Enzyme Classification - Biochemistry PDF
Document Details
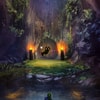
Uploaded by iiScholar
Arizona State University
Tags
Summary
This document is an introduction to Enzyme Classification, specifically focusing on different classes of enzymes. It highlights the key concepts of oxidoreductases and the catalytic mechanisms associated with them.
Full Transcript
Enzyme ClassificationIntroductionLesson 4.1 discusses many of the catalytic properties of enzymes. The next lessons delve deeper into enzymatic action in living systems and explore enzyme-catalyzed reactions, measurements, and optimization of enzyme activity.This lesson covers different classes of e...
Enzyme ClassificationIntroductionLesson 4.1 discusses many of the catalytic properties of enzymes. The next lessons delve deeper into enzymatic action in living systems and explore enzyme-catalyzed reactions, measurements, and optimization of enzyme activity.This lesson covers different classes of enzymes and the reactions they catalyze.4.2.01 Overview of Enzyme ClassificationEnzymes catalyze a range of biochemical reactions. These reactions act on a large variety of substrates to produce a similarly large variety of products. However, the types of reactions catalyzed are much less varied, and enzymes can be grouped by the type of reaction they catalyze.Early attempts to classify enzymes involved naming an enzyme based on its reaction and adding the suffix --ase (Figure 4.10). For example, enzymes with names that end in kinase catalyze reactions in which a phosphate group is transferred from a (eg, ATP) to a substrate. In contrast, enzymes with names that end in phosphatase catalyze reactions in which a phosphate group is removed from a substrate by addition of water.Similar rationale was used to name and group other enzymes; however, this system has limitations that become apparent when classifying larger groups. For example, if kinases add phosphate groups to a substrate while phosphatases break apart an already-phosphorylated substrate, how should phosphorylases---which break apart substrates by adding a phosphate group---be classified? Chapter 4: Enzyme Activity140Figure 4.10 Classes of enzymes that catalyze reactions involving phosphate.In 1961, the Enzyme Commission classified all enzymes into six major classes based on the catalytic mechanism of their major reaction. In 2018, a seventh class was added to this list (Table 4.1). Chapter 4: Enzyme Activity141Table 4.1 The seven major classes of enzymes.An enzyme\'s major classification is determined by the catalytic mechanism of its principal reaction; consequently, many enzymes have been reclassified over time as details about their mechanism have been elucidated through scientific research. Details about the mechanisms that define each major grouping are given in the following concepts of this lesson.4.2.02 OxidoreductasesThe first major class of enzymes is the oxidoreductase class. Members of this class catalyze oxidation-reduction, or , reactions, which transfer electrons from one molecule to another (see General Chemistry Lesson 9.1 and Organic Chemistry Lesson 5.7).Because redox reactions require a substrate to donate electrons and a substrate to receive electrons, all oxidoreductases are multisubstrate enzymes. Figure 4.11 shows a typical oxidoreductase-catalyzed reaction, in which a reducing agent and an oxidizing agent (the substrates) react and are converted to oxidized and reduced products, respectively. Several enzyme-catalyzed reactions deviate slightly from this model (eg, by reacting three substrates or releasing three products). Chapter 4: Enzyme Activity142Figure 4.11 A typical reaction catalyzed by an oxidoreductase enzyme. Electrons are transferred from the reducing agent to the oxidizing agent.In a typical oxidoreductase-catalyzed reaction, one reactant is converted into a product that either continues down other metabolic pathways or itself becomes the final product of a pathway. In other words, the original substrate is not regenerated. This reactant is typically referred to as the true substrate of the oxidoreductase enzyme.The second reactant is changed by the reaction, so it is also technically a substrate. However, this substrate typically cycles between various oxidoreductase enzymes, changing from its oxidized to its reduced state and back, which regenerates it. As such, it is often referred to as