Biochemistry Sheet Quiz 1 PDF
Document Details
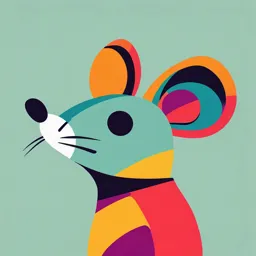
Uploaded by SweetBowenite4167
University of New South Wales
Tags
Summary
This document is a study sheet on Biochemistry, focusing on the structure and function of amino acids and proteins. It details the various types of amino acids and their properties, emphasizing how they form proteins. Additional information on protein structure and function is also part of the document's content.
Full Transcript
AMINO ACIDS Twenty kinds of side chains varying in size, PROTEINS Overview of protein functions shape, charge, hydrogen-bonding capacity, Protein structure level hydroph...
AMINO ACIDS Twenty kinds of side chains varying in size, PROTEINS Overview of protein functions shape, charge, hydrogen-bonding capacity, Protein structure level hydrophobic character, and chemical reactivity are commonly found in proteins. 1. Non-polar side chains Proteins are polymers of amino acid Depicted in prevailing ionic forms at pH 7 monomers. These polymers are commonly Tryptophan is the largest amino acid and glycine called polypeptides. is the smallest (and also sometimes classified as A protein consists of one or more polypeptides, polar) each folded into a specific three-dimensional Secondary structure refers to local regions of structure. the polypeptide chain that are stabilised by 2. Uncharged polar side chains There are 20 different amino acids that cells use hydrogen bonding between atoms of the to build their 1000s of proteins. polypeptide backbone All amino acids share a common structure: A central (alpha,α ) carbon atom attached to : – an amino group (NH3 + ); – a carboxyl group (COO- ); – a hydrogen atom (H); – and a variable side chain “R” (or R group) Non-essential - synthesised by mammals from All have side-chains that are polar with a net metabolic precursors neutral charge, except cysteine and tyrosine Essential - must be obtained from diet which have only weak charges Excess dietary amino acids are Cysteine forms disulfide bonds, the rest can neither stored nor excreted. Instead, participate in hydrogen bonding. Anti-parallel β sheet. adjacent β strands they are converted to metabolic run in opposite directions. Hydrogen bonds intermediates that are also 3. Charged polar side chains between NH and CO groups connect each precursors of glucose, fatty acids and amino acid on an adjacent strand ketone bodies Thus, they are considered metabolic fuels All are hydrophilic with net charges on their side-chains. The acidic amino acids have carboxyl groups in Parallel β sheet. Adjacent β strands run in the their side-chains which have a net negative same direction. Hydrogen bonds connect each charge. amino acid on one strand with two different Only L amino acids are constituents of proteins amino acids on the adjacent strand The basic amino acids: histidine has an imidazole group with a weak positive charge; lysine has an amino group with a strong positive charge and arginine has a guanidinium group with a very strong positive charge. Peptide bond formation The ionisation state of amino acids is altered by a change in pH The zwitterionic form predominates near physiological pH (~7) Weak interactions contributing to the tertiary structure of a protein: – Hydrophobic interactions – Hydrogen bonds – Ionic or electrostatic bonds Although these are weak bonds, their cumulative effect helps give the protein a specific shape. Much stronger are the disulfide bridges, covalent bonds between the side chains of two cysteine amino acids The rate of reaction is directly proportional to Free energy of activation, EA the enzyme concentration STRUCTURAL Chemical reactions between molecules involve Fibrous Proteins: Elongated, water-insoluble the breaking and formation of bonds. structural proteins, e.g. keratin (hair), muscle The initial amount of energy required to start a proteins, fibrin (blood clot), collagen. chemical reaction is called the free energy of Globular Proteins: These have backbones that activation, or activation energy (EA ). fold in on themselves to produce a more spherical, compact and water-soluble structure, Enzymes lower the energy barrier (activation e.g. enzymes, globins. energy) of a thermodynamically favourable reaction, thus increasing the rate of that reaction. This is called catalysis. Enzymes DO NOT affect the equilibrium or free energy change (∆G) of the reaction and cannot A plot of the reaction velocity (V0) as a function make a thermodynamically unfavourable of the substrate concentration [S] reaction favourable! Protein denaturation High temperature, mechanical forces and various chemical treatments -> can denature a protein, causing it to lose its conformation and hence its ability to function Formation of the enzyme-substrate complex Enzymes often require a non-protein component for activity. Cofactors may be metal ions or more complex organic molecules (coenzymes). Water soluble vitamins (“B” vitamins and vitamin C) are either coenzymes or converted into coenzymes. Experimentally a protein can often be re- Enzymes have active sites where catalysis takes Enzyme inhibition natured when the chemical and physical aspects place. Enzymes can be inhibited by specific molecules of its environment are restored to normal Part of the active site is the substrate binding Competitive inhibitors mimic the substrate, site. bind at the active site and block substrate ENZYMES Substrate binding may result in conformational binding. changes (an “induced fit” model) Non-competitive inhibitors bind at a separate Enzymes are biological catalysts or catalytic site, preventing catalysis. proteins Inhibition is commonly reversible (like substrate A catalyst is a chemical agent that changes the binding), but may be irreversible if a covalent rate of a reaction without being consumed by bond is formed between the inhibitor and an the reaction amino acid side chain Enzymes are highly specific -With respect to the reactions they catalyse -With respect to the choice of reactants (substrates) Enzymes have great catalytic power (can increase rates of reactions by > x106) Enzymes speed up the attainment of equilibrium without affecting the position of equilibrium Many enzymes require other components for Some of the main factors affecting enzyme activity (e.g. cofactors or coenzymes) activity include: In the absence of enzymes, chemical reactions Enzyme concentration [E] within cells would not occur rapidly enough to Substrate concentration [S] sustain life Temperature pH Cofactor requirements Inhibitors