BIOL 240 - Topic 8 Review PDF
Document Details
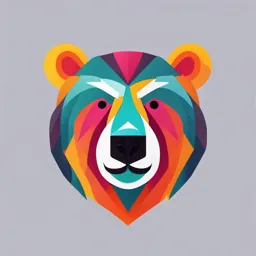
Uploaded by EventfulSnowflakeObsidian23
University of Waterloo
Tags
Summary
This document provides review material on differential gene expression in bacteria. It covers topics such as the role of DNA, transcription in bacteria (including rho-dependent and rho-independent termination), regulation of transcription (including the operon model and Lac operon), and post-translational regulation of proteins.
Full Transcript
Topic 8.1 Differential Gene Expression -------------------------------------- ### Refresher #### The Role of DNA - **Replication**: DNA must be retained intact, yet copied to make new cells (**copy DNA from new cells)** - **Transcription**: DNA must be turned into multiple "working copie...
Topic 8.1 Differential Gene Expression -------------------------------------- ### Refresher #### The Role of DNA - **Replication**: DNA must be retained intact, yet copied to make new cells (**copy DNA from new cells)** - **Transcription**: DNA must be turned into multiple "working copies" to provide instructions for enzymes/structural proteins production **(Making RNA copies of DNA for instructions)** - **Translation**: RNA must be read and decoded to form the enzymes/structural proteins of the cell **(Reading RNA to produce proteins)** - **DNA repair**: systems must have the ability to deal with damage **(Fixing damage to DNA)** #### Transcription in Bacteria 1 - Sigma factors bound to DNA pol core enzyme direct it to a promoter - Transcription proceeds - Different sigma factors can direct core RNA pol enzyme to different genes as needed So: - **Sigma factor role:** A **helper protein** (sigma factor) guides the RNA polymerase to the starting point on the DNA (called promoter) - **Transcription starts: RNA polymerase** opens the DNA and starts copying it into RNA - **Gene selection:** different sigma factors help RNA polymerase choose which genes to copy depending on what the cell needs #### Transcription in Bacteria 2 - **Rho dependent:** rho protein follows RNA pol and removes it from the DNA when it **reaches a termination sequence** (**protein called Rho chases DNA polymerase and stops it when it reaches the end of the gene)** - **Rho independent**: **RNA hairpin loop forms,** causing RNA pol to dissociate from the DNA (**RNA makes a loop shape, which pushes RNA polymerase off the DNA, ending transcription)** #### Transcription in Bacteria 3 - Small ribosome subunit (**30S in bacteria, attaches to mRNA to help start making proteins**) and the **Shine-Dalgarno sequence** help align all the machinery to the correct starting location (**a special sequence on mRNA that matches the small ribosome unit (16s rRNA), acts like a landing pad, guiding the ribosome to the starting codon), ensure protein synthesis starts at the right place).** #### Transcription in Bacteria 4 - Multiple **Shine‒Dalgarno** sequences allow bacterial mRNA to be **polycistronic;** bacterial mRNA can have **multiple starting points (Shine-Dalgarno sequences),** so it can make **several proteins** from **one mRNA strand.** - **Multiple \"Shiny Stops\" on a train track**, where each stop is a new protein-making station. ### Regulation - Different environmental conditions: - Changes in nutrients and availability - Changes in competition - Permits condition specific responses - Substrate-specificity - Metabolism and transport - Sporulation - **Bacterial as flexible workers: They switch tools (specific responses) depending on the job (environment).** - Key cellular enzymes are **constitutive genes (they need to run at all times, house keeping genes)** - TCA Cycle - ATP synthases - Making all enzymes all the time **impractical** - Excess protein production costly - Cellular activities regulated at multiple levels - Cells do not require all gene products at all times - **Constitute genes** always need to be "on" - **Inducible genes** only required at particular times (**only translated when needed**) - Basic control of gene expression can take place on the level of **transcription, translation,** or **post-translation (i.e. activity of enzymes)** **Ideally -- start from transcription to regulate as it doesn't involve making amino acids etc.