a redox cofactor (if inorganic) or a redox coenzyme (if organic). Figure 4.12 shows how a single redox cofactor can cycle between enzymes to assist various oxidoreductase reactions.Figure 4.12 Nicotinamide adenine dinucleotide (NAD+/NADH) cycles between its oxidized and reduced forms within a cell. Chapter 4: Enzyme Activity143Recognizing Oxidoreductase Enzymes and Their ReactionsEnzymes in the oxidoreductase class can be recognized both by the reactions they catalyze and by the name of the enzyme. As discussed previously, oxidoreductase-catalyzed reactions often involve a redox cofactor or coenzyme that changes oxidation state. For example, any reaction that interconverts NAD+ with NADH, FAD with FADH2, or ubiquinone with ubiquinol can be confidently assigned as a redox reaction catalyzed by an oxidoreductase. Some common redox factors are given in Table 4.2.Table 4.2 Common redox cofactors and coenzymes used by oxidoreductase enzymes.Importantly, some reactions involve these molecules but are not redox reactions. For example, NAD+ (nicotinamide adenine dinucleotide) can be used as a source of ADP-ribose to modify a target protein by ADP-ribosylation. In this case, NAD+ is converted into free nicotinamide and an ADP-ribosyl group, rather than into NADH, and so the enzyme that catalyzes this transfer is an oxidoreductase. Reactions with redox coenzymes are only considered oxidoreductase-catalyzed reactions if the coenzyme changes oxidation state.Aside from the involvement of a redox cofactor, oxidoreductase-catalyzed reactions can be identified by the oxidation or reduction of the substrate. An alcohol, for example, is oxidized to a carbonyl (ie, a ketone or an aldehyde), and aldehydes are oxidized to carboxylic acids or their derivatives (eg, esters, thioesters). Conversely, carboxylic acids (and their derivatives) are reduced to aldehydes, and carbonyl carbons are reduced to alcohols. Other common substrate oxidations or reactions are given in Table 4.3. Chapter 4: Enzyme Activity144Table 4.3 Common examples of substrate changes catalyzed by oxidoreductase enzymes.The name of an enzyme can also provide an important clue about an enzyme\'s classification. Any enzyme that includes the word oxidoreductase very likely falls into that class, but typically the name will focus on one direction (ie, either oxidation or reduction) and include terms such as oxidase (eg, cytochrome P450 oxidase), oxygenase (eg, cyclooxygenase), or reductase (eg, ribonucleotide reductase).Additionally, oxidoreductases tested on the exam often contain the term dehydrogenase. As shown in Table 4.2 and Table 4.3, the gain or loss of electrons is commonly accompanied by the gain or loss of hydrogen nuclei. Therefore, dehydrogenation reactions (ie, loss of hydrogen nuclei and electrons) are oxidation reactions. Conversely, reduction reactions that result in the gain of both electrons and hydrogen nuclei are hydrogenation reactions. However, because enzymes catalyze reactions in both directions (see Concept 4.1.04), many enzymes that catalyze reduction of the substrate (ie, hydrogenation) are still referred to as dehydrogenases. Chapter 4: Enzyme Activity145Concept Check 4.5Identify the redox coenzyme (if any) in each of the following reactions and whether it becomes oxidized or reduced during the reaction.4.2.03 TransferasesEnzymes within the transferase class catalyze the transfer of a from one molecule to another molecule (Figure 4.13).Figure 4.13 Schematic of transferase activity.Transferases require two substrates (ie, they are bisubstrate enzymes) and release two products. The substrate that loses a functional group during the reaction is often called the functional group donor. The substrate that receives the functional group is the acceptor (Table 4.4).Depending on the enzyme, the functional group being transferred can vary greatly in size. Methyltransferases, for example, transfer small --CH3 substituents whereas acyltransferases or aminoacyltransferases transfer much larger fatty acids or amino acids, respectively. Some enzymes can even transfer entire macromolecules such as proteins or polysaccharides. Chapter 4: Enzyme Activity146Table 4.4 Examples of reactions catalyzed by transferases.Given the large variation in functional group size, it is not uncommon for the functional group being transferred to be larger than the molecules it is being transferred to or from. This can complicate