Competitive inhibition The KM for an enzyme depends on the particular substrate and on environmental conditions such as pH, temperature and ionic strength. Reaction velocity vs substrate concentration For most enzymes KM lies between 10–1 and 10– Non-competitive inhibition A plot of the reaction velocity as a function of 7M. the substrate concentration shows that the KM is the substrate concentration at which HALF maximal velocity is approached asymptotically. the ACTIVE SITES are filled. Thus, KM provides a Most enzymes yield a hyperbolic curve, as measure of the substrate concentration shown required for significant catalysis to occur. Case study - Aldehyde dehydrogenase Normally, alcohol dehydrogenase in the liver converts ethanol to acetaldehyde ENZYMES KINETICS Acetaldehyde is the cause of the symptoms Kinetics = the study of chemical reaction rates when at high concentrations, but it is normally Consider the simple reaction where reactant A processed to acetate by aldehyde is converted to product P : A --> P The Michaelis - Menten model dehydrogenase The rate, or velocity (v), of this reaction can be determined by measuring the amount of P that forms or the amount of A that disappears per unit of time (t): Rate = v = change in [P] / t or change in [A] / t E = enzyme; S = substrate; P = product. Most people have 2 forms of aldehyde The units for v are usually moles (or mMoles, or There are two possible fates of the ES complex. dehydrogenase: a low KM mitochondrial form µMoles) per minute or second The terms k1 , k -1 , k2 and k -2 define rate and a high KM cytoplasmic form. For a reaction to occur molecules must collide constants for the individual steps. In susceptible persons, the mitochondrial or bump into each other in the proper To simplify the discussion of enzyme kinetics, enzyme is less active due to a mutation (single orientation and with sufficient kinetic energy the reverse reaction of product forming amino acid substitution). to break chemical bonds substrate (k -2 ) is ignored. Temperature and reactant concentration The maximal rate, Vmax, reveals the TURNOVER influence these conditions The Michaelis-Menten equation NUMBER of an enzyme. RATE equation - for a reaction with a single It is the number of substrate molecules reactant : A --> P converted into product by an enzyme molecule Rate = v = k[A] in a unit of time when the enzyme is fully Where k is the rate constant (units min -1 or sec- saturated with substrate. 1) which is a proportionality factor that accounts It is equal to the kinetic constant k 2 (kcat) for how well the reacting molecules are Where : oriented. v0 = initial velocity caused by substrate For a reactions with two reactants : A + B --> P : concentration, [S]. V = k[A][B] Vmax = maximum velocity. k2 + k3 Where [ET] = total enzyme concentration The units for k then become (concentration) -1 KM = k1 = Michaelis-Menten constant. (time)-1, e.g. M-1 (sec)-1 The turnover number of most enzymes with their physiological substrates fall in the range Michaelis-Menten Kinetics The kinetics properties of enzymes from 1 to 104 per second The initial rate of reaction (initial velocity, v0 ) increases linearly as substrate concentration Allosteric enzymes are an important group of increases, but then begins to level off and enzymes, but they DO NOT obey Michaelis- approach a maximum value at higher substrate Menten kinetics! concentrations. Consist of multiple subunits and multiple active This catalytic behaviour can be described by a sites. substrate saturation effect. Often display sigmoidal plots of reaction velocity versus substrate concentration (rather than hyperbolic plots). Binding of substrate to one active site can affect The Lineweaver - Burke Plot properties of other active sites, e.g. substrate A plot of reaction velocity (v0 ) as a function of binding can become “cooperative”. substrate concentration [S] does not permit completely accurate determinations of Vmax and KM because the maximal velocity is approached asymptotically. However, Lineweaver and Burke found that if we turn the Michaelis-Menten equation Determining initial reaction velocity “upside-down”, the intercepts on the resulting Allosteric regulation may either REMEMBER: All reactions eventually reach an double-reciprocal plot allow us to determine inhibit or stimulate enzyme equilibrium in which there is no net change in Vmax and KM values directly!!! activity. the concentration of substrate or product The reciprocal of the Michaelis-Menten Allosteric regulation occurs The amount of product formed at different equation : when a regulatory molecule substrate concentrations is plotted as a binds to an enzyme at one site, and affects the function of time. function at another site. The initial reaction velocity (v0) for each Note that the active site(s) and allosteric sites of substrate concentration is determined from an enzyme are different! slope of curve at the beginning of reaction (when reverse reaction is insignificant). CARBOHYDRATES Because biological interactions between Sucrose is an example of a di-saccharide, a molecules are stereospecific, the position of H molecule composed of two monosaccharides Adenosine triphosphate (ATP) is formed by and OH groups around C atoms is important! attachment (condensation) of the phosphate group on adenosine diphosphate (ADP). Two sugars that differ only in the configuration around one carbon atom are called epimers For long-term storage, plants make polymers of glucose release of energy, which is used to drive other Amylose is a linear polymer of glucose processes, e.g. movement of organelles (1 ATP molecule per 8.1 nm) BUT, ATP is a short-lived molecule, whereas plants also need energy when they do not have access to light. Enantiomers are mirror images of each other D- and L-glucose are enantiomers glucose units are linked in a linear way with α(1→4) glycosidic bonds; water insoluble, non-sweet hydrolyzed slowly dense Amylopectin is a branched polymer of glucose formed by plants store energy Most of the hexoses of living organisms are D isomers. A monosaccharide is the simplest form of carbohydrates produced by plants, which is 16 stereoisomers of aldohexose created by combining water and carbon dioxide with the release of oxygen, and have the following chemical formula: CX(H2O)X X molecules of CO2 + X molecules of H2O + light - > CxH2xOx + X molecules of O2 Monosaccharides are the simplest forms of Linear amylose and branched amylopectin form sugars, which can be classified according to the starch number of C atoms