Biochemistry Chapter 1 PDF
Document Details
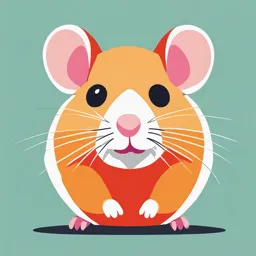
Uploaded by EasedWave
Tags
Summary
This chapter outline of biochemistry provides an introduction to fundamental themes in biochemistry and the chemical foundations of life. It explores the structure and function of biomolecules and the differences between prokaryotic and eukaryotic cells. The chapter also touches upon the relationship between biochemistry and other scientific disciplines, such as physics and chemistry.
Full Transcript
Chapter Outline 1-1 Basic Themes 1 1 Biochemistry and Life 1 Origin of Life on Earth 2 1-2 Chemical Foundations of Biochemistry 2 Bi...
Chapter Outline 1-1 Basic Themes 1 1 Biochemistry and Life 1 Origin of Life on Earth 2 1-2 Chemical Foundations of Biochemistry 2 Biochemistry and the Amino Acids 2 Carbohydrates 3 Nucleotides 4 Organization of Cells Lipids 4 Functional Groups Important in Biochemistry 4 1-3 The Beginnings of Biology 6 The Earth and Its Age 6 1-1 Basic Themes Biomolecules 8 Molecules to Cells 12 Biochemistry and Life 1-4 The Biggest Biological Distinction— c How does biochemistry describe life processes? Prokaryotes and Eukaryotes 16 Prokaryotic Cells 17 Living organisms, such as humans, and even the individual cells of Eukaryotic Cells 18 which they are composed, are enormously complex and diverse. Nevertheless, certain unifying features are common to all living 1-5 How We Classify Eukaryotes things from the simplest bacterium to the human being. They all and Prokaryotes 21 Five-Kingdom Classification System 22 use the same types of biomolecules, and they all use energy. As a result, 1A BIOCHEMICAL CONNECTIONS organisms can be studied via the methods of chemistry and physics. BIOTECHNOLOGY Extremophiles: The Toast Biochemistry can be defined in many ways. From the name, it is of the Industry 23 clear it is the chemistry of life. It combines biology and chemistry, Three-Domain Classification System 23 and any given instructor may have more of a biology focus, a chemis- Eukaryotic Origins 23 try focus, or anything in between. 1-6 Biochemical Energetics 25 Disciplines that appear to be unrelated to biochemistry can pro- Thermodynamic Principles 25 vide answers to important biochemical questions. For example, the Energy Changes 26 magnetic resonance imaging (MRI) tests that play an important role Spontaneity in Biochemical Reactions 26 in the health sciences originated with physicists, became a vital tool Life and Thermodynamics 27 for chemists, and currently play a large role in biochemical research. 1B BIOCHEMICAL CONNECTIONS The field of biochemistry draws on many disciplines, and its multidis- THERMODYNAMICS Predicting Reactions 28 ciplinary nature allows it to use results from many sciences to answer questions about the molecular nature of life processes. Important applica- tions of this kind of knowledge are made in medically related fields; an understanding of health and disease at the molecular level leads to more effective treatment of illnesses of many kinds. The activities within a cell are similar to the transportation sys- tem of a city. The cars, buses, and taxis correspond to the mole- cules involved in reactions (or series of reactions) within a cell. The routes traveled by vehicles likewise can be compared to the reac- tions that occur in the life of the cell. Note particularly that many vehicles travel more than one route—for instance, cars and taxis can go almost anywhere—whereas other, more specialized modes of transportation, such as subways and streetcars, are confined to single paths. Similarly, some molecules play multiple roles, whereas others take part only in specific series of reactions. Also, the routes operate simultaneously, and we shall see that this is true of the many reactions within a cell. To continue the comparison, the transportation system of a large city has more kinds of transportation than does a smaller 1 Copyright 2018 Cengage Learning. All Rights Reserved. May not be copied, scanned, or duplicated, in whole or in part. WCN 02-200-203 2 CHAPTER 1 Biochemistry and the Organization of Cells one. Although a small city may have only cars, buses, and taxis, a large city may have all of these plus others, such as streetcars or subways. Analogously, some reactions are found in all cells, and others are found only in specific kinds of cells. Also, more structural features are found in the larger, more complex cells of larger organisms than in the simpler cells of organisms such as bacteria. An inevitable consequence of this complexity is the large quantity of terminology that is needed to describe it; learning considerable new vocabu- lary is an essential part of the study of biochemistry. You will also see many cross-references in this book, which reflect the many connections among the processes that take place in the cell. Origin of Life on Earth The fundamental similarity of cells of all types makes speculating on the origins of life a worthwhile question. How did the components of our bodies come to be and to do the things that they do? What are the molecules of life? Even the structures of comparatively small biomolecules consist of several parts. Large biomolecules, such as proteins and nucleic acids, have complex structures, and living cells are enormously more complex. Even so, both molecules and cells must have arisen ultimately from very simple molecules, such as water, methane, carbon dioxide, ammonia, nitrogen, and hydrogen (Figure 1.1). In turn, these simple molecules must have arisen from atoms. c How did living things originate? The way in which the Universe itself, and the atoms of which it is com- posed, came to be is a topic of great interest to astrophysicists as well as other scientists. Simple molecules were formed by combining atoms, and reactions of simple molecules led in turn to more complex molecules. The molecules that play a role in living cells today are the same molecules as those encountered in organic chemistry; they simply operate in a different context. 