recognition of a transferase-catalyzed reaction. The following section provides advice on recognizing transferase enzymes and the reactions they catalyze.Recognizing Transferase Enzymes and Their ReactionsTransferases can be recognized both by the reactions they catalyze and by the name of the enzyme. As previously discussed, transferase reactions typically consume two substrates (one donor and one acceptor) and produce two products. The reaction involves the transfer of a functional group from the donor to the acceptor. One important exception is that water cannot be the acceptor. Transferase-like reactions that would involve water as an acceptor are instead classified as hydrolysis reactions and are catalyzed by hydrolases (Concept 4.2.04).Many transferases can be identified by their names. For example, acyltransferases are transferases that move fatty acyl groups from one molecule to another. Glycosyltransferases transfer carbohydrates by shifting a from one molecule to another.Some transferases do not include the term transferase in their name but instead have some other name specifying the subclass of transferase they belong to. Several of these subclasses are given in the next section. Knowing certain subclass names is helpful for identifying transferases. However, the specific enzymatic reactions described in the following section are only a means of demonstrating functional group transfer and do not need to be memorized.Special Classes of TransferasesKinases are transferases that transfer the of ATP or to a substrate molecule (the acceptor). The result is ADP and a phosphorylated molecule (Figure 4.14). Note that some kinases facilitate the reverse reaction (ie, phosphorylation of ADP using a Chapter 4: Enzyme Activity147phosphosubstrate as a donor) under physiological conditions (eg, phosphoglycerate kinase and during glycolysis).Figure 4.14 Kinases transfer the γ-phosphate group from ATP to a substrate (or vice versa).Phosphorylases are transferases with an interesting function. They use phosphate groups to break apart another molecule in a process called phosphorolysis, similar to hydrolases breaking apart molecules using water (see Concept 4.2.04). A typical phosphorolysis reaction yields two product molecules, one of which is covalently bonded to the phosphate group (Figure 4.15).Although they are similar to hydrolases, phosphorylases are considered transferases because a group is transferred from the parent substrate to the phosphate group. For example, consider the enzyme glycogen phosphorylase. In the reaction catalyzed by this enzyme, Pi breaks off a glucose subunit from the glycogen polymer (a large molecule composed of multiple glucose units), forming a shortened glycogen and glucose 1-phosphate (two molecules become two different molecules). In this reaction, glycogen acts as a glucose donor, and the glucose is donated to the phosphate, which acts as an acceptor. Chapter 4: Enzyme Activity148Figure 4.15 Glycogen phosphorylase is an example of a transferase enzyme in which the acceptor is smaller than the transferred group.Transaminases are enzymes that transfer amine groups. They are also sometimes called aminotransferases. Typically, the transferase moves the α-amine group of an amino acid onto an (Figure 4.16).Although this process can be considered a redox reaction, the most important physiological result is the amine group transfer. Therefore, transaminases are considered transferases.Figure 4.16 Transaminases are examples of transferase enzymes.Polymerases are another example of transferases. DNA and RNA polymerases transfer a nucleotide from a dNTP or an NTP, respectively, onto a growing nucleic acid chain. The products are a nucleic acid, which has accepted a nucleotide, and a pyrophosphate (PPi), which has donated a nucleotide (Figure 4.17).Although polymerases may use NTPs, they are not ligases (Concept 4.2.07) because the nucleotide of the NTP is incorporated into the growing molecule. In contrast, the NTPs used by ligases are independent of the two molecules being joined. Chapter 4: Enzyme Activity149Figure 4.17 Polymerases are examples of transferase enzymes.4.2.04 HydrolasesHydrolases catalyze reactions in which water (H2O) is used to break apart a in a substrate molecule. This type of reaction is known as a hydrolysis reaction (Figure 4.18).Figure 4.18 Hydrolases use water to break a sigma bond.Recognizing Hydrolase Enzymes