** ### Proteins #### Regulating protein activity (**Post Translation**) -- Covalent Modifications - **Covalent modification** may alter enzyme conformations - **Phosphorylation** - **Acetylation** - **Methylation** - **Glycosylation** (sugar) Modification can increase/decrease activity; folding different #### Regulating protein activity (Post Translation) -- **Allosteric Modification** (other site, changes activity) - **HAS ANOTHER SITE:** allosteric site: activator or inhibitor (**binding of a non-substrate molecule a site away from the active site)** - **Alters conformation, substrate no-longer binds** - **Allosteric inhibitor** end product of a multi-step pathway - Many **enzymes** use **allosteric regulation mechanisms** - **Allosteric inhibitor:** there is enough product, stop producing it ### Transcription #### Regulation transcription - **Energy** is **conserved** by **controlling synthesis of enzymes** - Many control mechanisms work to prevent transcription when they are not required #### The Operon - Transcriptional unit with a series of **structural gens** and their transcriptional regulatory elements - i.e. the lac operon - all the transcriptional regulations come together around the operon #### General Overview of the Regulators in Transcription **Positive Control**: **allosteric** **protein** acts to **activate mRNA synthesis** **Negative** **Control**: **allosteric protein** acts to **prevent mRNA synthesis.** **E-coli** has **genes** under **one/both/neither.** Some operons can have **more than one promoter,** each with their own control system #### **Negative** Control of Transcription (**operator**) - may involve specific **repression (put it on to inhibit activity)** or **induction (removing mechanism to allow RNA polymerase to proceed)** in response to conditions - **both are considered negative control and involve a repressor protein** - **Mechanism: RNA polymerase binds to promotor, negative control regulatory protein bond to operator to block RNA polymerase enzyme from working** #### Repression vs. Induction - **Repression:** inhibit transcription in response to a signal - **minority** of enzymes are controlled by repression - typically affects **anabolic (biosynthetic, builds complex molecules from simpler ones)** enzymes when there is enough mRNA, want to **repress, don't need to make more** - **Induction:** de-repression of enzyme production in response to a signal - typically affects **catabolic** enzymes **majority, turned off by default** - i.e. lac operon - when a **carbon** source shows up to the repressor protein, **induces the protein to get removed** and it can start RNA polymerase activity - enzymes synthesized only when substrates available - no wasted energy #### **Positive** Control of Transcription (at the **activator binding site**, w/ activator protein) - Allosteric regulator proteins activate binding of RNA polymerase to DNA - **activator proteins bind specifically to activator binding site of the promoter** - i.e. **maltose** catabolism in **E.coli** - maltose activator protein only binds DNA in the presence of maltose - **Using positive control, strengthen RNA polymerase binding with the promoter** - Modifying structure of DNA - Interact directly with polymerase - **Activator site** may be **close to the promoter or up to hundreds of base pairs away** #### Effectors (Effector Molecules) - Collective term for molecules that affect protein production in association with allosteric protein regulators - **Co-inducer or co-activator:** substance that turns on enzyme production - **Co-repressor:** substance that binds and activates as pressor - **Effectors interact with DNA-binding proteins.** #### Lac Operon - Glucose easier to use than lactose - Lac operon is not expressed until **all glucose** is consumed - **Diauxic** growth (2 phases of growth) - Specific mechanism: - LacY (permease): bring lactose into the cell - LacZ (Beta-Galactosidase): cut lactose in half into smaller sugars (glucose and galactose) for energy - Multiple control elements on both DNA and accessory proteins - Inducible expression - System is only turned on when needed (cheaper/easier to use glucose) - Components allow use of lactose sugar - **Sequence of events:** - Permease guide lactose into the cell - B-Galactosidase cuts lactose in half into smaller sugars - Mainly glucose and galactose - A little bit of allolactose as well #### Negative Control in Lac - **Repressor protein (LacI (the gene produces the protein to inhibit default))** binds to operator, **blocking RNA polymerase** and inhibiting transcription - **effector molecule (allolactose, an co-inducer) *induces*** transcription by inhibiting binding of the repressor to the operator  - LacI as the **default \"lock\"**, and allolactose as the **\"key\"** that unlocks the process when lactose is available. #### Positive Control in Lac - **Activator protein (Cyclic AMP receptor protein; CRP)** binds and increases transcription rates when **effector molecule (cAMP) present (low glucose)** - **When glucose level is high, since it is the preferred energy, it doesn't need alternative energy sources like lactose** - **Low glucose triggers cAMP production:** Activated CRP binds to the promoter region, helping RNA polymerase bind