Plants store starch within specialized organelles called amyloplasts. When energy is needed for cell work, the plant hydrolyzes the starch releasing the glucose subunits. Plants also form polymers of glucose, cellulose, providing structural support. Cellulose: is formed by glucose units linked in a linear way via β(1→4) glycosidic bonds has no taste cannot be digested by animals can be broken down by some bacteria, fungi Anomers are epimers which differ in the component of dietary fibre position of H and OH groups around the C1 All monosaccharides contain a carbonyl group atom in the cyclic forms of the monosaccharides. In solution, glucose predominantly exists as α and β anomers and each molecule of glucose Glycogen is the polymer of glucose used by continuously converts from one form to another animal cells to store energy via mutarotation Glucose and fructose have the same chemical formula but differ in the type of the carbonyl group Polysaccharides Homopolysaccharides Heteropolysaccharides -formed by at least -contain two or more six identical different monosaccharide Monosaccharides can also differ in the monosaccharides units arrangement of H and OH groups around the - have a well-defined - most naturally occurring asymmetric (chiral) centres chemical structure contain only two different - examples: glycogen, ones and are closely starch, cellulose associated with lipid or protein - examples: pectin, lignin, glycoproteins, glycolipids, and mucopolysaccharides GLYCOLYSIS 1. catalysed by hexokinase, which 7. Phosphoryl Transfer from 1,3- phosphorylates glucose to prevent its transport Bisphosphoglycerate to ADP by In our digestive system, complex carbohydrates from the cell phosphoglycerate kinase results in generation are disassembled into simple sugars with the The reaction is highly spontaneous and of ATP help of enzymes irreversible under cellular conditions. It acts to keep glucose in the cell, because cells can transport glucose across the membranes but lack transporters for D-Glucose-6-phosphate 8. Conversion of 3-Phosphoglycerate to 2- Phosphoglycerate 9. Dehydration of 2-Phosphoglycerate to From the digestive system, monosaccharides Phosphoenolpyruvate 2. phosphoglucose isomerase converts glucose are transported to the cells of the small the loss of the water molecule from 2- 6-phosphate to fructose 6-phosphate intestine and then to blood with the help of phosphoglycerate causes a redistribution of The reaction is reversible and is necessary to proteins, glucose transporters energy within the molecule prepare the molecule for the next steps – phosphorylation and cleavage 10. Transfer of the phosphoryl group from phosphoenolpyruvate to ADP by pyruvate kinase results in generation of ATP 3. phosphofructokinase phosphorylates the molecule second time Lactose intolerance is an example of a condition the reaction is irreversible under cellular caused by inefficient hydrolysis of disaccharides conditions Lactose is hydrolysed to monosaccharides by Balance sheet of beta galactosidase, a.k.a. lactase glycolysis : Mammals are unlikely to encounter lactose after they are weaned, so the level and activity of lactase are low in adult. Non-hydrolysed lactose moves through the digestive tract to the colon, fermented and large 4. aldolase cleaves fructose-1,6- bisphosphate quantities of CO2, H2 and acids are produced and generates two similar products Glycolysis releases only a fraction of energy This reaction is reversible, The products are stored in glucose From the blood, glucose enters cells in our body similar but not identical via different transporters located at the cell surface Importance of glycolysis Glycolysis is anaerobic (can take place in the 5. triosephosphate isomerase converts absence of oxygen) so it can provide ATP for dihydroxyacetone into glyceraldehyde-3 muscle during strenuous exercise phosphate. As a result, the preparatory phase It can provide ATP extremely rapidly – suitable Cells recycle ATP via glycolysis → process of leads to generation of two identical molecules for muscle contraction glucose degradation of glyceraldehyde-3 phosphate (note, that 1 Oxidative phosphorylation occurs in special molecule of glyceraldehyde-3 phosphate is organelles called mitochondria. Glycolysis is Glycolysis is a set of 10 reactions in the cytosol produced by aldolase). used as a primary mechanism for ATP production in cells that don’t have mitochondria Energy Supply During Exercise -ATP: up to 4 seconds. High jump, power lift, shot put, tennis serve -Phosphocreatine: up to 10 seconds. sprints, American football line play Preparation is completed! -Glycolysis: up to 1.5 minutes. 200-400 m race, 100 m swim. In the payoff phase, two identical cleavage -Oxidative phosphorylation: race beyond 500 products are converted into pyruvate with the meters release of energy using the same set of reaction Even under aerobic conditions, glycolysis is the major starting point for carbohydrate 6. oxidation of Glyceraldehyde 3- phosphate to metabolism 1,3-Bisphosphoglycerate with the help of dehydrogenase NAD+ - Nicotinamide adenine dinucleotide, a coenzyme found in all living cells TCA CYCLE Part 2C – only molecule of GTP or ATP formed Depletion of the TCA cycle components will result in reduced function of the cycle Part 3A – regeneration of oxaloacetate The TCA cycle occurs in the mitochondrial matrix The TCA cycle : regulation Part 3B – regeneration of oxaloacetate Cells can move mitochondria with the help of molecular waters and strategically position in places energy is most needed. Overview of TCA cycle Part 3C – regeneration of oxaloacetate Part 1 – the tricarboxylic acids The five reactions of the pyruvate dehydrogenase multienzyme complex Part 2A – the formation of CO2 Balance sheet of the TCA cycle Disorders caused by dysfunction of the TCA cycle α-Ketoglutarate dehydrogenase complex deficiency Only 1 molecule of ATP is produced. - impaired brain energy metabolism Part 2B – the formation of CO2 - causes infantile lactic acidosis, psychomotor The cycle produces NADH and FADH2 which are used by the electron transport chain to produce retardation in childhood, intermittent 2.5 ATP per 1 NADH and 1.5 ATP per 1 FADH2 neuropsychiatric disease with ataxia and other motor manifestations, Friedreich’s and other NAD+ and FAD have to be replenished. spinocerebellar ataxias, Parkinson’s disease, and They can be regenerated in the mitochondrion Alzheimer’s disease (AD). only by transfer of electrons to molecular Succinate dehydrogenase deficiency oxygen, i.e. in contrast