different nitrogen isotopes (15N and 14N). ○ DNA analyzed via centrifugation showed results aligning with the semiconservative model. Initiation Origin of Replication (oriC): ○ Contains AT-rich regions, DnaA boxes, and GATC methylation sites. DnaA Proteins: ○ Bind to DnaA boxes, initiating unwinding. DNA Helicase: ○ Unwinds DNA, generating supercoiling, alleviated by DNA gyrase (topoisomerase II). Elongation DNA Primase: ○ Synthesizes short RNA primers. DNA Polymerase III: ○ Attaches nucleotides to the 3’ end of the RNA primer. ○ Higher processivity due to the Beta (β) subunit (clamp protein), enabling the polymerase to synthesize DNA rapidly. Leading and Lagging Strand Synthesis: ○ Leading Strand: Continuous synthesis towards the replication fork. ○ Lagging Strand: Synthesized in Okazaki fragments away from the replication fork. RNA primers are replaced by DNA by DNA Polymerase I. DNA ligase connects DNA fragments. Termination Ter Sequences: ○ Terminate replication, facilitated by Tus proteins. Topoisomerases: ○ Resolve intertwined DNA (catenanes) by cutting and rejoining strands. Proofreading and Fidelity Mechanisms Ensuring High Fidelity: ○ Instability of Mismatched Pairs: Inherent instability reduces errors. ○ Active Site Configuration: DNA polymerase’s ability to correctly match nucleotides prevents errors. ○ Proofreading Activity: DNA polymerase 3’ to 5’ exonuclease activity corrects mismatches. Eukaryotic DNA Replication Complexity and Multiple Origins Large Linear Chromosomes: ○ Require multiple origins of replication for efficient synthesis. ○ Origins of replication resemble bacterial systems, particularly in yeast (e.g., ARS elements). Initiation and Assembly Pre Replication Complex (preRC): ○ Assembled at G1 phase, involves ORC (Origin Recognition Complex) and MCM helicase, which activate upon phosphorylation. Elongation DNA Polymerases α, δ, ε: ○ Involved in DNA synthesis. ○ Polymerase Switch: Transition from DNA Pol α synthesizing an RNA-DNA hybrid primer to DNA Pol δ/ε taking over for elongation. PCNA: ○ Acts as a clamp, similar to the bacterial β subunit, increasing processivity. Replication Machine (Replisome): ○ Includes helicase proteins complexed with polymerases to facilitate coordinated copying of DNA strands. Telomeres and Termination Telomeres: ○ Protect ends of linear chromosomes, prevent degradation and fusion. ○ Problematic at replication's 3’ ends, solved by the enzyme telomerase adding repetitive sequences. Telomerase Activity and Cancer: ○ Telomeres shorten with age, leading to cellular senescence; cancer cells often exhibit increased telomerase activity preventing telomere shortening. Practice Questions Effects of mutations on DNA replication a. Loss of 3’→5’ exonuclease activity of DNA polymerase III: This activity is responsible for proofreading during replication. A mutation that eliminates it would lead to an increased error rate in DNA replication, resulting in more mutations. b. Loss of 5’→3’ exonuclease activity of DNA polymerase I: This function removes RNA primers from Okazaki fragments. Without it, the RNA primers would remain in the lagging strand, preventing proper DNA synthesis and ligation. Effect of deleting the RNA component of telomerase The RNA component of telomerase serves as a template for adding telomeric repeats. Without it, telomerase would be unable to extend the ends of chromosomes, leading to progressive telomere shortening with each cell division. Okazaki fragments and primer removal A. The right Okazaki fragment was made first because DNA synthesis occurs in the 5'→3' direction, meaning newer fragments are synthesized closer to the replication fork. B. The right RNA primer will be removed first since primer removal proceeds in the same 5'→3' direction as DNA synthesis. The middle Okazaki fragment does not need to be synthesized beforehand because polymerase I can remove the primer and fill in the gap as long as there is an adjacent DNA strand. C. DNA ligase is needed at the left arrow to join the left Okazaki fragment with the newly synthesized DNA that replaced the middle primer. The right arrow does not require ligase at this stage because polymerase I fills in the gap left by primer removal, but a nick remains between the filled-in DNA and the adjacent fragment, requiring ligase to seal it. Translation Introduction: Gene transcription is the process through which genetic information from DNA is copied to RNA by the enzyme RNA polymerase. This sequence is foundational, as RNA then plays various roles, including being translated into proteins. This overview covers various steps, mechanisms, and structures involved in gene transcription, focusing on both bacterial and eukaryotic systems. Stages of Transcription Transcription occurs in three main stages: 1. Initiation: RNA polymerase binds to a DNA sequence called the promoter, which signals the start of transcription. 