and increasing transcription of the lac operon. - **Effector molecule induces conformational change in activator protein, which increases affinity for binding site**, **increasing** RNA polymerase affinity for the Lac operon promoter. #### **Control of the Lac Operon** - **Low glucose, low lactose (no transcription):** - **Low glucose: cAMP** levels are high as need to activate using alternative method, CRP activated and binds to DNA, encourage transcription - **Low lactose:** Not enough lactose to produce allolactose, in which the repressor is still bound to the operator, blocking RNA polymerase - **High glucose, low lactose (no transcription):** - **High glucose: cAMP** levels are low, CRP inactive and cannot help RNA polymerase bind effectively - **Low lactose:** Not enough lactose to produce allolactose, in which the repressor is still bound to the operator, blocking RNA polymerase - **High glucose, high lactose (no transcription):** - **High glucose:** cAMP is low (because glucose is high), so **CRP** is **inactive** and cannot assist RNA polymerase. **Without CRP to help RNA polymerase bind** strongly to the promoter, **transcription is very weak**. - **High lactose:** Lactose is present, so allolactose binds to the repressor (LacI), removing it from the operator. #### Negative Control (one more) Anabolic process - Effector molecules can also inhibit transcription by binding to the repressor protein and enhancing its ability to bind to the operator (common for anabolic operons) - **Effector molecule like tryptophan binds to repressor protein, make it stronger.** - Example here is **tryptophan** **amino** **acid** (**co-repressor**) synthesis operon Topic 8.2 Transcription Translation ----------------------------------- ### Attenuation #### Attenuation (Backup Plan, most complex concept) - **Attenuation: like quenching, interruption of transcription after initiation** but before termination - control of transcription by **mRNA secondary structure** - an interaction between translation and transcription processes - if ribosome quickly follows RNA polymerase, rho-independent terminator hairpin RNA loops are formed in the leader sequence and the polymerase detaches - "Stalling out" of ribosome in mRNA leader sequence (i.e. not enough of that amino acid loaded in tRNA) allows transcription to continue #### **Detailed Mechanism** - **Leader**: located between operator and first structural gene - **Region 2 and 3** forms **anti-attenuator stem loop, allowing transcription to continue** - **Region 3 and 4** form **attenuator stem loop, causing transcription to stop (termination loop)** - **Translation of Leader Peptide:** - The leader peptide contains two tryptophan codons. The ribosome\'s behavior depends on tryptophan availability: - **High tryptophan:** - **What happens:** - Ribosome translates the leader peptide without stalling and **blocks Regions 1 and 2**. This **allows Regions 3 and 4 to pair, forming the attenuator loop**, which **stops transcription**. - **Why it happens:** The cell already has enough tryptophan to function, no need to make more enzymes to produce it - **Low tryptophan**: - Ribosome stalls at the tryptophan codons (due to lack of charged tRNA). This **frees Region 2 to pair with Region 3**, forming the **anti-attenuator loop, allowing transcription to continue**. **Why does this process not able to occur in eukaryotes?** Attenuation by hairpin loops does not occur in eukaryotes because of key differences in transcription and translation processes: 1. **Separation of Transcription and Translation**: - In eukaryotes, transcription happens in the **nucleus**, and translation occurs in the **cytoplasm**. These processes are physically separated. - Attenuation relies on transcription and translation happening **simultaneously** (as in bacteria), so the ribosome's position affects RNA folding. This can\'t happen in eukaryotes. 2. **RNA Processing in Eukaryotes**: - Eukaryotic RNA undergoes modifications (e.g., splicing, capping, polyadenylation) before translation. These steps would disrupt or prevent the formation of attenuation loops. **Order of the regulatory genes: ABS, promoter, operator, leader** ### Quorum Sensing - **Quorum**: **members of a group that must be present** in order to conduct business - **Main functions**: communicate, count, gene expression changes based on how many cells are present - A **chemical signaling system** that allows microbes to **communicate with each other** - Regulation of **gene** **expression** based on **population** **density** - Cells release **autoinducer** molecules into the environment as the **population** **density** **increases** - Detecting changes in autoinducer levels causes regulation of gene expression -- when signal