to glycolysis the cycle - Leigh syndrome (a neurodegenerative can operate only under aerobic conditions condition) α-Ketoglutarate dehydrogenase complex is - paraganglioma and pheochromocytoma similar to the pyruvate dehydrogenase complex Functions of the TCA cycle (cancer) Oxidises an acetyl- group (from acetylCoA) to 2 Fumarase deficiency CO2 Produces reduced cofactors (3 NADH and 1 - Early encephalopathy, seizures, and muscular FADH2) which can be re-oxidised by the hypotonia respiratory chain to produce ATP Is the common - Leiomyomatosis and papilliary renal cell final stage in the catabolism of fatty acids, cancer amino acids, and carbohydrates Succinyl-CoA synthetase deficiency Pyruvate dehydrogenase complex: E1, E2, E3 - Encephalomyopathy and mtDNA depletion subunits α-Ketoglutarate dehydrogenase complex: E1k, E2k, E3 subunits REGULATION Glycolysis must be slowed down when the In yeasts, regeneration of NAD+ from NADH in energy needs of the cells are met to prevent the absence of oxygen is coupled to formation Levels of AMP, ADP and ATP determine the accumulation of unwanted sugars of ethanol and CO2 (the anaerobic or energy state of the cells fermentation pathway in yeast) Wine & beer industry Yeasts carry out fermentation in the production of ethanol in beers, wines and other alcoholic drinks, along with the production of large quantities of carbon dioxide. Baking industry CO2 released during fermentation causes dough to rise Yeast consume far more sugar when growing Summary of the key enzymes in the regulation In skeletal muscle during exercise and in red anaerobically than when growing aerobically of the glycolysis: blood cells in mammals, regeneration of NAD+ (the “Pasteur effect”). This observation Hexokinase (step 1): Glucose + ATP -> glucose- from NADH is coupled to the anaerobic indicates that glycolysis is regulated. 6-phosphate + ADP is inhibited by glucose-6- fermentation of lactate Yeasts contain mitochondria and can obtain phosphate (product inhibition) ATP via glycolysis (5%) and oxidative Phosphofructokinase (step 3): Fructose-6- phosphorylation (95%) in the presence of phosphate + ATP -> fructose-1,6-biphosphate + oxygen. In anaerobic conditions, oxidative ADP is activated by ADP and AMP, inhibited by phosphorylation is not possible and only ATP and NADH glycolysis can be used to generate ATP. To Pyruvate kinase (step 10): maintain ATP levels, yeasts need more glucose, Phosphoenolpyruvate + ADP -> pyruvate + ATP because glycolysis is less efficient. Yeasts also is inhibited by ATP need to enhance glycolysis to process more Skeletal muscle and red blood cells release glucose and generate the same amount of Liver cells store excess glucose when it is not lactate to the bloodstream and it is recycled to energy as in the presence of oxygen needed for other cells by expressing glucokinase glucose in liver (Cori cycle named after Carl and instead of hexokinase Gerty Cori) In a low energy state, the speed of glycolysis is Hexokinase Glucokinase enhanced via an increase in the activity of -ubiquitous -liver cell phosphofructokinase, a rate limiting enzyme -end product : -end product : glucose-6- glucose-6- phosphate phosphate -high affinity to -low affinity to glucose glucose -initial step in -traps only excess glycolysis glucose to store as glycogen Summary : the fate of pyruvate Glucose comes from the diet. The ATP is utilized for cellular processes and is recycled to ADP. PROBLEM: Cells need NAD+ for glycolysis to continue. An increase in fructose 6-phosphate levels leads Cells need to prevent accumulation of NADH to generation of the fructose 2,6 bisphosphate, and pyruvate which allosterically activates Cells solve these problems differently phosphofructokinase depending on whether they have access to oxygen. We must consider: 1. Aerobic conditions (abundant oxygen) In the presence of oxygen, pyruvate is 2. Anaerobic conditions (limiting oxygen) transported from the cytosol to the mitochondrial matrix by the pyruvate In the presence of oxygen, regeneration of translocase NAD+ from NADH is coupled to oxidative In the mitochondrial matrix, pyruvate is phosphorylation (the aerobic pathway) converted into the acetyl-CoA by pyruvate dehydrogenase. This process is called pyruvate decarboxylation and is the entry to the TCA Glycolysis rates depend on the transport of cycle. glucose to the cytosol. The efficiency of the transport is determined by the numbers of The pyruvate dehydrogenase complex (PDH) is a glucose transporters at the cell surface large, highly integrated complex of three kinds Glucose transporters are stored in vesicles, of enzymes which deliver the transporters to the cell This is the pathway used by all cells with surface when glucose levels increase mitochondria (e.g. yeasts or cells in our body) REGENERATION OF NAD+ FROM NADH the anaerobic pathway: Fermentation Fermentation is a process, which extracts energy (as ATP) but does not consume oxygen or change the concentrations of NAD+ or NADH. Fermentations are carried out by a wide range of organisms, many of which occupy anaerobic niches. The five reactions of the pyruvate Oxidative phosphorylation is the synthesis of dehydrogenase multienzyme complex ATP powered by the free energy of reduced compounds that are produced by other metabolic pathways, including glycolysis and the TCA cycle. FREE ENERGY refers to the portion of a molecule’s or biological system’s energy that is available to perform work (when temperature and pressure are uniform throughout the surrounding environment). E1, E2 and E3 associate with each other to form Gibbs’ free energy ΔG the pyruvate dehydrogenase complex ΔG negative = spontaneous INTEGRAL Proteins: ΔG positive = non-spontaneous Intercalated into lipid bilayer through hydrophobic interactions. REDUCED COMPOUNDS are those that have Transmembrane proteins are the predominant gained one or more electrons. In the case of group of integral proteins. oxidative phosphorylation, they are reduced PERIPHERAL Proteins: cofactors such as NADH and FADH2. Non-covalently bound to one face of the During oxidative phosphorylation, cells use the membrane, usually through another protein. free energy stored in molecules like NADH and FADH2 to drive ATP synthesis. Oxidation reduction reaction : One reactant (the oxidising agent) is reduced as Pyruvate Dehydrogenase is a multienzyme it gains electrons. complex. The other reactant (the reducing agent) is oxidised as it gives up electrons OIL RIG Multienzyme complexes are groups of noncovalently associated enzymes that catalyse two or more sequential steps in a metabolic pathway. Advantages: Fluid mosaic model of membrane structure 1.Enzymatic rates are limited by the frequency The energy released in electron transfer with which enzymes collide with their processes (redox reactions) depends on the substrates. When a series of reactions occur energy levels of the oxidising and reducing within a multienzyme complex, the distance agents. that substrates must diffuse between active The relative energy levels of these agents are sites is minimized. quantified using standard reduction potentials, 2.The channelling of metabolic intermediates E ′ between successive enzymes in a metabolic The reduction potential (measured in volts, V) pathway reduces the opportunity for these indicates the tendency of a substance to accept The mitochondrion intermediates to react with other molecules. electrons. 