2. Elongation: RNA polymerase moves along the DNA strand, synthesizing an RNA molecule. 3. Termination: RNA polymerase reaches a terminator sequence, causing synthesis to stop, releasing the newly formed RNA molecule. Initiation: - The promoter region contains critical sequence elements, such as the Pribnow box in bacteria, necessary for the binding of RNA polymerase and other transcription factors. Elongation: - The RNA polymerase synthesizes the RNA strand in a 5’ to 3’ direction, reading the template DNA strand in a 3’ to 5’ direction. Termination: - In bacteria, there are two mechanisms: rho-dependent and rho-independent termination, utilizing specific signals within the RNA to release the RNA polymerase and the newly synthesized RNA strand. Promoter Structure and Function in Bacteria and Eukaryotes Bacteria: - Promoters contain conserved sequences, such as -10 (TATAAT) and -35 elements, recognized by sigma factors within RNA polymerase holoenzymes for transcription initiation. Eukaryotes: - Eukaryotic promoters are more complex and variable but commonly have a core promoter containing a TATA box and a transcriptional start site. They require multiple transcription factors and a mediator complex to initiate transcription efficiently. RNA Polymerases in Eukaryotes Eukaryotic cells have three main RNA polymerases: 1. RNA Polymerase I: Transcribes ribosomal RNA (rRNA) genes. 2. RNA Polymerase II: Transcribes all protein-coding genes (mRNAs) and some snRNAs. 3. RNA Polymerase III: Transcribes transfer RNA (tRNA) genes and the 5S rRNA gene. RNA Modification: RNA modifications are crucial for the stability, export, and translation of RNA. Pre-mRNA undergoes several modifications before becoming a mature mRNA: 1. 5’ Capping: - Most mature mRNAs have a 7-methyl guanosine cap attached at the 5’ end during RNA polymerase II transcription. This cap plays roles in RNA stability, export to the cytoplasm, and initiation of translation. 2. Splicing: - RNA splicing removes non-coding sequences (introns) from pre-mRNA, connecting coding sequences (exons) together. This process can occur through spliceosomes or self-splicing mechanisms, which involve the RNA itself functioning as a ribozyme. 3. 3’ Polyadenylation: - Addition of a polyA tail at the 3’ end after transcription. This tail enhances the stability and translational efficiency of the mRNA. 4. Alternative Splicing: Alternative splicing is a mechanism allowing a single gene to produce multiple proteins. Different exons can be spliced together in various combinations, leading to the production of proteins with different functions from the same gene precursor. Chromatin Remodeling and Transcription Regulation Chromatin Structure: - DNA is packaged into chromatin, affecting accessibility for transcription. The structure can be altered through covalent histone modifications (e.g., acetylation, methylation) and ATP-dependent chromatin remodeling complexes, which modify nucleosome positioning. Regulatory Elements: - Enhancers: DNA sequences that increase transcription when bound by activator proteins. - Silencer: DNA sequences that decrease transcription when bound by repressor proteins. These elements interact with the core promoter and transcription machinery to regulate gene expression effectively. Complementarity Rule: RNA synthesis follows base-pairing rules where adenine (A) pairs with uracil (U) and cytosine (C) pairs with guanine (G). DNA Strands: Template strand (3' to 5'): 3'–CCGTACGTAATGCCGTAGTGTGATCCCTAG–5' Coding strand (5' to 3'): 5'–GGCATGCATTACGGCATCACACTAGGGATC–3' Promoter Location: The promoter is located on the 3' side of the template strand. Effect of Start Codon Mutation to Stop Codon: Transcription will still occur, but translation will terminate prematurely, producing a nonfunctional or truncated protein. Consensus Sequence Meaning & Example: A consensus sequence is a conserved nucleotide pattern found in DNA or RNA, often playing a regulatory role. Example: The -10 (TATAAT) and -35 (TTGACA) sequences in bacterial promoters. Consensus Sequences in Bacterial Promoters: -10 (Pribnow box, TATAAT): Facilitates DNA unwinding. -35 (TTGACA): Recognized by RNA polymerase for promoter binding. ρ-Dependent vs. ρ-Independent Termination: ρ-Dependent: Requires the Rho (ρ) protein to terminate transcription. ρ-Independent: Involves a GC-rich hairpin followed by a U-rich sequence causing RNA polymerase to dissociate. Mutations Affecting Transcriptional Termination: A. Prevent ρ-Independent Termination: Mutations in the GC-rich hairpin or U-rich region. B. Prevent ρ-Dependent Termination: Mutations in the rut site (Rho utilization site). C. Effect on Transcription: Without termination, transcription could continue beyond the intended stop site, potentially into downstream genes or noncoding regions. Core Promoter Elements in Eukaryotic Structural Genes: TATA Box (-25 to -30 bp): Binds TATA-binding protein (TBP) for transcription initiation. BRE (TFIIB Recognition Element, -35 bp): Enhances polymerase binding. Inr (Initiator, +1 bp): Defines the transcription start site. DPE (Downstream Promoter Element, +28 to +32 bp): Works with Inr for promoter recognition Practice Question: 1. Transcription initiation process: - Rna Polymerase holoenzyme binds to dna and scans for the promoter region - Sigma factor recognizes -35, -10 sequences - Tight binding of rna polymerase to dna prepares for transcription 2. Rna polymerase synthesizes rna transcript during elongation - Rna polymerase moves along the dna strand and reads 3’ to 5’ - Uses non coding strand as template - Dna t is replaced with rna u 3. What happens during termination - Rna synthesis ends - rna/dna separates - Rna and rna polymerase is released - Rho-dependent and rho independent 4. Different rna molecules and their role - mRNA: carries genetic information to ribosomes - rRNA: forms ribosomes - tRNA: transfer amino acids to ribosomes - snRNA: involved in splicing pre-mrna to produce rna 5. Consensus sequences enhance transcription efficiency in bacteria by - Provide specific binding site for rna polymerase - Increase recognition by transcription proteins - Higher transcription rates when matching consensus sequences 6. Regulatory elements impact RNA polymerase binding to the promoter by: - Enhancers: Attract activators, stimulating transcription - Silencers: Attract repressors, inhibiting transcription 7. The open complex is crucial for transcription because it: - Unwinds DNA at the promoter - Allows RNA polymerase II to access the template strand - Initiates RNA synthesis - Marks the transition from initiation to elongation phase 8. transcription factors and their DNA-binding domains. - Transcription factors are regulatory proteins that bind to specific DNA sequences, known as enhancer or silencer sequences. Their DNA-binding domains allow them to influence gene expression by either promoting (activators) or inhibiting (r) the binding of RNA polymerase to the promoter. 9. Mechanism by which cell regulate gene expression at the transcription level: - Cis-acting elements: DNA sequences like TATA box, enhancers (stimulate transcription), and silencers (inhibit transcription) - Trans-acting elements: Regulatory proteins (transcription factors) that bind to these sequences to influence RNA polymerase activity. 10. Impact of mutations in the promoter region have on transcription initiation: Mutations in the promoter region hinder RNA polymerase binding, leading to decreased transcription initiation and overall gene expression. 11. Transcription factors influence the rate of transcription - Transcription factors can either enhance or inhibit transcription by binding to regulatory sequences, thus affecting the recruitment and activity of RNA polymerase at the promoter. 