builds up, microbes detect signals and change their gene activity (they are smart!) #### **Characteristics of Quorum Sensing** - Regulation of gene expression based on population density - Positive feedback -- more microbes produce signaling molecules, stronger signal gets, amplifying the response - Rapid induction -- once signal reaches threshold, response triggered quickly, allowing microbes to act simultaneously - Links behaviour to population density - Coordinates expensive, additive processes - Roles in interactions with eukaryotes #### Bioluminescence of Allivibrio fischeri - Lux -- prototypical quorum-sensing system found in Allivibrio fischeri - A. fischeri live freely or in symbiosis with the Hawaiian bobtail squid - cells only emit light (via the enzyme luciferase) when in the light organ of the squid - **need enough population density to trigger the glowing process: quorum sensing system called lux, help them detect when population is high enough** - **need regulation of production of light, when needed** - **Mechanism**: - At high density, bacteria make a signal molecule called **AHL** - **The LuxI protein** produces AHL - **AHL level high,** interacts with **regulator protein LuxR,** which **activates the lux genes** - **Produces luciferase** and **more AHL, positive feedback loop** - **How do they keep growing?** - **luxR,** a **regulator** **transcriptional** **activator (POSITIVE)**, interacts with AHL when it reaches a **high enough concentration** - binds the **lux box DNA regulatory site** (**activator binding site)** - **leads to transcription of luciferase protein genes and luxI, creates + feedback loop forming more AHL** #### **Low Quorum vs. Quorum** - **low level transcription from operon**: low concentration of AHLs in the cell. AHL and LuxR do not interaction. LuxR does not bind *lux* box for activation. Light is not produced - **low population density, low concentration of AHL** - **high level transcription from operon:** high concentration of AHL is in cell. AHL and LuxR interact. LuxR binds *lux* box for activation. Light is produced. - **high population density, high concentration of AHL** - **ATP and O2 required for light (energy expensive!)** #### Quorum Sensing is Widespread - Broad range of microbes possess quorum sensing systems - Mechanism controlled include: - Motility - Conjugation - Biofilm formation - Pathogenesis - Autoinducers may even play a role in competition - Interrupting or inhibiting a control pathway in other MOs in the environment (gain an advantage by stopping competitors from coordinating activities like forming biofilms or producing toxins) Topic 8.3 Two Component Regulatory Systems and Chemotaxis --------------------------------------------------------- ### Two Component Regulatory Systems - can use one protein as a sensor and another to control transcription - allows for **response to changes in environment** - **signal transduction** induced inside the cell alters it to respond appropriately #### Two Component Regulatory Systems Mechanism - **Sensor kinase (i.e. HPK, histidine phosphorylated kinase):** in cell envelop, detects the environmental stimulus - **Response regulator (RR):** regulates transcription 1. External signaling molecule binds the input domain of the component 1, the sensor 2. The transmitter domain becomes phosphorylated using ATP 3. The sensor transfers the phosphate group to component 2, the response regulator 4. The phosphorylated response regulator interacts with DNA of the target gene and RNA polymerase to increase or decrease transcription In short: 1. **Signal detection:** signal (external molecule) attaches to **sensor 1** (like an antenna) 2. **Phosphorylation:** sensor uses **energy from ATP to add a phosphate group to itself (change shape)** 3. **Phosphate transfer**: sensor passes **phosphate to regulator 2** (like a switch, **change shape**) 4. **Gene regulation:** phosphorylated regulator binds to DNA and works with RNA polymerase to **turn a gene off or on (increase or decrease transcription)** - It is like a **relay system**: the signal activates the sensor, the sensor passes the message (phosphate), and the regulator changes gene activity. - **Can't be permanent, short-lived environmental signal** #### Two Component Regulatory Systems Mechanism - Functions: oxygen detection, osmolarity, phosphate detection - Tree tumours (**crown gall tumours**) - requires plant be damages, so pathogen can go in, and then make modification to the cells #### **Virulence of A.tumefaciens** - *vir* genes found on the Ti plasmid are only expressed under conditions similar to a plant wound site - *virA/virG* are required for expression of the other virulence genes 1. sugar and phenolic compounds in the prescence of low