1-2 Chemical Foundations of Biochemistry organic chemistry the study of compounds of Organic chemistry is the study of compounds of carbon and hydrogen and carbon, especially of carbon and hydrogen and their derivatives. Because the cellular apparatus of living organisms is made their derivatives up of carbon compounds, biomolecules are part of the subject matter of or- ganic chemistry. Additionally, many carbon compounds are not found in any organism, and many topics of importance to organic chemistry have little connection with living things. We are going to concentrate on the aspects of organic chemistry that we need in order to understand what goes on in living cells. The small molecules found in the cell can usually be lumped into four basic classes. We will see these over and over again during our study of biochemistry. They are the basic building blocks of life. Amino Acids The simplest compounds are the amino acids. They get their name from the fact that they all contain an amino group and a carboxyl group, as shown in Figure 1.2. Under physiological conditions both the carboxyl group and amino group are ionized (-COO2 and –NH3+, respectively). Amino acids can be shown in various ways, including a structural formula or a ball and stick formula. Amino acids have a basic structure where a central carbon atom is bonded to a carboxyl group, an amino group, a hydrogen, and a variable group, called Copyright 2018 Cengage Learning. All Rights Reserved. May not be copied, scanned, or duplicated, in whole or in part. WCN 02-200-203 1-2 Chemical Foundations of Biochemistry 3 Body system of organism Organ Atoms Oxygen and hydrogen Bone Tissue Molecules O H H Water Bone tissue Cell Macromolecules Nucleus Protein Plasma Golgi membrane Bone cell Nucleus Organelles Mitochondria Figure 1.1 Levels of structural organization in the human body. Note the hierarchy from simple to complex. COO2 COO– 1 H 3N C H + the R group. It is the difference between the R groups that makes each NH3 C amino acid unique. H CH3 Carbohydrates L-Alanine Carbohydrates are compounds made up of carbon, hydrogen, and oxy- CH3 gen, with a general formula of (CH2O)n, where n is at least 3. The sim- plest forms are called monosaccharides, or sugars. The most common Figure 1.2 Basic structure of the amino acid, alanine. Copyright 2018 Cengage Learning. All Rights Reserved. May not be copied, scanned, or duplicated, in whole or in part. WCN 02-200-203 4 CHAPTER 1 Biochemistry and the Organization of Cells H O monosaccharide is glucose, which has the formula C 6H 12O 6, as shown in 1 C Figure 1.3. For convenience, sugars are often drawn as a straight chain, but in 2 solution they form cyclic structures. Simple sugars often make up much larger H C OH polymers and are used for energy storage and structural components. 3 HO C H CH2OH H 4 C OH H C O H Nucleotides H 5 C OH H C Nucleotides are the basic unit of the hereditary materials DNA and RNA. They H C OH HO C C OH also form the molecular currency of the cell, adenosine triphosphate (ATP). A 6 CH2OH nucleotide is composed of a five-carbon sugar, a nitrogen-containing ring, and H OH one or more phosphate groups. The important nucleotide, ATP, is shown in D-Glucose -D-Glucopyranose Figure 1.4. It is composed of the nitrogenous base adenine, the sugar ribose, Figure 1.3 Straight chain and cyclic depictions and three phosphates. of glucose, the most common monosaccharide. Lipids NH2 The fourth major group of biochemicals consists of lipids. They are the most Phosphoric anhydride N N diverse and cannot be shown with a simple structure common to all lipids. linkages However, they all have the common trait that they are poorly soluble in water. N N This is because most of their structure is composed of long chains of hydrocar- O O O bons. A simple lipid is palmitic acid, which has 16 carbons. There are several –O P O P O P O CH2 ways to depict such a lipid, as shown in Figure 1.5. O Another important lipid you have heard of is cholesterol, shown in O– O– O– H H Figure 1.6. It differs considerably in its structure from palmitic acid, but is still H H very insoluble in water due to the chains of carbon and the fact that it has only OH OH a single oxygen molecule in it. ATP c Can a chemist make the molecules of life in a laboratory? (adenosine-5'-triphosphate) Until the early part of the 19th century, there was a widely held belief in “vital Figure 1.4 The structure of ATP, an important forces,” forces presumably unique to living things. This belief included the idea nucleotide in energy production. that the compounds found in living organisms could not be produced in the laboratory. German chemist Friedrich Wöhler performed the critical experi- O OH O OH ment that disproved this belief in 1828. Wöhler synthesized urea, a well-known C C waste product of animal metabolism, from ammonium cyanate, a compound CH2 obtained from mineral (i.e., nonliving) sources. H2C NH4OCN S H2NCONH2 CH2 H2C Ammonium Urea CH2 cyanate H2C It has subsequently been shown that any compound that occurs in a living CH2 organism can be synthesized in the laboratory, although in many cases the H2C synthesis represents a considerable challenge to even the most skilled organic chemist. CH2 Palmitic acid The reactions of biomolecules can be described by the methods of organic H2C chemistry, which requires the classification of compounds according to their CH2 functional groups. The reactions of molecules are based on the reactions of their respec- H2C tive functional groups. CH2 H2C Functional Groups Important in Biochemistry CH3 Table 1.1 lists some biologically important functional groups. Note that most Figure 1.5 The simple lipid palmitic acid, shown of these functional groups contain