3.The reactions can be co-ordinately controlled. Electrons flow spontaneously from the substance with the lower reduction potential to Pyruvate Dehydrogenase complex deficiency the substance with the higher reduction causes diseases: potential. - Pyruvate dehydrogenase complex deficiency caused by mutations in genes coding for the E1 Mitochondrial electron transport chain Matrix → TCA cycle subunit is a type of metabolic disease. The Inner mitochondrial membrane → Oxidative signs appear in infancy and include brain phosphorylation of ETC → ATP synthesis abnormalities, low weight, high levels of lactate, extreme tiredness, rapid breathing and other OUTER Mitochondrial Membrane symptoms. People often pass away in the first Approximately 50% lipid and 50% protein years of life. Relatively permeable (porin protein) - Deficiency in thiamine (B1) pyrophosphate Presence of some enzymes bound to E1 causes beriberi INTERMEMBRANE Space OXIDATIVE PHOSPHORYLATION Presence of some enzymes e.g. the myokinase, adenylate kinase, which catalyses: “Cellular respiration” is the term commonly In the mitochondrial electron transport chain, ATP + AMP ADP + ADP used to describe the process by which cells NADH (best electron donor) has the lowest E 0 ’ break down organic molecules using various and O2 (best electron acceptor) has the highest INNER Mitochondrial Membrane catabolic pathways for the purpose of E0’ More complex (~ 80% protein) generating energy (ATP). Intrinsically impermeable Contains: Extracting energy from food involves the -Many carrier proteins transfer and relocation of electrons during the -Peripheral and integral proteins chemical reactions releases the energy stored in -The electron transport (respiratory) chain the organic molecules and this energy is -ATP synthesising enzymes ultimately used to synthesise ATP. -Dehydrogenase enzymes -(e.g. succinate dehydrogenase – TCA cycle) Phospholipids are an essential type of lipid MITOCHONDRIAL MATRIX because they make up cell membranes. In Pyruvate dehydrogenase complex aqueous media, phospholipids spontaneously TCA cycle (except succinate dehydrogenase) form a bimolecular sheet which is only a few Fatty acid oxidation (β-oxidation) molecules thick. This structure is called a lipid Part of the urea cycle bilayer. Miscellaneous biochemical activities COMPLEX II : SUCCINATE COENZYME Q Cytochromes a and a3 are the only two carriers ELECTRON TRANSPORT CHAIN REDUCTASE in the electron transport chain that are capable of transferring electrons directly to O2. Electrons are removed from nutrients and metabolic intermediates during oxidative reactions. They are transferred by dehydrogenase enzymes to the cofactors NAD+ and FAD. Thus, electrons from substrates are collected in the form of reduced cofactors NADH and FADH2 Since cells contain a limited supply of oxidised cofactors NAD+ and FAD, they must be recycled Doesn’t pump out H+ to form hydrogen As electrons flow from NADH or FADH2 through from the reduced forms if metabolism is to be gradient. Energy release isn’t big enough the various complexes to O2 , protons are continuous Embedded half way of the membrane→integral simultaneously pumped from the mitochondrial matrix to the intermembrane space. The chain is composed of four large protein The proton gradient across the inner complexes: mitochondrial membrane provides the energy for ATP formation Energy – coupling site Carriers are arranged in order of increasing electron affinity so that electrons can flow spontaneously from one complex to the next. There are at least two forms of Complex II: One that accepts electrons from FADH2 made Electrons from NADH are passed to Complex I in the TCA cycle while electrons from FADH2 enter the electron One that accepts electrons from FADH2 made transport chain via Complex II. by fatty acid breakdown. Both have Fe-S clusters as prosthetic groups Complex I,II,III,IV are embedded in the inner and are present as integral proteins in the inner Oxidative phosphorylation is the coupling of two mitochondrial membrane mitochondrial membrane. distinct processes: Similar to Complex I, at the end of Complex II is the carrier ubiquinone (coenzyme Q or CoQ), which accepts two electrons and two protons to be reduced to ubiquinol (CoQH2 ). COMPLEX III: CYTOCHROME C REDUCTASE There are four molecular types of redox centres in the complexes: flavin mononucleotide (FMN), iron-sulfur clusters (Fe-S), ubiquinone (CoQ) and cytochromes (e.g. cyt c) Energy is released in incremental amounts at Complex III transfers electrons from ubiquinol each redox step and is collected in the form of a (CoQH2 ) to cytochrome c (cyt c). There are several different chemicals that transmembrane proton gradient that is used to The electron carriers in Complex III include Fe-S inhibit electron flow at specific points in the drive the synthesis of ATP clusters and cytochromes. chain. Cytochromes are electron transferring proteins Studies with these electron flow inhibitors COMPLEX I : NADH COENZYME Q REDUCTASE that are highly coloured(red) due to the helped biochemists to elucidate the sequence presence of a heme prosthetic group. of electron carriers in the chain Since CoQH2 is a two-electron donor and the cytochromes are one-electron acceptors, electrons entering Complex III via CoQH2 separate into interlinking paths known as the Q cycle. The Q cycle is thought to assist in maintaining the proton gradient necessary for ATP The redox centres associated with Complex I synthesis. include: flavin mononucleotide (FMN) and an iron-sulfur clusters (Fe-S). COMPLEX IV: CYTOCHROME C OXIDASE FMN, the first carrier in the complex, accepts two electrons from NADH and is reduced