pH gets senced by **VirA, (Component 1 sensor: ATP -\> ADP, phosphate group as by-product, attach and transported to VirG)** 2. **VirA transfers the phosphate group to VirG,** in which **VirG changes shape** 3. **Vir G producing an active transcription factor (component 2: response regulator), transcription begins** - Different two-component regulatory systems can allow microbes to respond differently to environmental stimuli - Pairing particular HPKs and RRs, cells can better control which genes are express in response to cues from the environment ### Chemotaxis - Complex bacterial behaviour modulated by **shifts in protein activity** - **sense changes in chemical gradients** over time - changes induce **altered direction and duration of flagellar rotation, leading to directed movement overtime** - **a modified two-component regulatory system towards or away from nutrients** #### Study of Chemotaxis Using Mutants - isolated using a capillary tube filled with nutrients (semi-solid capillary tubes) - microbes with **normal chemotaxis move into tube (move towards nutrients)** - **mutations remain outside the tube** (can't swim, genes broken) ### Regulation of Chemotaxis 1. Response to Signal 2. Control of flagella rotation 3. Adaptation (how do we know if it is going in the right direction?) (high or long sensitivity: **far, high sensitivity; close, low sensitivity)** #### Step 1: Response to Signal - Run in right direction, tumble in the wrong direction - MCPs sense specific attractants/repellants - Methyl-accepting chemotaxis proteins - Initiates signal transduction #### Step 2: Controlling of Flagella Rotation - CheY protein - Phosphorylated by CheA-P - CheY-P initiates **flagellar reversal: tumbling** #### Step 3: Adaptation - Feedback loop: - Allows system to **reset** itself - CheR -- adding methyl groups to the chemotaxis protein (always happening, changes sensitivity) - CheA also goes to CheB, starts removing methyl groups from CheB (sometimes remove them) - Allows temporal detection of signal concentration - Requires modification of MCPs by methylation - **When adding methyl groups, away from repellent (become fully methylated, most sensitive to repellents, least sensitive to attractants)** Here, it shows sensing a repellent (NOT GOOD!) #### Repellent Bound/No Attractant Bound NOT GOOD Mechanism: 1. Phosphorylation of CheA 2. Transfer CheA to CheY 3. CheY is phosphorylated 4. Changes direction of protein (tumble, **away**) 5. CheZ dephosphorylate it #### Move away from repellent /No repellent / Attractant Bound GOOD Mechanism - Methyl-accepting chemotaxis proteins (MCPs) also work in chemotaxis systems - By interacting with CheW proteins, autophosphorylation of CheA is modulated - **Attractants** **decrease phosphorylation; repellants increase phosphorylation** - **Provide for longer sustained runs of directed motion when attractants are present** - **Methylation of MCPs** also regulates **attraction** during periods of very high attractant levels in a process known as **adaptation** - **Highly methylated MCPs** will only respond to **very high levels of attractant** - High level not maintained, phosphorylation of CheA/CheB will lead to eventual demethylation of MCP - Results in greater sensitivity to the attractant, helping the system "reset" and avoid saturation over time #### **Chemotaxis Summary** #### **Chemotaxis Overview** #### **Process Table for Chemotaxis in Absence and Presence of Attractant** **Step** **Absence of Attractant** **Presence of Attractant** ---------- ------------------------------------------------------------------------------------------------------------- ------------------------------------------------------------------------------------ **1** **MCP (receptor)** is unbound by an attractant. **CheA** autophosphorylates using ATP. **MCP (receptor)** is bound by an attractant. **CheA** does not autophosphorylate. **2** The phosphate group is transferred from **CheA** to **CheY**, activating it (**CheY-P**). **CheY** remains unphosphorylated and inactive. **3** **CheY-P** interacts with the flagellar motor, causing it to turn **clockwise**, resulting in a **tumble**. The flagellar motor turns **counterclockwise**, producing a **smooth run**. **4** After about 10 seconds, **CheZ** removes the phosphate from **CheY-P**, stopping the tumble. No tumbling occurs as CheY remains inactive. **5** The cell alternates between runs and tumbles, moving in random directions. The cell moves in a smooth, directed motion toward the attractant. **Key Differences:** - **In absence of attractant**: The system promotes **tumbles** for random movement. - **In presence of attractant**: The system allows smooth movement (**runs**) toward the attractant. #### **Pathways of Low vs. High Attractant** **Low Attractant (MCP Unbound)** **Condition** **Process** **Result** ---------------------------- ----------------------------------------------------------------------------------------- ----------------------------------------------------------------------- 1\. Signal **MCP receptor** is not bound by attractant. No attractant signal is detected. 