oxygen and nitrogen, which are among the with a structural formula, an abbreviated formula, most electronegative elements. As a result, many of these functional groups and a space-filling model. are polar, and their polar nature plays a crucial role in their reactivity. Some functional groups groups of atoms that give rise to groups that are vitally important to organic chemists are missing from the the characteristic reactions of organic compounds table because molecules containing these groups, such as alkyl halides and acyl Copyright 2018 Cengage Learning. All Rights Reserved. May not be copied, scanned, or duplicated, in whole or in part. WCN 02-200-203 1-2 Chemical Foundations of Biochemistry 5 chlorides, do not have any particular applicability in biochemistry. Conversely, CH3 carbon-containing derivatives of phosphoric acid are mentioned infrequently CHCH2CH2CH2CH(CH3)2 H 3C in beginning courses on organic chemistry, but esters and anhydrides of phos- H phoric acid (Figure 1.7) are of vital importance in biochemistry. ATP, a mol- H3C H ecule that is the energy currency of the cell, contains both ester and anhydride linkages involving phosphoric acid. H H HO Important classes of biomolecules have characteristic functional groups H that determine their reactions. We shall discuss the reactions of the functional Cholesterol groups when we consider the compounds in which they occur. Figure 1.6 The structure of cholesterol, an important lipid in biological membranes. Table 1.1 Functional Groups of Biochemical Importance Classof Class of General Characteristic Characteristic NameName of of Compound Compound General Structure Structure Functional Group Functional Group Functional Group Functional Group ExampleExample RCH CH2 RCH CHR Alkenes C C Double bond CH2 CH2 R2C CHR R2C CR2 Alcohols ROH OH Hydroxyl group CH3CH2OH Ethers ROR O Ether group CH3OCH3 RNH2 Amines R2NH N Amino group CH3NH2 R3N Thiols RSH SH Sulfhydryl group CH3SH O O O Aldehydes Carbonyl group R C H C CH3CH O O O Carbonyl group Ketones R C R C CH3CCH3 O O O Carboxylic Carboxyl group acids R C OH C OH CH3COH O O O Ester group Esters R C OR C OR CH3COCH3 O O O R C NR2 C N Amide group CH3CN(CH3)2 O Amides R C NHR O R C NH2 O O O Phosphoric acid Phosphoric ester R O P OH O P OH CH3 O P OH esters group OH OH OH O O O O O O Phosphoric acid Phosphoric R O P O P OH P O P HO P O P OH anhydrides anhydride group OH OH OH OH OH OH Copyright 2018 Cengage Learning. All Rights Reserved. May not be copied, scanned, or duplicated, in whole or in part. WCN 02-200-203 6 CHAPTER 1 Biochemistry and the Organization of Cells O O HO P OH + HO R HO P O R 1 Reaction of phosphoric acid with a hydroxyl group to form an ester, which OH H2O OH contains a P-O-R linkage. Phosphoric Phosphoric acid Alcohol An ester of phosphoric acid acid is shown in its nonionized form in this figure. Space-filling models of phosphoric acid and its methyl ester are shown. The red spheres represent oxygen; the white, hydrogen; the green, R carbon; and the orange, phosphorus. O O O O HO P OH + HO P OH HO P O P OH Reaction of two molecules of phosphoric OH OH H2O OH OH 2 acid to form an anhydride, which Anhydride of phosphoric acid contains a P-O-P linkage. A space-filling model of the anhydride of phosphoric acid is shown. NH2 Ester O O O C N C N HO P O P O P O HC C CH OH OH OH CH2 O N N 3 The structure of ATP (adenosine triphosphate), showing two anhydride C C linkages and one ester. Anhydride H H H C C H OH OH ATP Figure 1.7 ATP and the reactions for its formation. 1-3 The Beginnings of Biology The Earth and Its Age Although humans in general and science fiction writers in particular are fas- cinated by the idea of life on other planets, to date, we are aware of only one planet that unequivocally supports life: our own. The Earth and its waters are universally understood to be the source and mainstay of life as we know it. A natural first question is how the Earth, along with the Universe of which it is a part, came to be. c How and when did the Earth come to be? Currently, the most widely accepted cosmological theory for the origin of the Universe is the big bang, a cataclysmic explosion. According to big-bang cosmology, all the matter in the Universe was originally confined to a com- paratively small volume of space. As a result of a tremendous explosion, this “primordial fireball” started to expand with great force. Immediately after the big bang, the Universe was extremely hot, on the order of 15 billion Copyright 2018 Cengage Learning. All Rights Reserved. May not be copied, scanned, or duplicated, in whole or in part. WCN 02-200-203 1-3 The Beginnings of Biology 7 (15 3 109) K. (Note that Kelvin temperatures are written without a degree symbol.) The average temperature of the Universe has been decreasing ever since as a result of expansion, and the lower temperatures have permitted the formation of stars and planets. In its earliest stages, the Universe had a fairly simple composition. Hydrogen, helium, and some lithium (the three smallest and simplest elements on the periodic table) were present, having been formed in the original big-bang explosion. The rest of the chemical elements are thought to have been formed in three ways: (1) by thermonu- clear reactions that normally take place in stars, (2) in explosions of stars, and (3) by the action of cosmic rays outside the stars since the formation of the galaxy. The process by which the elements are formed in stars is a topic of interest to chemists as well as to astrophysicists. For our purposes, note that the most abundant isotopes of biologically important elements such as car- bon, oxygen, nitrogen, phosphorus, and sulfur have particularly stable nuclei. These elements were produced by nuclear reactions in first-generation stars, the original stars produced after the beginning of the Universe (Table 1.2). Many first-generation