to FMNH2. Electrons from FMNH2 are then passed to the iron-sulfur clusters. At the end of Complex I is the carrier ubiquinone (also called coenzyme Q or CoQ), which accepts two electrons and two protons to be reduced to ubiquinol (CoQH2 ) Complex IV consists of ten protein subunits with two types of prosthetic groups, two hemes (cytochromes a and a3 ), and two copper atoms (CuA and CuB). On their way from cytochrome c to O2 , electrons flow through the Fe atoms in the two hemes and the copper atoms. ATP SYNTHESIS ATP synthesis occurs as follows: 1. The substrates ADP and Pi are trapped in the Glycerol-3-Phosphate shuttle This is often expressed as the P/O ratio: the β subunit in the loose -This shuttle is used predominately by skeletal number of molecules of ATP that are formed state. muscle cells. per pair of electrons passed through the 2. Substrates are -NADH from glycolysis bypasses complex 1 transport chain to oxygen. converted to ATP as -Therefore, it only produces 1.5 ATP molecule rotation of the γ subunit per NADH. causes the β subunit to -In contrast, NADH from the TCA cycle produces shift to the tight 2.5 ATP molecule per NADH. conformation. 3. The product ATP is These results can be explained by the presence released after the next of three discrete coupling sites along the rotation, when the β electron transport chain subunit shifts to the open conformation. Once the Coupling process ATP is released into the Electron transport through the carriers in the matrix a new ADP and Pi inner mitochondrial membrane causes the will enter unidirectional pumping of protons from the mitochondrial matrix to the other side of the Malate-Aspartate shuttle membrane (the intermembrane space). This shuttle is used predominately by heart and Chemiosmotic theory summary Chemical energy from the respiratory oxidation- liver cells. The imbalance of protons represents a source Here, NADH from glycolysis is regenerated in reduction reactions is transduced to a proton- of free energy, called a proton motive force, the matrix and it can pass on electrons at motive force (electrochemical gradient), then to that can drive the activity of an ATP synthase complex 1. the mechanical movement of a rotary engine (the c-ring and its attached γ shaft), and finally Therefore, it produces 2.5 ATP molecule per ATP Synthase NADH. back to chemical energy in the form of ATP The Stoichiometry of Proton Extrusion by Respiratory Complexes -The protein that utilises the electrochemical The Stoichiometry of Proton Influx and ADP Electrons do not flow down the electron proton gradient to phosphorylate ADP is known Phosphorylation: transport chain to O2 unless ADP and Pi are as the F1F0 ATP synthase (or Complex V). It present for phosphorylation. Therefore, the consists of two main units: F1 and F0. flow of electrons is regulated by the -The F0 component functions as a concentration of ADP transmembrane channel that permits the flow ATP Yield from the Oxidation of NADH and of protons (H+ ) back into the matrix. FADH2 About 4 protons are required per ATP -The F1 synthesised, so if… component contains catalytic sites for ATP synthesis, where ADP and Pi are brought together for combination. ADP/O or ADP/2e ratios for NADH and FADH2 Inhibitors of Oxidative Phosphorylation -Conformational Oxidative phosphorylation can be inhibited at changes in the various stages of the process: F1F0 complex Electron transport (last lecture) resemble the ATP synthesis rotation of a The coupling process motor shaft and ATP-ADP translocation release ATP from the bound ADP Uncouplers and Pi with each turn. Uncouplers work by carrying protons across the inner mitochondrial membrane. Electron Binding change mechanism transport proceeds normally, but ATP is NOT The binding change mechanism describes how formed because the proton-motive force across rotation-driven conformational changes alter the membrane is “short-circuited” by the the affinity of each catalytic β subunit for uncoupler. adenine nucleotides. Transport across the mitochondrial At any moment, each catalytic site has a membranes different conformation and binding affinity, -Cytoplasmic NADH (e.g. generated by referred to as the open (O), loose (L) or tight (T) glycolysis) cannot normally be transported state. through the inner mitochondrial membrane to The next schematic shows the catalytic β the matrix. subunits of the F1 component of the ATP -Therefore, cytoplasmic NADH must be recycled synthase and illustrates the binding change by electron shuttle systems that carry electrons mechanism. through the membrane in the form of reduced substrates. If ATP synthesis were partially uncoupled from -The glycerol-3-phosphate shuttle functions in the respiratory chain, more respiratory chain skeletal muscle and brain. When using this function would be required to make the same shuttle, cytoplasmic NADH leads to the amount of ATP. → More catabolism of formation of only 1.5 ATP. macronutrients would be required, and weight -The malate-aspartate shuttle is present in loss would be assisted. Energy would be wasted heart and liver. This shuttle gives rise to the as heat. generation of the usual 2.5 ATP for each cytoplasmic NADH GLYCOGEN METABOLISM Overview of glucose metabolism 3.Phosphoglycomutase Living organisms can use glycogen or starch to -Converts the G1P units produced by store glucose for later metabolic use. phosphorylase to G6P. Animals, fungi, bacteria → glycogen -The enzyme transfers a phosphoryl group from Plants →starch its serine residue to the C6 position of G1P to form G1,6-bisP. -The phosphoryl group from the C1 position of G1,6-bisP is then transferred back to the serine residue of the enzyme Glycogen breakdown Glucose units are mobilised by their sequential Glycogen function removal from the nonreducing ends of Storage polysaccharide of animals. glycogen. Glycogen breakdown, also called Present in all cells but most prevalent in skeletal GLYCOGENOLYSIS, requires three enzymes: muscle and liver, where it occurs as cytoplasmic 1.glycogen phosphorylase granules. 