Biochemistry Enzymes PDF
Document Details
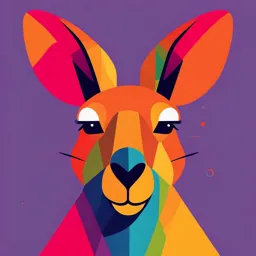
Uploaded by PopularRhodochrosite2288
Charles University
Tags
Summary
This document details lecture notes on enzymes, a key topic in biochemistry. It covers various aspects of enzyme functions, types and interactions, including catalytic mechanisms, and inhibition types. This resource provides a comprehensive overview for undergraduate biochemistry students.
Full Transcript
Functions (bindings) of proteins Functions of many proteins involve the reversible binding of other molecules (ligands) A ligand binds a protein at a binding site that is complementary to the ligand in size, shape, charge and hydro- character Proteins are flexible The binding of a...
Functions (bindings) of proteins Functions of many proteins involve the reversible binding of other molecules (ligands) A ligand binds a protein at a binding site that is complementary to the ligand in size, shape, charge and hydro- character Proteins are flexible The binding of a protein and ligand is often coupled to a conformational change in the protein that makes the binding site more complementary to the ligand, permitting tight binding In multisubunit protein, a conformational change in one subunit often affects the conformation of other subunits Two types of protein interactions 1) Protein, acting as a reaction catalyst (enzyme) alters the chemical configuration or composition of a bound molecule 2) Neither the chemical configuration nor the composition of the bound molecule is changed E.g. Reversible ligand binding of oxygen (Lehninger chapter 5.2) https://www.youtube.com/watch?v=Qv- KExGKAYw https://www.youtube.com/watch?v=K1S7STZ2BrA ENZYMES – lecture overview An introduction to enzymes How enzymes work Enzyme kinetics Examples of enzymatic reactions Inhibition of enzymes Introduction to Enzymes Life depends on powerful and specific catalysts: enzymes Almost every biochemical reaction is catalyzed by an enzyme Your body contains around 3,000 unique enzymes, each speeding up the reaction for one specific protein product Enzymes can make your brain cells work faster and help make energy to move your muscles They also play a large role in the digestive system, including amylases that break down sugar, proteases that break down protein, and lipases that break down fat All enzymes work on contact, so when one of these enzymes comes in contact with the right substrate, it starts to work immediately Key principles of enzymes Enzymes are powerful biological catalysts Enzymes exhibit a very high degree of specificity Enzymatic reactions occur in specialized pockets called active sites Two concepts explain the catalytic power of enzymes Many enzmes are regulated Brief History of Enzymes First discovered by Eduard Buchner in 1897 who observed that cell-free yeast extracts can ferment sugar to alcohol (Nobel prize 1907) - This proved that fermentation was promoted by molecules that continued to function when removed from cells The first enzyme to be purified and crystallized was urease in 1926 by James Sumner – these crystals consisted entirely of protein (Nobel prize 1946) Later, pepsin, trypsin and other digestive proteins were isolated and determined to be purely proteins as well Enzymes Biological catalysts which speed up the rate of reaction without becoming part of the reaction but themselves cannot initiate any chemical reaction With the exception of a few catalytic RNAs, all known enzymes are proteins Their catalytic activity depends on the integrity of their native protein conformation If an enzyme is denatured or dissociated into its subunits, catalytic activity is usually lost If an enzyme is broken down into its component amino acids, its catalytic activity is always destroyed Thus the primary, secondary, tertiary, and quaternary structures of protein enzymes are essential to their catalytic activity! Some enzymes require no chemical groups for activity other than their amino acid residues Others require an additional chemical component called a cofactor - either one or more inorganic ions, such as Fe2+ , Mg2+ , Mn2+ , or Zn2+ or A complex organic (often come from vitamins) or metalloorganic molecule called a coenzyme - a coenzyme or metal ion that is very tightly or even covalently bound to the enzyme protein is called a prosthetic group A complete, catalytically active enzyme together with its bound coenzyme and/or metal ions is called a holoenzyme The protein part of such an enzyme is called the apoenzyme or apoprotein Some Inorganic Ions That Serve as Cofactors for Enzymes Some Coenzymes That Serve as Transient Carriers of Specific Atoms or Functional Groups Enzymes are classified according to the type of reaction they catalyze How enzymes work Enzymes catalyse chemical reactions that do not normally proceed under conditions such as neutral pH, mild temperature, and aqueous solvent The site of catalytic activity of the enzyme is known as the active site The molecule that binds to the active site and is acted upon by the enzyme is called the substrate The two together form what is known as the enzyme-substrate complex The function of an enzyme is to increase the rate of a chemical reaction without affecting its equilibrium Therefore, enzyme do not make more product, they just make product