stars were destroyed by explosions called supernovas, and their stellar material was recycled to produce second-generation stars, such as our own Sun, along with our solar system. Radioactive dating, which uses the decay of unstable nuclei, indicates that the age of the Earth (and the rest of the solar system) is 4 billion to 5 billion (4 3 109 to 5 3 109) years. The atmosphere of the early Earth was very different from the one we live in, and it probably went through several stages before reaching its current composi- tion. The most important difference is that, according to most theories of the origins of the Earth, very little or no free oxygen (O2) existed in the early stages (Figure 1.8). The early Earth was constantly irradiated with ultraviolet light from the Sun because there was no ozone (O3) layer in the atmosphere to block it. Under these conditions, the chemical reactions that produced simple biomolecules took place. The gases usually postulated to have been present in the atmosphere of the early Earth include NH3, H2S, CO, CO2, CH4, N2, H2, and (in both liquid and vapor forms) H2O. However, there is less agreement on the rela- tive amounts of these components, from which biomolecules ultimately arose. Many of the earlier theories of the origin of life postulated CH 4 as Table 1.2 Abundance of Important Elements Relative to Carbon* Element Abundance in Organisms Abundance in Universe Hydrogen 80–250 10,000,000 Carbon 1,000 1,000 Nitrogen 60–300 1,600 Oxygen 500–800 5,000 Sodium 10–20 12 Magnesium 2–8 200 Phosphorus 8–50 3 Sulfur 4–20 80 Potassium 6–40 0.6 Calcium 25–50 10 Manganese 0.25–0.8 1.6 Iron 0.25–0.8 100 Zinc 0.1–0.4 0.12 *Each abundance is given as the number of atoms relative to a thousand atoms of carbon. Copyright 2018 Cengage Learning. All Rights Reserved. May not be copied, scanned, or duplicated, in whole or in part. WCN 02-200-203 8 CHAPTER 1 Biochemistry and the Organization of Cells Figure 1.8 Formation of biomolecules on the early Earth. Conditions on early Earth would have been inhospitable for most of today’s life. Very little or no oxygen (O2) existed. Volcanoes erupted, spewing gases, and violent thunderstorms produced torrential rainfall that covered the Earth. The green arrow indicates the formation of biomolecules from simple precursors. the carbon source, but more recent studies have shown that appreciable amounts of CO 2 must have existed in the atmosphere at least 3.8 billion (3.8 3 109) years ago. This conclusion is based on geological evidence: The earliest known rocks are 3.8 billion years old, and they are carbonates, which arise from CO2. Any NH3 originally present must have dissolved in the oceans, leaving N 2 in the atmosphere as the nitrogen source required for the formation of proteins and nucleic acids. Biomolecules c How were biomolecules likely to have formed on the early Earth? Experiments have been performed in which the simple compounds of the early atmosphere were allowed to react under the varied sets of conditions that might have been present on the early Earth. The results of such experi- ments indicate that these simple compounds react abiotically or, as the word indicates (a, “not,” and bios, “life”), in the absence of life, to give rise to bi- ologically important compounds such as the components of proteins and nucleic acids. Of historic interest is the well-known Miller–Urey experiment. In each trial, an electric discharge, simulating lightning, is passed through a closed system that contains H 2, CH4, and NH3, in addition to H2O. Simple organic molecules, such as formaldehyde (HCHO) and hydrogen cyanide (HCN), are typical products of such reactions, as are amino acids, the build- ing blocks of proteins. According to one theory, reactions such as these took place in the Earth’s early oceans; other researchers postulate that such reac- tions occurred on the surfaces of clay particles that were present on the early Earth. It is certainly true that mineral substances similar to clay can serve as catalysts in many types of reactions. Recent theories of the origin of life focus Copyright 2018 Cengage Learning. All Rights Reserved. May not be copied, scanned, or duplicated, in whole or in part. WCN 02-200-203 1-3 The Beginnings of Biology 9 on RNA, not proteins, as the first genetic molecules. Proteins are thought to have developed later in the evolution of the earliest cells. This point does not diminish the importance of this first experiment on abiotic synthesis of biomolecules. Recent experiments have shown it is possible to synthesize nucleotides from simple molecules by a pathway that includes a precursor that is neither a sugar nor a nucleobase, but a fragment consisting of a sugar and a part of a base. This fragment, 2-aminooxazole, is highly volatile and can vapor- ize and condense so as to give rise to pockets of pure material in reasonably large amounts. In turn, phosphates released by volcanic action can react with the 2-aminooxazole to produce nucleotides (Figure 1.9). The products in- clude nucleotides that are not part of present-day RNA, but intense ultravio- let light, which was present on the early Earth, destroyed those nucleotides, leaving those found in RNA today. Living cells today are assemblages that include very large molecules, such as proteins, nucleic acids, and polysaccharides. These molecules are larger by FAILED NUCLEOTIDES A NEW ROUTE Chemists have long been unable to find a In the presence of phosphate, the raw materials route by which nucleobases, phosphate and ribose (the for nucleobases and ribose first form 2-amino- sugar component of RNA) would naturally combine to oxazole, a molecule that contains part of a sugar generate quantities of RNA nucleotides. and part of a C or U nucleobase. Further reac- tions yield a full ribose-base block and then a full nucleotide. The reactions also produce “wrong” combinations