and Their ReactionsHydrolysis reactions often result in one substrate being broken into two products. However, if a bond within a cyclic structure is broken, only forms. This occurs with in the oxidative phase of the , for example.Most hydrolases are simply given the name of the substrate or functional group being acted upon with the suffix --ase (eg, esterases hydrolyze esters, glycosidases hydrolyze glycosidic bonds), making it difficult to determine whether an enzyme is a hydrolase based on its name alone. Instead, hydrolases can be recognized by chemical equation of the reaction they catalyze. The following sections list some common hydrolases and potential pitfalls in identifying an enzyme as a hydrolase.Commonly Encountered HydrolasesProteases are hydrolases that typically catalyze the hydrolysis of a peptide bond between two amino acids residues, as shown in Figure 4.19. Proteases are usually sequence-specific and only hydrolyze specific peptide bonds based on the identities or properties of the surrounding amino acids. In contrast to most other enzymes, some proteases have common names that do not end in --ase, so their status as proteases should be remembered. These enzymes include pepsin, trypsin, and chymotrypsin. Chapter 4: Enzyme Activity150Figure 4.19 Proteases are hydrolases that hydrolyze peptide bonds.Phosphatases are enzymes that hydrolyze the phosphate group from a phosphorylated substrate. Recall from Concept 4.2.03 that kinases add a phosphate group to substrates through a transfer reaction involving ATP. This involves the removal of a phosphate group from ATP, which is energetically very favorable. The reverse reaction, moving a phosphate back onto ADP to regenerate ATP, is typically energetically unfavorable. To circumvent this unfavorable reaction, phosphatases hydrolyze the added phosphate using water; no ATP is involved in a typical phosphatase-catalyzed reaction (Figure 4.20).Figure 4.20 Kinases add phosphate groups to a substrate through a transfer reaction involving ATP. Phosphatases remove phosphate groups from substrates by hydrolysis.Potential Pitfalls in Hydrolase RecognitionAlthough water is necessary in a hydrolase-catalyzed reaction, water\'s involvement alone is not sufficient to identify a reaction as hydrolysis. For example, some enzymes add water across a double bond. Although the pi bond breaks, the atoms involved remain connected afterward through the sigma bond, and the sigma bond is not broken. These reactions are catalyzed by (and dehydratases), which are a class of lyase (Concept 4.2.05), not hydrolases.Furthermore, some enzyme-catalyzed reactions do involve hydrolysis, but only as a secondary function. These enzymes are therefore grouped in another class. For example, all ligases (Concept 4.2.07) involve hydrolysis of an NTP, but this hydrolysis is coupled to the joining of two substrates together, which is their primary purpose. Many enzymes in other classes similarly hydrolyze an NTP to power an otherwise-endergonic reaction and are classified according to the enzyme\'s primary function. Chapter 4: Enzyme Activity151Enzymes should only be classified as hydrolases if hydrolysis alone is the main function of the enzyme (eg, phosphatases, proteases, phosphodiesterases, glycosidases). Enzymes that hydrolyze an NTP or another substrate to power a separate reaction are not typically classified as hydrolases.Concept Check 4.6The following enzyme-catalyzed reactions occur in glycolysis or gluconeogenesis. Both reactions remove a phosphate group from a substrate, and the relevant phosphate group is shown in red. For each reaction, categorize the enzyme first as a transferase or as a hydrolase, and then further classify it as a kinase, a phosphatase, or a phosphorylase.4.2.05 LyasesLyases catalyze either the removal or addition of a functional group, depending on the direction of the reaction. Importantly, functional group removal must not be hydrolytic or tied to a redox reaction; otherwise, the enzyme would be classified as a hydrolase (Concept 4.2.04) or an oxidoreductase (Concept 4.2.02), respectively.Instead, when lyases remove functional groups they leave behind a double bond (ie, they catalyze an ) or a cyclic ring. Similarly, when lyases act in the opposite direction and add functional groups, they do so across a double bond (ie, they catalyze an ) or by breaking open a ring (Figure 4.21). Chapter 4: Enzyme