12. How enhancer and silencer influence the transcription rate of the gene - The enhancer increases transcription by facilitating RNA polymerase binding, while the silencer decreases transcription by hindering RNA polymerase access to the promoter. mRNA Translation and Protein Synthesis The Genetic Basis for Protein Synthesis Function of Genetic Material: The primary role of genetic material is to encode the production of proteins necessary for cellular structure and function. Structural Genes: Genes encoding polypeptides are known as structural genes and are transcribed into messenger RNA (mRNA) Archibald Garrod: Proposed a relationship between genes and protein production, particularly studying alkaptonuria and establishing the concept of "inborn errors of metabolism". Beadle and Tatum's Experiments: Concluded that a single gene controlled the production of a single enzyme, leading to the one gene-one enzyme hypothesis, which later evolved to the one gene-one polypeptide concept. Translation of mRNA Components Ribosomes: The site of protein synthesis consisting of large and small subunits made up of proteins and rRNA. tRNA (Transfer RNA): Acts as an adaptor molecule matching amino acids with mRNA codons. It has a specific structure that includes an anticodon region and an acceptor stem for amino acid attachment Codons: Triplet sets of nucleotide bases in mRNA that correspond to specific amino acids or stop signals in protein synthesis. AUG is the start codon, while UAA, UAG, and UGA are stop codons. Degeneracy and Wobble Hypothesis: - The genetic code is degenerate, meaning multiple codons can encode the same amino acid. - Wobble hypothesis explains that codon-anticodon interactions can tolerate certain mismatches at the third base position. Stages of Translation Initiation In Bacteria: The formation of the initiation complex involves the small ribosomal subunit, initiation factors, and initiator tRNA (tRNAfmet) recognizing the start codon (AUG, GUG, or UUG). The Shine-Dalgarno sequence facilitates mRNA binding to the ribosome. In Eukaryotes: Involves a more complex assembly with additional initiation factors (eIFs). The 40S subunit, tRNAmet, and eIFs scan the mRNA for the start codon, forming the 80S initiation complex. Elongation Ribosomes move along the mRNA, decoding sequences and adding corresponding amino acids to the growing polypeptide chain. tRNAs charged with amino acids enter the ribosome at the A site, transfer the amino acid to the polypeptide chain at the P site, and exit from the E site. Termination Occurs when the ribosome reaches a stop codon. Release factors (RFs) recognize the stop codons, leading to the release of the newly synthesized polypeptide and ribosomal subunits. Bacteria have three RFs, while eukaryotes have two. Ribosome Structure Functional Sites: Ribosomes have three key sites - Peptidyl (P) site, Aminoacyl (A) site, and Exit (E) site. Differences Between Prokaryotic and Eukaryotic Ribosomes: Prokaryotic ribosomes (70S) are simpler compared to eukaryotic ribosomes (80S), which are found in the cytoplasm and organelles like mitochondria and chloroplasts. Function and Structure of Proteins Levels of Protein Structure 1. Primary Structure: The sequence of amino acids in a polypeptide chain. 2. Secondary Structure: Regular, repeating shapes such as α-helices and β-sheets stabilized by hydrogen bonds. 3. Tertiary Structure: The three-dimensional folding of a polypeptide driven by hydrophobic and ionic interactions, hydrogen bonds, and van der Waals forces. 4. Quaternary Structure: Complex association of multiple polypeptide subunits forming a functional protein. Function Enzymatic Activity: Enzymes accelerate biochemical reactions and are essential for cellular metabolism. Structural and Functional Diversity: Proteins serve a multitude of cellular functions, including structural support, transport, signaling, and immune responses. Practice Questions: 1. What is the role of tRNA during translation? → To recognize codons and carry specific amino acids 2. What hypothesis did Beadle and Tatum propose regarding gene function? → One gene controls the synthesis of a single enzyme 3. How does the directionality of polypeptide synthesis relate to the orientation of mRNA? →Polypeptide synthesis occurs in the N-terminal to C-terminal direction, paralleling the 5’ to 3’ orientation of mRNA. Each amino acid is added to the growing chain by forming peptide bonds, reflecting the mRNA's codon sequence during translation. 4. Describe the process of translation from mRNA to polypeptide. → Codons in mRNA are read by ribosomes tRNA brings corresponding amino acids Amino acids are linked by peptide bonds The chain grows from the N-terminal (5’) to the C-terminal (3’) end This continues until a stop codon is reached, completing the protein. 