of the original Chemicals present before first living cells molecules, but after exposure to ultraviolet rays, only the “right” versions—the nucleotides—survive. Chemicals present before first living cells Sugar Nucleobase C 2-aminooxazole Phosphate Phosphate Arabino- oxazoline Sugar C Oxygen Carbon Nitrogen Phosphate Phosphorus RNA NUCLEOTIDE Figure 1.9 Abiotic synthesis of nucleotides. The volatile compound 2-aminooxazole is a key intermediate that eventually gives rise to nucleotides. (Copyright © Andrew Swift) Copyright 2018 Cengage Learning. All Rights Reserved. May not be copied, scanned, or duplicated, in whole or in part. WCN 02-200-203 10 CHAPTER 1 Biochemistry and the Organization of Cells many powers of ten than the smaller molecules from which they are built. monomers small molecules that may bond to Hundreds or thousands of these smaller molecules, or monomers, can be many others to form a polymer linked to produce macromolecules, which are also called polymers. The polymers macromolecules formed by the bonding versatility of carbon is important here. Carbon is tetravalent and able to form of smaller units bonds with itself and with many other elements, giving rise to different kinds of monomers, such as amino acids, nucleotides, and monosaccharides (sugar monomers). Proteins and nucleic acids play a key role in life processes. In present-day cells, proteins macromolecules formed by the amino acids (the monomers) combine by polymerization to form proteins, polymerization of amino acids nucleotides (also monomers) combine to form nucleic acids, and the po- nucleic acids macromolecules formed by the lymerization of sugar monomers produces polysaccharides. Polymerization polymerization of nucleotides experiments with amino acids carried out under early-Earth conditions have produced proteinlike polymers. Similar experiments have been done on the abiotic polymerization of nucleotides and sugars, which tends to happen less readily than the polymerization of amino acids. Much of this discussion is spec- ulative, but it is a useful way to start thinking about biomolecules. The several types of amino acids and nucleotides can easily be distinguished from one another. When amino acids form polymers, with the loss of water accompanying this spontaneous process, the sequence of amino acids deter- mines the properties of the protein formed. Likewise, the genetic code lies in the sequence of monomeric nucleotides that polymerize to form nucleic acids, the molecules of heredity (Figure 1.10). In polysaccharides, however, the order of monomers rarely has an important effect on the properties of the polymer, nor does the order of the monomers carry any genetic information. (Other aspects of the linkage between monomers are important in polysaccharides, as we shall see when we discuss carbohydrates in Chapter 16.) Note that all the building blocks have a “head” and a “tail,” giving a sense of direction even at the monomer level (Figure 1.11). The effect of monomer sequence on the properties of polymers can be illus- catalytic activity the ability to increase the rate of trated by another example. Proteins of the class called enzymes display catalytic a chemical reaction activity, which means that they increase the rates of chemical reactions com- pared with uncatalyzed reactions. In the context of the origin of life, catalytic molecules can facilitate the production of large numbers of complex molecules, allowing for the accumulation of such molecules. When a large group of related molecules accumulates, a complex system arises with some of the characteris- tics of living organisms. Such a system has a nonrandom organization, tends to reproduce itself, and competes with other systems for the simple organic mol- ecules present in the environment. One of the most important functions of catalysis the process of increasing the rate of proteins is catalysis, and the catalytic effectiveness of a given enzyme depends chemical reactions on its amino acid sequence. The specific sequence of the amino acids present ultimately determines the properties of all types of proteins, including enzymes. A strand of DNA T T C A G C A A T A A G G G T C C T A C G G A G 5' 3' A polypeptide segment Figure 1.10 Informational macromolecules. Phe Ser Asn Lys Gly Pro Thr Glu Biological macromolecules are informational. The sequence of monomeric units in a biological polymer has the potential to contain information A polysaccharide chain if the order of units is not overly repetitive. Nucleic acids and proteins are informational Glc Glc Glc Glc Glc Glc Glc Glc Glc macromolecules; polysaccharides are not. Copyright 2018 Cengage Learning. All Rights Reserved. May not be copied, scanned, or duplicated, in whole or in part. WCN 02-200-203 1-3 The Beginnings of Biology 11 Amino acid Amino acid Polypeptide H R1 H R2 H R1 H C + C C N COO– H+3N COO – H+3N COO – H+3N C C...... H2O O H R2 N C Sense A Amino acids build proteins by connecting the carboxyl group of one amino acid with the amino group of the next amino acid. Sugar Sugar Polysaccharide H H H HO 4 6 HO 4 6 5 CH2OH 5 CH2OH HO................ CH2OH H H O + H O H H O HO HO H 3 3 HO H H HO 2 OH H HO 2 OH H HO 1 1 H 2O O 4..... 1 CH2OH H H H H O HO 4 1 OH HO H Sense H HO OH B Polysaccharides are built by linking the first carbon of one sugar H with the fourth carbon of the next sugar. Nucleotide Nucleotide Nucleic acid NH2 NH2 NH2 N N N N O O O 5' N O 5' N N 5' N O HO P OCH2 + HO P OCH2 HO P OCH2 O O O O– O– O– H H H H NH2........... 