Activity152Figure 4.21 Lyases catalyze removal or addition of a chemical group by forming or breaking a double bond or ring.Similar to transferases (Concept 4.2.03), the functional group involved in a lyase-catalyzed reaction can vary greatly in size. In some cases, the functional group added is as small as a water molecule (H--OH) adding across a double bond of a much larger molecule. In other cases, the group being added (or the group being eliminated) is just as large as or larger than the molecule with the double bond or ring.Recognizing Lyase Enzymes and Their ReactionsThe double bond or the ring is a characteristic feature of a lyase-catalyzed reaction. However, many biomolecules have double bonds or rings that are not involved in every reaction, so classifying an enzyme based only on the presence of a double bond or ring in its reaction can lead to errors. Instead, a lyase-catalyzed reaction can be more easily identified by examining the number of substrates and products.Lyase enzymes catalyze addition and elimination reactions. Addition reactions join two molecules into one, so lyase-catalyzed additions have two substrates and one product. Elimination reactions remove a group from a molecule, so lyase-catalyzed eliminations have one substrate but two products, as shown in Table 4.5. Chapter 4: Enzyme Activity153Table 4.5 Examples of lyase-catalyzed reactions.Examples of LyasesAldolase, an enzyme in and gluconeogenesis, is an example of a lyase. The aldolase-catalyzed reaction is shown in Figure 4.22. During glycolysis, aldolase acts on fructose 1,6-bisphosphate, eliminating dihydroxyacetone phosphate (DHAP) from glyceraldehyde 3-phosphate (G3P). The double bond formed is a C=O double bond. Note that the \"functional group\" being eliminated (DHAP) is the exact same size as the molecule left behind (G3P). Chapter 4: Enzyme Activity154Figure 4.22 The reaction catalyzed by aldolase.Hydratases and dehydratases are enzymes that add water across a double bond or eliminate water to form a double bond, respectively (Figure 4.23). Fumarate hydratase (also known as fumarase) of the is an example of a hydratase. In , β-hydroxyacyl-ACP dehydratase acts as a dehydratase. Despite the use of water to break a pi bond, the carbon atoms involved in the double bond remain connected through their sigma bond, so these reactions are not hydrolysis.Figure 4.23 Hydration and dehydration reactions are catalyzed by lyases, not by hydrolases.Nucleotidyl cyclases such as adenylyl cyclase are examples of lyases that use elimination reactions to form ring structures instead of double bonds, as shown in Figure 4.24. A single NTP molecule acts as the sole substrate. Pyrophosphate is eliminated, and the remaining nucleoside monophosphate is cyclized into a 3′,5′-cyclic NMP (eg, cAMP). Chapter 4: Enzyme Activity155Figure 4.24 Adenylyl cyclase is an example of a lyase that catalyzes ring formation.Many synthases are also formally classified as lyases. However, \"synthase\" is an informal classification mostly used to designate enzymes that form larger molecules without the use of an external energy source such as ATP. Because lyases can catalyze addition reactions that join two molecules into one product, many lyases that operate primarily in that direction are called synthases.4.2.06 IsomerasesIsomerases catalyze reactions that change the structure of a single substrate. In other words, isomerases catalyze the changing of a substrate into a different (Figure 4.25). Depending on the enzyme, the catalyzed isomerization reaction can result in a constitutional isomer, a configurational isomer, or a conformational isomer.Figure 4.25 Isomerases catalyze the structural rearrangement of their substrate.Recognizing Isomerase Enzymes and Their ReactionsIsomerases catalyze the structural rearrangement of a molecule with no net functional group addition, elimination, or transfer between molecules. Therefore, most isomerases have only one substrate and one product. Many isomerases contain the term isomerase in their name; however, certain subclasses of isomerases use different terms. These terms will be discussed, along with the type of isomerization reactions they describe, in the following sections. Chapter 4: Enzyme Activity156Types of Isomerization Reactions: Constitutional Isomerization are isomers that have the same types and numbers of atoms but different covalent bond connectivity between them. The