5. What are the three sites present in a ribosome during translation? → Peptidyl site (P site), Aminoacyl site (A site), and Exit site (E site) 6. What is the significance of the P, A, and E sites in ribosomal function? → P site: Holds the growing polypeptide chain A site: Binds incoming aminoacyl-tRNA for addition to the chain E site: Releases empty tRNA after amino acid transfer 7. What does the wobble hypothesis explain about codon-anticodon pairing? →The wobble hypothesis explains that a single anticodon can pair with multiple synonymous codons. 8. What are the characteristics that define the primary structure of a protein? → The specific sequence of amino acids Peptide bonds linking amino acids Determines the protein's overall shape and function This sequence dictates how the protein will fold into secondary, tertiary, and quaternary structures. 9. How do secondary structures like alpha helices and beta sheets form within proteins? → Secondary structures like alpha helices and beta sheets form through hydrogen bonds between the backbone atoms of the polypeptide chain. The specific amino acid sequence determines which regions fold into these structures, stabilizing them and contributing to the protein's overall shape. 10.How do aminoacyl-tRNA synthetases contribute to the accuracy of protein synthesis? → Charging tRNAs with the correct amino acids Using recognition sites on tRNA for selection Maintaining an error rate of less than 1 in 10,000 Dr.Biliran Questions: 1. An mRNA has the following sequence: 5ʹ‐GCGAUGGGCAAUAAACCGGGCCAGUAAGC–3ʹ. Determine the complete amino acid sequence that would be translated from this mRNA → Met - Gly - Asn - Lys - Pro - Gly - Gln - Stop 2. Describe the sequence in bacterial mRNA that promotes recognition by the 30S subunit → The Shine-Dalgarno sequence is a purine-rich sequence in bacterial mRNA that promotes recognition by the 30S ribosomal subunit. It is typically located 4-9 nucleotides upstream of the start codon (AUG) and has the consensus sequence: 5'-AGGAGG-3'. This sequence base-pairs with a complementary region in the 16S rRNA of the 30S subunit, facilitating ribosome binding and translation initiation. 3. How does a eukaryotic ribosome select its start codon? → The 40S ribosomal subunit binds the 5' cap and scans until it finds an AUG start codon in a favorable Kozak sequence. 4. In which of the ribosomal sites, the A site, P site, and/or E site, could the following be found? A. A tRNA without an amino acid attached B. A tRNA with a polypeptide attached C. A tRNA with a single amino acid attached → A. E site B. P site C. A site 5. Explain what is meant by the coupling of transcription and translation in bacteria. Does coupling occur in bacterial and/or eukaryotic cells? Explain. → In bacteria, transcription and translation are coupled, meaning translation begins while mRNA is still being transcribed. This occurs because bacteria lack a nucleus, allowing ribosomes to bind the mRNA as it emerges from RNA polymerase. In eukaryotic cells, coupling does not occur because transcription happens in the nucleus, while translation occurs in the cytoplasm, requiring mRNA processing and transport before translation can begin. 6. An mRNA encodes a polypeptide that is 312 amino acids in length. The 53rd codon in this polypeptide is a tryptophan codon. A mutation in the gene that encodes this polypeptide changes this tryptophan codon into a stop codon. How many amino acids would be in the resulting polypeptide: 52, 53, 259, or 260? → 52 because the mutation creates a premature stop codon at the 53rd position, halting translation, so only the first 52 amino acids are synthesized. 7. Which steps during the translation of bacterial mRNA involve an interaction between complementary strands of RNA? → Initiation – The Shine-Dalgarno sequence in mRNA base-pairs with the 16S rRNA of the 30S ribosomal subunit. Elongation – The tRNA anticodon base-pairs with the mRNA codon in the A site. Termination – Release factors recognize the stop codon, but no tRNA base-pairing occurs. 8. Lactose permease, a protein of E. coli, is composed of a single polypeptide that is 417 amino acids in length. By convention, the amino acids within a polypeptide are numbered from the amino‐terminus to the carboxyl‐ terminus. Are the following questions about lactose permease true or false? A. Because the 64th amino acid is glycine and the 68th amino acid is aspartic acid, the codon for glycine, 64, is closer to the 3' end of the mRNA than the codon for aspartic acid, 68. → False – Codons in mRNA are read 5' to 3', meaning the 64th codon (glycine) appears before the 68th codon (aspartic acid) in the mRNA sequence. B. The mRNA that encodes lactose permease must be greater than 1241 nucleotides in length. → True – Each amino acid is encoded by a codon of 3 nucleotides, so a polypeptide of 417 amino acids requires at least 1251 nucleotides (417 × 3). Additionally, the mRNA includes untranslated regions (UTRs), making it even longer. 