4' 1' 4' 1' H2O H H H H H H H H 3' 2' 3' 2' 3' 2' N N OH OH OH OH O OH.... N N 5' PO4 3' OH O P OCH2 Sense O O– H H C In nucleic acids the 3'-OH of the ribose ring of one nucleotide H H 3' forms a bond to the 5'-OH of the ribose ring of a neighboring OH OH nucleotide. All these polymerization reactions are accompanied by the elimination of water. Figure 1.11 Directionality in macromolecules. Biological macromolecules and their building blocks have a “sense” or directionality. If not for protein catalysis, the chemical reactions that take place in our bodies would be so slow as to be useless for life processes. We are going to have a lot to say about this point in Chapters 6 and 7. In present-day cells, the sequence of amino acids in proteins is determined by the sequence of nucleotides in nucleic acids. The process by which genetic information is translated into the amino acid sequence is very complex. DNA (deoxyribonucleic acid), one of the nucleic acids, serves as the coding material. The genetic code is the relationship between the nucleotide sequence in nucleic genetic code the information for the structure and acids and the amino acid sequence in proteins. As a result of this relationship, function of all living organisms Copyright 2018 Cengage Learning. All Rights Reserved. May not be copied, scanned, or duplicated, in whole or in part. WCN 02-200-203 12 CHAPTER 1 Biochemistry and the Organization of Cells Polynucleotide template the information for the structure and function of all living things is passed from G G A C U C one generation to the next. The workings of the genetic code are no longer completely mysterious, but they are far from completely understood. Theories G on the origins of life consider how a coding system might have developed, and U G A C C new insights in this area could shine some light on the present-day genetic code. Complementary polynucleotides Synthesis of a Molecules to Cells complementary H2O polynucleotide c Which came first—the catalysts or the hereditary molecules? Until recently, our understanding of biochemistry led to a “chicken vs. the egg” conundrum when we tried to figure out how life evolved. If RNA and DNA are G G A C U C genetic materials that convey the information of heredity and proteins are the molecules that act as catalysts for biochemical reactions, then how did life ever C C U G A G start? Which molecule came first, and how did the other develop? A discovery with profound implications for discussions of the origin of life Strands separate is that RNA (ribonucleic acid), another nucleic acid, is capable of catalyzing its The complementary own processing. Until this discovery, catalytic activity was associated exclusively strand acts as a new template strand with proteins. RNA, rather than DNA, is now considered by many scientists to have been the original coding material, and it still serves this function in some viruses. The idea that catalysis and coding both occur in one molecule has pro- C C U G A G vided a point of departure for more research on the origins of life. The “RNA world” is the current conventional wisdom, but many unanswered questions U C exist regarding this point of view. A C G G According to the RNA-world theory, the appearance of a form of RNA capable of coding for its own replication was the pivotal point in the origin of life. Polynu- Synthesis of new copies of the cleotides can direct the formation of molecules whose sequence is an exact copy H2O original strand of the original. This process depends on a template mechanism (Figure 1.12), which is highly effective in producing exact copies but is a relatively slow process. A catalyst is required, which can be a polynucleotide, even the original molecule C C U G A G itself. Polypeptides, however, are more efficient catalysts than polynucleotides, but there is still the question of whether they can direct the formation of exact G G A C U C copies of themselves. Recall that in present-day cells, the genetic code is based on nucleic acids, and catalysis relies primarily on proteins. How did nucleic acid Figure 1.12 The role of templates in synthesis of synthesis (which requires many protein enzymes) and protein synthesis (which polynucleotides. Polynucleotides use a template requires the genetic code to specify the order of amino acids) come to be? mechanism to produce exact copies of themselves: According to this hypothesis, RNA (or a system of related kinds of RNA) origi- G pairs with C, and A pairs with U by a relatively weak nally played both roles, catalyzing and encoding its own replication. Eventually, interaction. The original strand acts as a template to direct the synthesis of a complementary strand. The the system evolved to the point of being able to encode the synthesis of more complementary strand then acts as a template for the effective catalysts, namely proteins (Figure 1.13). Even later, DNA took over as production of copies of the original strand. Note that the primary genetic material, relegating the more versatile RNA to an intermedi- the original strand can be a template for a number ary role in directing the synthesis of proteins under the direction of the genetic of complementary strands, each of which in turn can code residing in DNA. A certain amount of controversy surrounds this theory, produce a number of copies of the original strand. but it has attracted considerable attention. This process gives rise to a many-fold amplification Another key point in the development of living cells is the formation of the original sequence. (Copyright © 1994 from of membranes that separate cells from their environment. The clustering of The Molecular Biology of the Cell, 3rd Edition, by coding and catalytic molecules in a separate compartment brings molecules A. Alberts, D. Bray, J. Lewis, M. Raff, K. Roberts, and into closer contact with each other and excludes extraneous material. For J. D. Watson.) reasons we shall explore in detail in Chapters 2 and 8, lipids are perfectly suited to form cell membranes (Figure 1.14). Recently, attempts have been made to combine several lines of reason- ing about the origin of life into a double-origin theory. According to this line of thought, the development of catalysis and the development of a coding system came about separately, and the combination of the two produced life as we know it. The rise of aggregates of molecules capable of catalyzing reactions was one origin of life, and the rise of a nucleic acid–based coding system was another origin. Copyright 2018 Cengage Learning. All Rights Reserved. May not be copied, scanned, or duplicated, in whole or in part. WCN 02-200-203 1-3 The Beginnings of Biology 13 1 3 Catalyst Replication The RNA sequence becomes a template for the sequence of amino acids in the protein by using A catalytic RNA directs its own the adaptor mechanism. replication with the original nucleotide sequence and shape. 