2\. Activation **CheA** autophosphorylates, becoming active. **CheA** transfers phosphate to **CheY**, activating it (**CheY-P**). 3\. Flagellar Motion **CheY-P** interacts with the flagellar motor, causing it to rotate **clockwise (CW)**. The cell enters a **tumbling** motion. 4\. Reset **CheZ** removes the phosphate from CheY-P (dephosphorylates it). The cell resets and alternates between runs and tumbles. 5\. Sensitivity Adjustment **CheB** (demethylase) removes methyl groups from MCP receptors (demethylation). Ensures receptors are ready to sense future attractants. **Condition** **Process** **Result** ---------------------------- ---------------------------------------------------------------------------------------------------- ----------------------------------------------------------------------------- 1\. Signal **MCP receptor** binds to the attractant. The attractant signal is detected. 2\. Inhibition **CheA** does not autophosphorylate (inactive). **CheY remains inactive**, so it doesn't interact with the flagellar motor. 3\. Flagellar Motion The flagellar motor rotates **counterclockwise (CCW)** continuously. The cell moves in a **smooth, directed run** toward the attractant. 4\. Sensitivity Adjustment **CheR** (methyltransferase) adds methyl groups to MCP. Increases receptor sensitivity to stronger attractant signals. 5\. Desensitization Once the attractant fades, **CheA reactivates**, and the process switches back to random movement. The system is reset for new stimuli. **High Attractant (MCP Bound)** **Key Notes:** 1. **Low Attractant**: - **Tumbling** helps the cell explore its surroundings randomly. - CheB ensures receptors are reset for future signals. 2. **High Attractant**: - **Smooth runs** help the cell move directly toward the attractant. - CheR adjusts receptor sensitivity to stronger signals and keeps the system responsive. ### Regulons #### Regulon definition - Sets of genes coordinated together, responding to same regulatory systems - **Catabolite repression**: shutdown of several systems that use various carbon sources when glucose is present - When **glucose is available**, the cell **turns off other systems that break down different carbon sources to save energy**. - **SOS response**: multigene system for wide scale DNA repair in response to serious DNA damage - When DNA gets badly damaged, the cell activates **a bunch of repair genes to fix it quickly.** - Two of the most important regulatory proteins for the **SOS response regulon** are ***recA and lexA*** - **Single stranded DNA exposed in the cell** **Condition** **SOS Regulon Repressed (No DNA Damage)** **SOS Regulon Induced (DNA Damage)** ------------------------- --------------------------------------------------------------------------------------------- ----------------------------------------------------------------------------------- **DNA State** DNA is intact and undamaged. DNA is damaged, creating single-stranded DNA (ssDNA). **RecA Activity** **RecA** is inactive because there is no ssDNA. **RecA** binds to ssDNA, becoming active and forming a complex. **LexA Repressor** **LexA** protein represses SOS genes by binding to their promoters, keeping expression low. Active RecA triggers LexA to cleave itself, removing the repression of SOS genes. **SOS Gene Expression** SOS genes remain **off or low-level expressed**. SOS genes are **highly expressed** to initiate DNA repair processes. **Repair Activity** No repair mechanisms are needed; DNA remains stable. SOS genes encode proteins to repair the DNA, like polymerases and helicases. **Result** The system stays inactive, conserving resources. DNA repair is initiated to fix the damage and protect the cell. **Key Takeaways:** 1. **Repressed State**: LexA keeps SOS genes off when there is no damage, maintaining stability. 2. **Induced State**: DNA damage activates RecA, which destroys LexA, allowing SOS genes to turn on and repair the damage. This ensures the cell only activates DNA repair mechanisms when necessary. #### Alternative Sigma Factors - In bacteria, use of different sigma factors directs RNA polymerases to certain genes - Most *E.Coli* Promoters are recognized by sigma-70 - **Sigma-54**: nitrogen utilization genes regulator (regular) - **Sigma-32**: heat shock protein gene regulator - **Sigma-38**: general stress response gene regulator (can switch things up for different functions)