2.glycogen debranching enzyme Muscle cells contain up to 1-2% glycogen by 3.phophoglycomutase weight, while liver cells contain up to 10% Fates of G6P: glycogen by weight. (more total muscle cells The G6P produced by glycogen breakdown can 1.Glycogen Phosphorylase than liver cells, total glycogen stored in skeletal continue along the glycolytic pathway or the -Catalyses the rate-controlling step in glycogen muscles is more than glycogen storage in liver pentose phosphate pathway (not covered in breakdown. cell) more quantity BIOC2181). -Is regulated by both allosteric interactions and Glycogen granules also contain enzymes that In the liver, G6P is also made available to other by covalent modification (phosphorylation and catalyse glycogen synthesis and breakdown, as tissues. However, because G6P cannot pass dephosphorylation). well as many proteins that regulate these through cell membranes, it must firstly be -Binds the cofactor pyridoxal-5- phosphate processes hydrolysed by glucose-6-phosphatase (G6Pase) (PLP), which is a vitamin B6 derivative prosthetic to glucose: group and is required for this enzyme’s activity -In animals, a constant supply of glucose is essential for tissues such as the brain and RBC. -The mobilisation of glucose from glycogen Glucose leaves the liver cell via the GLUT2 stores, primarily in the LIVER, provides a glucose transporter and is carried by the blood constant supply of glucose to ALL tissues. to other tissues. -Low levels of glycogen in muscle are degraded Muscle and other tissues lack G6Pase and by phosphorolytic cleavage and glycolysis to therefore retain their G6P provide ATP for local muscle contraction only. When glucose is plentiful, such as after a meal, glycogen synthesis accelerates. Glycogen structure A main backbone composed of glucose units linked by (1 → 4) glycosidic bonds. Branches connected to the backbone via (1 → 6) glycosidic bonds, occurring about once every Glycogen synthesis tenth glucose residue in the main chain 2.Glycogen Debranching Enzyme Synthesis of glycogen from G1P is -Glycogen phosphorylase continues to remove thermodynamically unfavourable under G1P units from a glycogen branch until it physiological conditions without energy input. approaches to within four or five residues of an Consequently, glycogen synthesis and (1 → 6) branch point, leaving a “limit branch”. breakdown must occur by separate pathways. -Glycogen debranching enzyme is a The three enzymes that participate in glycogen glycosyltransferase that transfers an (1 → 4)- synthesis are: linked trisaccharide unit from a limit branch to 1.UDP-glucose pyrophosphorylase -Glycogen is highly branched. the nonreducing end of another branch. 2.glycogen synthase -The branched structure means that a single 3.glycogen branching enzyme glycogen molecule can be expanded quickly by -This reaction forms a new (1 → 4) linkage adding glucose residues to its many ends and with three more units for glycogen 1.UDP-glucose pyrophosphorylase degraded quickly by simultaneously removing phosphorylase to act upon. In mammalian cells, glycogen synthesis requires glucose from the ends of many branches. -The (1 → 6) bond of the residue remaining at the free energy released by the nucleotide -Glycogen consists of a single “reducing” end the branch point is then hydrolysed by the uridine triphosphate (UTP), which is (glucose with a free hydroxyl group on C1) and debranching enzyme to yield free glucose energetically equivalent to ATP. many “nonreducing” ends (glucose with a free G1P is combined with UTP in a reaction hydroxyl group on C4) catalysed by UDPglucose pyrophosphorylase. The product of this reaction is uridine diphosphate glucose (UDP-glucose), which is an “activated” compound that can readily donate a glucosyl unit to the growing glycogen chain The main backbone of glycogen (made up of (1 → 4) glycosidic linkages) folds into a helical arrangement. 2.Glycogen Synthase Glycogen storage diseases GLUCONEOGENESIS Glycogen synthase transfers the glucose unit to -Inherited disorders that affect glycogen the non-reducing end of one of glycogen’s metabolism. Demand for glucose branches. -They result in the production of glycogen that is abnormal in either quality or quantity. -Those affecting the liver generally produce hepatomegaly (enlarged liver) and hypoglycaemia (low blood sugar). ~160g of glucose needed by an average adult -Those affecting muscles cause muscle cramps body daily. and weakness. 20g is found in bodily fluids and 190g is -Both types may also cause cardiovascular and available from glycogen. renal disturbances. → enough to last about a -At least ten different types of glycogen storage day. 3.Glycogen Branching Enzyme diseases have been characterised so far Most organs can use variety Glycogen synthase generates only (1 → 4) of carbon sources to linkages. generate energy, but there Glycogen branching is accomplished by a are some exceptions that separate branching enzyme. require glucose as their sole A branch is created by transferring a 7-residue or primary carbon source segment from the end of a chain to the C6-OH group of a glucose residue on the same or Gluconeogenesis another glycogen chain McArdle’s Disease -Gluconeogenesis is the synthesis of glucose Hereditary Glycogen Storage Disease Type V: from noncarbohydrate precursors. Muscle Glycogen Phosphorylase Deficiency -When dietary sources of glucose are not (McArdle’s Disease) available and when the liver has exhausted its supply of glycogen, glucose is synthesised via Symptoms include painful muscle cramps upon gluconeogenesis. exertion. These typically don’t appear until early -Gluconeogenesis is a UNIVERSAL pathway – adulthood and can be prevented by avoiding present in plants, animals, fungi and strenuous exercise. microorganisms This condition affects glycogen metabolism in muscle but not in liver, which contains normal Gluconeogenesis occurs primarily in the liver Glycogen breakdown vs synthesis amounts of a different phosphorylase and, to a lesser extent, in the kidneys. In the isoenzyme liver, the important precursors for glucose synthesis are: Pyruvate, Lactate and Glycerol Other non-carbohydrate precursors -Other non-carbohydrate precursors of gluconeogenesis include: TCA Cycle intermediates. Carbon skeletons of MOST amino acids. (Pyruvate) FIRSTLY, ALL of these precursors must be Control of glycogen metabolism converted to the 4C compound, oxaloacetate. Regulation of glycogen metabolism involves both allosteric control and hormonal control by covalent modification of regulatory enzymes Allosteric control Covalent modification -Covalent modification (in the form of The ONLY amino acids in animals that cannot be phosphorylation and dephosphorylation) is the converted to oxaloacetate and act as precursors primary mechanism for regulating glycogen are leucine and lysine because their breakdown synthase and glycogen phosphorylase. yields only acetyl-CoA. -Phosphorylation deactivates glycogen synthase Similarly, fatty acids cannot act as glucose and activates glycogen phosphorylase. precursors