9. What is a polysome or polyribosome? → A polysome (polyribosome) is a single mRNA strand with multiple ribosomes attached, simultaneously translating the mRNA into protein. 10. What is the role of aminoacyl‐tRNA synthetase? → Aminoacyl-tRNA synthetase attaches the correct amino acid to its corresponding tRNA, ensuring accurate translation. 11. A tRNA has an anticodon sequence 3'‐CGG‐5'. What amino acid does it carry? → The anticodon 3'-CGG-5' pairs with the mRNA codon 5'-GCC-3', which codes for alanine (Ala). 12. If a tRNA molecule carries a glutamic acid, what are the two possible anticodon sequences that it could contain? Be specific about the 5' and 3' ends. → 3'-CUU-5' pairs with 5'-GAA-3' 3'-CUC-5' pairs with 5'-GAG-3' Gene Mutations: Introduction: Gene mutations refer to heritable changes in the genetic material, and the cause of most genetic disorders. Some mutations can be detrimental and some can be beneficial which drive evolutionary progress Types of Mutations: Chromosome Mutations: Changes in chromosome structure. Genome Mutations: Variations in chromosome number. Single-Gene Mutations: Small-scale changes within a specific gene. Point Mutations Point mutations involve changes in a single base pair. Base Substitutions: Where one base is swapped for another. ○ Transitions: A pyrimidine replaces another pyrimidine or a purine replaces another purine. ○ Transversions: A pyrimidine is swapped for a purine, or vice versa. Insertions and Deletions Mutations can also result from the insertion or deletion of nucleotide sequences: Insertions: Addition of extra base pairs. Deletions: Removal of base pairs. These alterations can lead to - Frameshift Mutations, changing the entire downstream amino acid sequence. Effects on Protein Polypeptides Gene mutations within the coding sequence can affect the protein structure. Silent Mutations: Base substitutions without altering amino acids due to genetic redundancy. - Genetic Redundancy: multiple codons specifying the same amino acids. Missense Mutations: Change in amino acids, potentially altering protein function. ○ Neutral Mutations: Changes where substituted amino acids have similar properties. ○ Example: Sickle-cell anemia, caused by a single nucleotide substitution, leading to the replacement of hydrophilic glutamate with hydrophobic valine. Nonsense Mutations: Substitutions creating premature stop codons, halting protein synthesis. Frameshift Mutations: Insertions/deletions not in multiples of three, disrupting the reading frame. Classifying Mutations Mutations are characterized by their phenotypic effects. Deleterious Mutations: Reduce survival rates, potentially lethal. Beneficial Mutations: Enhance survival or reproduction. Conditional Mutations: Affect phenotype under specific conditions. Variants emerge with altered phenotypes and differential survival rates. Suppressor Mutations provide a secondary mutation that counteracts the first. Intragenic Suppressors: Second mutation within the same gene. Intergenic Suppressors: Second mutation in a different gene. Gene Expression Alterations Mutations can also impact gene expression: Promoter Mutations: Change the transcription rate. ○ Up Promoter Mutations: Increase transcription by making the sequence more like the consensus. ○ Down Promoter Mutations: Decrease transcription by diverging from the consensus. Splice Junction Mutations: Affect mRNA splicing in eukaryotes. Mutation Origins Mutations originate spontaneously or are induced by environmental mutagens. Spontaneous Mutations: Arise from cellular biological process errors. ○ Example: DNA replication errors. Induced Mutations: Result from exposure to environmental agents or mutagens (chemical or physical). Testing for Mutagenicity The Ames Test Developed by Bruce Ames, this test evaluates chemical mutagen potency using Salmonella typhimurium strains: The test strain cannot synthesize histidine due to a point mutation. Reversion mutations restore the ability, indicating mutagen presence. Process: 1. Control Plate: Indicates spontaneous mutation rate without mutagen exposure. 2. Test Plate: Assesses mutation rate with potential mutagen exposure.