enzyme triose phosphate isomerase, which is involved in , is an example of an isomerase that produces constitutional isomers. Specifically, it interconverts an and a by changing the positions of covalent bonds (Figure 4.26).Figure 4.26 The interconversion of aldoses and ketoses is catalyzed by isomerases. The atoms and bonds shown in blue move, producing constitutional isomers.Mutases are a subclass of isomerase that catalyze intramolecular transfer reactions. In other words, they move a functional group substituent from one position to another position on the same molecule. An example of a mutase is phosphoglycerate mutase, which plays a key role in glycolysis. Phosphoglycerate mutase transfers a phosphate group from C3 of glyceric acid to C2, or vice versa (Figure 4.27).Figure 4.27 Mutases transfer substituents from one position on a molecule to another position on the same molecule.Protein disulfide isomerases are enzymes that cause a disulfide bond to move from one pair of cysteines to another pair of cysteines from the same molecule, as shown in Figure 4.28. Lesson 2.2 discusses how the formation and breaking of an individual disulfide bond is a redox process. However, because the net oxidation and reduction reactions both occur on the same molecule (ie, the protein), enzymes that catalyze these reactiosn are considered isomerases rather than oxidoreductases. Chapter 4: Enzyme Activity157Figure 4.28 Protein disulfide isomerases move disulfide bonds within the same molecule.Types of Isomerization Reactions: Configurational IsomerizationIn configurational isomers, all the atoms in the molecule remain bonded to the same atoms. However, the relative placement of atoms differs, leading to distinct molecules that cannot be superimposed or interconverted through freely rotating bonds. Configurational isomers (see Organic Chemistry Lesson 3.3) include cis/trans or E/Z isomers around a and R/S or ʟ/ᴅ isomers around a.Peptide bonds have double bond character due to. Therefore, peptide bonds can be described as with respect to the adjacent α-carbons. Lesson 2.1 discusses how most peptide bonds favor the trans configuration because it has much less than the cis configuration. Proline, however, experiences similar clashing---and therefore has similar free energy---in both configurations. Consequently, the peptide bond preceding a proline residue can exist in either the cis or the trans configuration. The enzyme peptidyl-prolyl isomerase catalyzes the exchange (Figure 4.29).Figure 4.29 A cis-trans isomerase interconverts a trans peptide bond with a cis peptide bond.Isomerization of a chiral center is another common configurational isomerization, and enzymes that catalyze these reactions often have unique suffix terms to describe them. For substrates with only one chiral center, configurational isomerization produces the enantiomer. If allowed to go to equilibrium, a 1:1 mixture of ---also known as a ---would be produced. Therefore, enzymes that invert the stereochemistry of a substrate\'s sole chiral center are called racemases (Figure 4.30). Chapter 4: Enzyme Activity158Figure 4.30 A racemase interconverts stereochemistry at a molecule\'s sole chiral carbon to produce a racemic mixture of enantiomers.If a substrate has multiple chiral centers, then isomerization of a single center forms a special type of known as an epimer (see Chapter 7). Therefore, isomerase enzymes that catalyze these reactions are known as epimerases (Figure 4.31).Figure 4.31 In a molecule with multiple chiral centers, epimerases change the stereochemistry at a single chiral carbon.Monosaccharides, the monomeric unit of carbohydrates (see Chapter 7), gain an additional chiral center when they cyclize. This new chiral center is known as the , and the two possible epimers are known as the. The interconversion of anomers is called mutarotation, and therefore enzymes that catalyze mutarotation are a subclass of epimerases called mutarotases (Figure 4.32).Figure 4.32 A mutarotase catalyzes the stereochemical inversion of a carbohydrate\'s anomeric carbon. Chapter 4: Enzyme Activity159Concept Check 4.7Suppose that glucose 6-phosphate can be converted to Product A, Product B, or Product C, and that each conversion occurs through only one enzyme.Which enzyme is phosphoglucose isomerase, which is phosphoglucomutase, and which is phosphoglucose epimerase?4.2.07 LigasesLigases are the sixth class of