2 Coding RNA Adaptor RNA Growing protein More catalytic RNAs evolve. Some One RNA molecule in a group (adaptor RNAs) bind to amino acids. catalyzes the synthesis of all The adaptor RNAs also engage in RNAs in the group. complementary pairing with coding RNA. Figure 1.13 Stages in the evolution of a system of self-replicating RNA molecules. At each stage, more complexity appears in the group of RNAs, leading eventually to the synthesis of proteins as more effective catalysts. (Copyright © 1994 from The Molecular Biology of the Cell, 3rd Edition, by A. Alberts, D. Bray, J. Lewis, M. Raff, K. Roberts, and J. D. Watson.) Without compartments With compartmentalization by cell membrane en co de s Protein catalyzes reactions for all RNA The protein made by the cell’s RNA is retained for use in the Self-replicating RNA cell. The RNA can be selected molecules, one of which on the basis of its use of a more can direct protein synthesis effective catalyst. Figure 1.14 The vital importance of a cell membrane in the origin of life. Without compartments, groups of RNA molecules must compete with others in their environment for the proteins they synthesize. With compartments, the RNAs have exclusive access to the more effective catalysts and are closer to each other, making it easier for reactions to take place. (Copyright © 1994 from The Molecular Biology of the Cell, 3rd Edition, by A. Alberts, D. Bray, J. Lewis, M. Raff, K. Roberts, and J. D. Watson. ) Copyright 2018 Cengage Learning. All Rights Reserved. May not be copied, scanned, or duplicated, in whole or in part. WCN 02-200-203 14 CHAPTER 1 Biochemistry and the Organization of Cells 4 Membrane incorporates new 5 Protocell fatty molecules divides, and and grows daughter cells D repeat the cycle co irec nv ti ec ontion of Daughter cells Fatty molecules 1 Nucleotides Nucleotides enter and form comple- mentary 3 Heat separates strand the strands RNA double strand 2 Protocell reaches “maturity” Figure 1.15 Hypothetical beginning of replication. Simple strings of nucleotides could have formed, perhaps initially lined up on the surface of clay particles. (1) On the cold side of the pond, RNA strands become surrounded by simple membranes. Nucleotides enter and form complementary strands by base pairing. (2) Over time, these protocells gain more molecules and complexity. (3) Cells find their way to the warm side of the pond, where the heat allows the RNA molecules to separate. (4) The protocell grows and gains more components. (5) Finally, the cell divides, produces daughter cells, and the process repeats. (Based on Scientific American, a division of Nature America, Inc.) A theory that life began on clay particles is a form of the double-origin theory. According to this point of view, coding arose first, but the coding material was the surface of naturally occurring clay. The pattern of ions on the clay surface is thought to have served as the code, and the process of crystal growth is thought to have been responsible for replication. Nucleotides, then RNA molecules, formed on the clay surface. The RNA molecules thus formed were released from the clay surface and enclosed in lipid sacs, forming protocells. In this scenario, protocells exist in a pond with a warm side and a cold side. Double-stranded polynucleo- tides are formed on the cold side of the pond on a single-stranded template (Figure 1.15). The protocell moves to the warm side of the pond, where the strands separate. The membrane incorporates more lipid molecules. The protocell di- vides, with a single-stranded RNA in each daughter cell, and the cycle repeats. In the development from protocells to single cells similar to modern bac- teria, proteins and then DNA enter the picture. In this scenario, ribozymes (catalytic RNA molecules) develop and direct the duplication of RNA. Other ribozymes catalyze metabolic reactions, eventually giving rise to proteins (Figure 1.16). Eventually, proteins rather than ribozymes catalyze most of the reactions in the cell. Still later, other enzymes catalyze the production of DNA, which takes over the primary role in coding. RNA now serves as an intermedi- ary between DNA and proteins. This scenario assumes that time is not a limit- ing factor in the process. In an attempt to study the origins of life, scientists Copyright 2018 Cengage Learning. All Rights Reserved. May not be copied, scanned, or duplicated, in whole or in part. WCN 02-200-203 1-3 The Beginnings of Biology 15 2 RNA CATALYSTS Ribozymes—folded RNA mole- cules analogous to protein-based enzymes—arise and take on such Waste jobs as speeding up reproduction Energy Double- Lipid and strengthening the protocell’s stranded membrane membrane. Consequently, RNA protocells begin to reproduce on their own. Ribozyme Nu trie nts RNA is duplicated New 1 EVOLUTION STARTS strand 3 METABOLISM BEGINS The first protocell is just a Ribozyme Other ribozymes catalyze sac of water and RNA and metabolism—chains of chemical requires an external stimulus reactions that enable protocells (such as cycles of heat and to tap into nutrients from the cold) to reproduce. But it environment. will soon acquire new traits. 