faster Substrate binding site – active site 2 hypotheses into the mechanism: 1) lock and key model - Active site is complementary to the substrate - AA sidechains in the active site bind the substrate - Failed to explain the transition state 2) induced fit model - Enzyme structure is flexible, not rigid - Enzyme and active site adjust their shape to bind the substrate - Shape changes also improve catalysis during reaction by stabilizing the transition-state Binding of a substrate to an enzyme at the active site (The enzyme chymotrypsin with bound substrate) The structure has a unique geometric shape that is designed to fit the molecular shape of the substrate Understanding catalysis The function of a catalyst is to increase the rate of a reaction Catalysts do not affect reaction equilibria (Recall that a reaction is at equilibrium when there is no net change in the concentrations of reactants or products) Any reaction, such as S ⇌ P, can be described by a reaction coordinate diagram, a picture of the energy changes during the reaction Energy in biological systems is described in terms of free energy, G In the coordinate diagram, the free energy of the system is plotted against the progress of the reaction (the reaction coordinate) The starting point for either the forward or the reverse reaction is called the ground state (the contribution to the free energy of the system by an average molecule (S or P) under a given set of conditions) In biochemistry at pH 7 Reaction coordinate reflects the progressive chemical changes (e.g., bond breakage or formation) as S is converted to P. The activation energies, ΔG‡, for the S → P and P → S reactions are indicated. ΔG′° is the overall standard free energy change in the direction S → P (this reaction is exergonic) and at equilibrium there is more P than S (the equilibrium favors P) Recap window – chemical energetic reactions Endergonic and exergonic are two types of chemical reactions, or processes, in thermochemistry or physical chemistry. The names describe what happens to energy during the reaction. The classifications are related to endothermic and exothermic reactions, except endergonic and exergonic describe what happens with any form of energy, while endothermic and exothermic relate only to heat or thermal energy. https://www.thoughtco.com/endothermic-and-exothermic-reactions- 602105 https://www.thoughtco.com/endergonic-vs-exergonic-609258 How enzymes work A favorable equilibrium does not mean that the S → P conversion will occur at a detectable rate There is an energy barrier between S and P: the energy required for the alignment of reacting groups, formation of transient, unstable charges, bond rearrangements, and other transformations required for the reaction to proceed in either direction To undergo reaction, the molecules must overcome this barrier and therefore must be raised to a higher energy level. In the reaction S → P, the ES and EP intermediates occupy minima in the energy progress curve of the enzyme catalyzed reaction. The terms ΔG‡ uncat and ΔG‡ cat correspond to the activation energy for the uncatalyzed reaction and the overall activation energy for the catalyzed reaction Sucrose – sugar example again This general principle can be illustrated in the conversion of sucrose and oxygen to carbon dioxide and water: This conversion takes place through a series of separate reactions, has a very large and negative ΔG′°, and at equilibrium, the amount of sucrose present is negligible Yet sucrose is a stable compound, because the activation energy barrier that must be overcome before sucrose reacts with oxygen is quite high Catalytic Power and Specificity of Enzymes Enzymes are extraordinary catalysts The rate enhancements they bring about are in the range of 5 to 17 orders of magnitude Enzymes are also very specific, readily discriminating between substrates with quite similar structures Urease - catalyzes the hydrolysis of urea, forming ammonia and carbon dioxide. Found in large quantities in jack beans, soybeans, and other plant seeds, it also occurs in some animal tissues and intestinal microorganisms. How can we explain it? 1) By COVALENT bonding between E and S The rearrangement of covalent bonds during an enzyme-catalyzed reaction. Chemical reactions of many types take place between substrates and enzymes’ functional groups (specific amino acid side chains, metal ions, and coenzymes). Catalytic functional groups on an enzyme may form a transient covalent bond with a substrate and activate it for reaction, or a group may be transiently transferred from the substrate to the enzyme (at active site!). Covalent interactions between enzymes and substrates lower the activation energy (and thereby accelerate the reaction) 2) By NONCOVALENT interactions between E and S (Recall that weak, noncovalent interactions help stabilize protein structure and protein- protein interactions!). These same interactions are critical to the formation of complexes between proteins and small molecules, including enzyme substrates. Much of the energy required to lower activation energies is derived from weak, noncovalent interactions between substrate and enzyme. 