enzymes. They catalyze the joining of two molecules using the hydrolysis of ATP (or another nucleoside triphosphate) for the energy needed to do so, as shown in Figure 4.33.Note that there are two net effects of a ligase-catalyzed reaction: two substrates are joined and an NTP molecule is hydrolyzed. Although NTP hydrolysis is an important result of the reaction energetically, it typically is not the main physiological result. For this reason, the NTP in a ligase reaction is often called a coenzyme of the ligase (like the redox coenzymes discussed in Concept 4.2.02).Because NTP hydrolysis is not the primary function of the enzyme, these enzymes are distinct from hydrolases. When classifying enzymes, it is important to consider all processes catalyzed by the enzyme to determine the enzyme\'s proper category. Chapter 4: Enzyme Activity160Figure 4.33 Ligases hydrolyze a nucleoside triphosphate (eg, ATP) to covalently join two substrate molecules together.Recognizing Ligase Enzymes and Their ReactionsLigase enzymes can be recognized by the reactions they catalyze. Typically, two substrates are joined into one product, and an NTP coenzyme (ie, ATP, GTP, CTP, or UTP) is hydrolyzed to NDP and an inorganic phosphate (Pi). Some ligases instead hydrolyze the NTP into an NMP and pyrophosphate (PPi). Although NTP hydrolysis is highly exergonic, ligase-catalyzed reactions may also proceed in the reverse direction depending on the free energy change ∆G of the coupled reaction. In this case, ligase reactions break apart a molecule while forming NTP.Ligase enzymes can also be recognized by their names. Many ligase enzymes have the term ligase in their name (eg, ). Other ligases include the term synthetase. As introduced in Concept 4.2.05, the term synthase (no \"et\" in the name) is usually used to describe enzymes that join molecules without NTP hydrolysis. In contrast, the term synthetase (with the \"et\") is used to describe enzymes that join molecules with NTP hydrolysis. While synthases can be found in several enzyme classes, the term synthetase is generally synonymous with ligase.One example of a synthetase is succinyl-CoA synthetase, an enzyme in the. The ligation reaction it catalyzes joins succinate and coenzyme A to form succinyl-CoA, while consuming GTP in the process.Succinate+CoA-SH+GTP⇌Succinyl-CoA+GDP+PiSuccinyl-CoA synthetase is also an example of a reversible ligase-catalyzed reaction. During the citric acid cycle, succinyl-CoA is broken apart and GTP is formed from GDP and Pi.Succinyl-CoA+GDP+Pi⇌Succinate+CoA-SH+GTP Chapter 4: Enzyme Activity161Concept Check 4.8Consider the following two enzyme-catalyzed reactions.Based on these reaction descriptions, fill in the blanks in the following paragraphs:Reaction 1 synthesizes the molecule XYZ \_\_\_\_\_\_\_\_\_ (using / without using) ATP. Therefore, an appropriate name for its enzyme is XYZ \_\_\_\_\_\_\_\_\_\_\_ (synthase / synthetase). This reaction joins two molecules into one and involves addition across a double bond. Therefore, its enzyme is most likely classified as a \_\_\_\_\_\_\_ (ligase / lyase).In contrast, Reaction 2 synthesizes the molecule XYZ and \_\_\_\_\_\_\_\_\_\_\_ (uses / does not use) the energy in ATP to do so. Therefore, an appropriate name for its enzyme is XYZ \_\_\_\_\_\_\_\_\_\_ (synthase / synthetase). ATP is hydrolyzed into ADP + Pi. Despite this, the enzyme is not classified as a hydrolase, but rather as a \_\_\_\_\_\_\_\_\_ (ligase / lyase), because of its function of joining two molecules together.4.2.08 TranslocasesThe seventh enzyme classification is the translocase class. Translocases are a relatively new classification of enzyme. As such, they may not appear on the exam; however, they still catalyze an important subset of cellular processes and are worth reviewing.Translocases move a substance across a lipid bilayer membrane (Figure 4.34). Unlike other transporters that mediate passive , translocases couple substance movement with a catalyzed chemical reaction. Therefore, translocases are responsible for across a membrane. Chapter 4: Enzyme Activity162Figure 4.34 Translocases couple the movement of a solute across a membrane with a catalyzed chemical reaction (eg, ATP hydrolysis, NADH oxidation).Most translocases couple solute movement to either a hydrolysis reaction or to a redox reaction. Consequently, these enzymes were once classified as hydrolases or oxidoreductases, respectively. Complexes