5 PROTEINS TAKE OVER Amino Proteins take on a wide range of acid chain tasks within the cell. Protein- DNA based catalysts, or enzymes, gradually replace most Ribosome ribozymes. Ribozymes Enzymes Folded protein Folded Waste Energy protein 4 PROTEINS APPEAR N 6 THE BIRTH OF DNA Complex systems of RNA Enzyme Other enzymes begin to make 7 BACTERIAL WORLD ut catalysts begin to translate rie DNA. Thanks to its superior Organisms resembling modern strings of RNA letters nts stability, DNA takes on the role bacteria adapt to living virtually (genes) into chains of amino of primary genetic molecule. everywhere on earth and rule acids (proteins). Proteins RNA’s main role is now to act unopposed for billions of years, later prove to be more as a bridge between DNA and until some of them begin to evolve efficient catalysts and able proteins. into more complex organisms. to carry out a variety of tasks. Figure 1.16 Hypothetical evolution of simple protocells to more complex cells. (1) Protocells were just a simple sac containing simple RNA molecules. They could not even replicate without cycling between cold and warm temperatures. (2) In time the cells started replicating on their own using ribozymes. (3) With increasing complexity of RNA molecules, ribozymes begin to catalyze metabolic pathways. (4) As metabolism grows in complexity, RNA begins to be translated into proteins. Proteins prove to be more efficient catalysts. (5) Proteins gradually take over metabolism, replacing most of the functions of ribozymes. (6) New enzymes start producing DNA, which due to its superior stability, replaces RNA as the primary heredity material. (7) Organisms resembling bacteria evolve all over the Earth and rule for a billion years before evolution works to create more complex organisms. (Based on Scientific American, a division of Nature America, Inc.) have also attempted to combine the best properties of proteins and nucleic ac- ids and have created peptide nucleic acids, PNA. Evidence shows that the build- ing blocks of these hybrids could also have formed in the primordial world, and some theorize that PNA may have been the original molecule that allowed life to form. Currently scientists are attempting to create artificial living cells Copyright 2018 Cengage Learning. All Rights Reserved. May not be copied, scanned, or duplicated, in whole or in part. WCN 02-200-203 16 CHAPTER 1 Biochemistry and the Organization of Cells based on PNA. The goal is to demonstrate that under the conditions of the “primordial soup,” simple molecules could form complex molecules possess- ing the critical functions of catalysis and replication and that these could then form cells capable of dividing. At this writing, none of the theories of the origin of life is definitely established, and none is definitely disproved. The topic is still under active investigation. It seems highly unlikely that we will ever know with certainty how life originated on this planet, but these conjectures allow us to ask some of the important questions, such as those about catalysis and coding, that we are going to see many times in this text. 1-4 The Biggest Biological Distinction— Prokaryotes and Eukaryotes genome the total DNA of the cell All cells contain DNA. The total DNA of a cell is called the genome. Individual units of heredity, controlling individual traits by coding for a functional pro- genes individual units of inheritance tein or RNA, are genes. The earliest cells that evolved must have been very simple, having the mini- mum apparatus necessary for life processes. The types of organisms living to- prokaryotes microorganisms that lack a distinct day that probably most resemble the earliest cells are the prokaryotes. This nucleus and membrane-enclosed organelles word, of Greek derivation (karyon, “kernel, nut”), literally means “before the nucleus.” Prokaryotes include bacteria and cyanobacteria. (Cyanobacteria were formerly called blue-green algae; as the newer name indicates, they are more closely related to bacteria.) Prokaryotes are single-celled organisms, but groups of them can exist in association, forming colonies with some differentiation of cellular functions. c What is the difference between a prokaryote and a eukaryote? eukaryotes organisms whose cells have a The word eukaryote means “true nucleus.” Eukaryotes are more complex or- well-defined nucleus and membrane-enclosed ganisms and can be multicellular or single celled. A well-defined nucleus, set organelles off from the rest of the cell by a membrane, is one of the chief features distin- guishing a eukaryote from a prokaryote. A growing body of fossil evidence in- dicates that eukaryotes evolved from prokaryotes about 1.5 billion (1.5 × 109) years ago, about 2 billion years after life first appeared on the Earth. Examples of single-celled eukaryotes include yeasts and Paramecium (an organism fre- quently discussed in beginning biology courses); all multicellular organisms (e.g., animals and plants) are eukaryotes. As might be expected, eukaryotic cells are more complex and usually much larger than prokaryotic cells. The diameter of a typical prokaryotic cell is on the order of 1 to 3 µm (1 × 10–6 to 3 × 10–6 m), whereas that of a typical eukaryotic cell is about 10 to 100 µm. The distinction between prokaryotes and eukaryotes is so basic that it is now a key point in the classification of living organisms; it is far more important than the distinction between plants and animals. The main difference between prokaryotic and eukaryotic cells is the existence organelle a membrane-enclosed portion of a cell of organelles, especially the nucleus, in eukaryotes. An organelle is a part of the with a specific function cell that has a distinct function; it is surrounded by its own membrane within the cell. In contrast, the structure of a prokaryotic cell is relatively simple, lacking membrane-enclosed organelles. Like a eukaryotic cell, however, a prokaryotic