in animals because most are -Dephosphorylation activates glycogen synthase completely degraded to acetyl-CoA. and deactivates glycogen phosphorylase. -Since these two enzymes catalyse key reactions in opposing metabolic pathways, their reciprocal regulation is metabolically efficient The interconversions of the two enzymatic forms of glycogen synthase and glycogen phosphorylase are controlled by the hormones epinephrine and glucagon, which activate protein kinases Gluconeogenesis is sometimes called “Reverse Bypass II : Fructose-1,6-bisphosphate →F-6-P Fructose-2,6-bisphosphate is a potent allosteric Glycolysis” -Phosphofructokinase (PFK) is the major ACTIVATOR of phosphofructokinase (glycolysis) BUT, Three of the ten reactions in glycolysis are regulatory enzyme in glycolysis and catalyses and a potent INHIBITOR of fructose 1,6- thermodynamically irreversible. the irreversible phosphoryl transfer from ATP to bisphosphatase (gluconeogenesis). These three reactions are specifically bypassed fructose-6-phosphate. in gluconeogenesis while the remaining seven -Reversal of this reaction would require the This mode of regulation is efficient because a reactions can be used in reverse synthesis of ATP and insufficient energy is single compound can control flux through two present in fructose-1,6-bisphosphate to do so. opposing pathways in a reciprocal manner -In gluconeogenesis, this step is bypassed and the phosphoryl group is removed by hydrolysis catalysed by fructose 1,6-bisphosphatase Bypass III : Glucose-6-phosphate → glucose In the third and final gluconeogenesis bypass, a phosphoryl group is removed from glucose-6- -At different steps, different molecules exert phosphate. allosteric effects on the enzymes of the This hydrolytic reaction is catalysed by the pathway. enzyme glucose-6-phosphatase (breaks down -Products of the pathway or if they occur lower glycogen to glucose in the liver) down → usually have inhibitory effects. -Molecules that are at the starting point of the pathway → usually have activating effects. -High or low energy levels will also control the The Cori Cycle pathway (ATP, ADP/AMP) Bypass I : pyruvate → Phosphoenolpyruvate -A process that happen in corporation between (PEP) the skeletal muscles and the liver under low Part of bypass 1 takes place in the mitochondrial oxygen conditions. matrix (glycolysis & gluconeogenesis occur in Active skeletal muscle catabolises glucose via the cytoplasm ) glycolysis and lactate fermentation. In higher animals, initiation of glucose synthesis Lactate is then transported back to the liver typically begins with transport of pyruvate to where it is used in gluconeogenesis. the mitochondrial matrix. Glucose synthesised in the liver is transported Pyruvate carboxylation is catalysed by pyruvate via blood to muscle. carboxylase to form oxaloacetate. The essential cofactor of pyruvate carboxylase is biotin, which serves as a carrier of “activated CO2 ” Gluconeogenesis summary Since oxaloacetate cannot pass through mitochondrial membrane, it is first reduced to malate by malate dehydrogenase. It is then transported out to the cytoplasm where it is reoxidised to oxaloacetate and then Energy requirements decarboxylated to phosphoenolpyruvate (PEP) Producing 1 glucose from 2 pyruvate consumes by phosphoenolpyruvate carboxykinase. 6 ATP. If glycolysis and gluconeogenesis occurred simultaneously, there would be a net consumption of ATP To avoid this waste of metabolic free energy, gluconeogenic cells (mainly liver) carefully regulate the opposing pathways of glycolysis and gluconeogenesis according to the cell’ s energy needs Control of gluconeogenesis - Allosteric Control Requires 2 ATP - Hormonal Control - Transcriptional Control The major regulatory point is centered around the interconversion between fructose-6- phosphate and fructose-1,6-bisphosphate. Most naturally occurring fatty acids contain Glycolipids INTRO TO FATS even numbers of carbon atoms, typically Glycolipids are lipids that have sugars attached between 14 and 24. to them. Water insoluble molecules that are highly Found in the cell membranes and play a role in soluble in organic solvents. Unsaturated fatty acids have lower melting cell-cell interactions There are five main classes: points compared to saturated fatty acids of the – Free Fatty Acids same length. – Triacylglycerols – Phospholipids Chain length also affects the melting point – Glycolipids Longer chain length → higher melting point – Steroids Cis vs trans double bonds Lipids in foods Provide essential fatty acids. Unsaturated fatty acids normally have cis- Provide concentrated food energy. double bonds; produces kink in hydrocarbon Carry fat-soluble vitamins (A, D, E, K). chain. Provide a sense of fullness (satiety). Slow gastric emptying. Energy value: 37 kJ/g for fat vs. 16 kJ/g for carbohydrate/proteins Lipids in the body Chief form of stored energy in the body Double bond-caused kink in fatty acid increases (anhydrous). phospholipid mobility and makes the membrane Serve as emergency fuel in hard times. more fluid, so that protein machinery located Protect internal organs from shock. within membrane can work more efficiently Insulate against cold. Major component of cell membranes. Role in signal transduction pathways. Steroids Converted to other compounds on demand. Functions as powerful hormones such as estradiol and testosterone. Fatty acids Facilitate the digestion of lipids in the diet. Chains of hydrogen bearing carbons called Key part of the membranes. Trans-double bonds produced during processing hydrocarbons that terminate in a carboxylic They have a cyclical structure. of unsaturated fatty acids in foods acid group. (Hydrogenation → ‘hardening’ of an Hydrocarbon chains can be various lengths. All steroids have a four unsaturated oil e.g. in margarine production); May have one or more double bonds. fused ring structure called behaves very much like saturated fatty acids. Considered to be good fuels as they are more the steroid nucleus. All reduced than carbohydrates. Yields more ATP biochemically active steroids when undergoing catabolism to carbon dioxide are modified versions of this and water basic structure Cholesterol Triacylglycerol (triglyceride) -Is the most common steroid and belongs to a Storage form of fatty acids class of molecules called sterol-steroid. -Needed to maintain proper membrane fluidity. The simplest lipids constructed from fatty acids, -Is the major sterol in animal tissues also known as neutral fats. Saturated fatty acids (no double bonds)