1. Binding energy Formation of each weak interaction in the ES complex is accompanied by release of a small amount of free energy that stabilizes the interaction The energy derived from enzyme-substrate interaction is called binding energy Binding energy is a major source of free energy used by enzymes to lower the activation energies of reactions Much of the catalytic power of enzymes is ultimately derived from the free energy released in forming many weak bonds and interactions between an enzyme and its substrate This binding energy contributes to specificity as well as to catalysis Weak interactions are optimized in the reaction transition state 2. Acid-base catalysis Transfer of proton(s) P+ between an enzyme and a substrate or intermediate General acid-base catalysis becomes crucial in the active site of an enzyme, where water may not be available as a proton donor or acceptor Several amino acid side chains can and do take on the role of proton donors and acceptors These groups can be precisely positioned in an enzyme active site to allow proton transfers, providing rate enhancements of the order of 102-5 3. Covalent catalysis A transient covalent bond is formed between the enzyme and the substrate Consider the hydrolysis of a bond between groups A and B: In the presence of a covalent catalyst (an enzyme X:), the reaction becomes Formation and breakdown of a covalent intermediate creates a new pathway for the reaction, but catalysis results only when the new pathway has a lower activation energy than the uncatalyzed pathway. 4. Metal ion catalysis Metals, whether tightly bound to the enzyme or taken up from solution along with the substrate, can participate in catalysis in several ways Ionic interactions between an enzyme-bound metal and a substrate can help orient the substrate for reaction or stabilize charged reaction transition states This use of weak bonding interactions between metal and substrate is similar to some of the uses of enzyme-substrate binding energy described earlier Metals can also mediate oxidation-reduction reactions by reversible changes in the metal ion’s oxidation state Nearly a third of all known enzymes require one or more metal ions for catalytic activity Chemotrypsin (a) A representation of primary structure, showing disulfide bonds and the amino acid residues crucial to catalysis. The active-site amino acid residues are grouped together in the three-dimensional structure. (b) A depiction of the enzyme emphasizing its surface. The hydrophobic pocket in which the aromatic amino acid side chain of the substrate is bound is shown in yellow. Key active-site residues, including Ser 195 , His 57 , and Asp 102 , are red. (c) The polypeptide backbone as a ribbon structure. Disulfide bonds are yellow; the three chains are colored as in part (a). (d) A close-up of the active site with a substrate (white and yellow) bound. The hydroxyl of Ser195 attacks the carbonyl group of the substrate (the oxygens are red); the developing negative charge on the oxygen is stabilized by the oxyanion hole (amide nitrogens from Ser 195 and Gly 193 , in blue). The aromatic amino acid side chain of the substrate (yellow) sits in the hydrophobic pocket. The amide nitrogen of the peptide bond to be cleaved (protruding toward the viewer and projecting the path of the rest of the substrate polypeptide chain) is shown in white. Factors influencing enzyme activity Enzyme kinetics Velocity = rate of reaction Enzyme inhibition Inhibitors: compounds that decrease or eliminate activity of an enzyme Can decrease binding of substrate (affect Km) or turnover number (affect kcat) or both Most drugs are enzyme inhibitors Inhibitors are also important for determining enzyme mechanisms and the nature of the active site Some examples: Antibiotics inhibit enzymes by affecting bacterial metabolism Nerve gases cause irreversible enzyme inhibition Insecticides – choline esterase inhibitors Many heave metal poisons work by irreversibly inhibiting enzymes especially cysteine residues Types of enzyme inhibitions Reversible inhibition - Reversibly bind and dissociate from enzyme - Activity of enzyme recovered on reoval of inhibitor – usually non-covalent in nature - competitive – I competes for the site (substrate mimic or analogue) - uncompetitive – I binds only to E-S complex - non-competitive (mixed) – I can bind to E and also to E-S complex; allosteric inhibition Irreversible inhibition - associate with enzyme - Activity of enzyme not recovered on removal – usually covalent in nature Reversible inhibition Allosteric regulation of enzymes A small molecule (ligand) can bind an enzyme and act as an effector or regulator to activate or inactivate its function In such cases, the protein is said to be under allosteric control -regulation is a result of conformational changes in the protein when the ligand is bound Irreversible inhibition: kills the enzyme Combine with or destroy a functional group on an enzyme that is essential for activity They usually form covalent linkages to the enzyme A special class of II is the suicide inactivators – unreactive until bound to the active site Designed to carry out the first few steps of a normal enzyme reaction, but instead of forming a product, they form a highly reactive compound that binds irreversibly to the enzyme Called also mechanism-based-inactivators Transition-state analogs stable molecules designed to assemble transition states - ES complex