I, III, and IV of the are examples of translocases that couple proton movement to the reduction of a redox cofactor.The is another example of a translocase that moves multiple solutes. In this case, the Na+/K+ pump also moves multiple types of solutes to different sides of the membrane. Solute movement is linked to a chemical reaction (ie, ATP hydrolysis), which classifies the Na+/K+ pump as a translocase.Like other enzymes, translocases can also catalyze their reactions in both directions. In other words, instead of hydrolyzing ATP to pump a solute against its electrochemical gradient, a translocase can also form ATP as a solute moves down its gradient. , the final enzyme in the oxidative phosphorylation pathway, is a translocase that operates in this direction.4.2.09 Receptor EnzymesReceptor enzymes are not a distinct class of enzymes in that they do not catalyze a distinct type of reaction or reaction-coupled process. Instead, receptor enzymes are each classified under one of the discussed in the previous concepts of this lesson. However, receptor enzymes also have additional properties that make them worth reviewing.In general, receptors are the through which cells interact with their external environment. Because the enclosing the cell is only , most external stimuli interact only with the ---they do not interact directly with anything inside the cell. Chapter 3 discusses how some membrane proteins bind extracellular (ie, ligands that activate a receptor), which leads to intracellular conformational changes and intracellular effects (eg, ligand-gated ion channels, GPCRs).Receptor enzymes all act on a similar principle. Each has an extracellular binding domain and an intracellular catalytic (ie, enzyme) domain, as shown in Figure 4.35. The catalytic domain is typically inactive until an agonist binds. Binding leads to a conformational change, which then leads to an increase in catalytic activity. In this way, receptor enzymes exhibit allosteric regulation. Although receptor enzymes can belong to any class of enzyme, many are either kinases (a type of transferase) or guanylyl cyclases (a type of lyase). Chapter 4: Enzyme Activity163Figure 4.35 Receptor enzymes bind an external signal, which activates their catalytic activity. Activation of receptor enzymes leads to downstream signal propagation.Of the receptor kinases, many are receptor tyrosine kinases (RTKs). As their name implies, RTKs phosphorylate tyrosine residues when activated. Note that this contrasts with most soluble (ie, nonmembrane receptor) protein kinases, which typically phosphorylate serine or threonine residues. Consequently, phosphotyrosine (pTyr) is relatively scarce, which allows it to act as a potent intracellular signal. Downstream proteins in the signaling pathway are \"recruited\" by pTyr. In other words, they bind to and are stimulated by pTyr on the substrate protein. This leads to propagation of the signal (Figure 4.36). Chapter 4: Enzyme Activity164Figure 4.36 Phosphotyrosine \"recruits\" effector proteins that bind to it. These activated effectors can then recruit downstream effectors, leading to signal transduction.One important mechanistic feature of RTKs is their autophosphorylation. RTKs are typically dimers or become dimers upon agonist binding, bringing the catalytic domains of each monomer close together. Each monomer then phosphorylates a tyrosine residue within the other monomer. This self-phosphorylation further activates the RTK dimer and provides pTyr residues, which recruit the receptor\'s substrate (Figure 4.37). The insulin receptor, discussed in Unit 4, is an example of an RTK. Chapter 4: Enzyme Activity165Figure 4.37 The binding of a ligand induces RTKs to undergo autophosphorylation. This helps to recruit substrate and increases the RTK\'s catalytic activity.The second major class of receptor enzymes is receptor guanylyl cyclases (Figure 4.38). Receptor guanylyl cyclases are less commonly tested on the exam but play important roles in (eg, atrial and brain natriuretic peptide receptors, nitric oxide receptors) (see Biology Lesson 13.1). In addition, receptor guanylyl cyclase action demonstrates the role that receptor enzymes play in and.Upon binding their agonist, receptor guanylyl cyclases catalyze a cyclization reaction that converts GTP to 3′,5′-cyclic GMP and pyrophosphate (PPi). The resulting cGMP then acts as a to activate protein kinase G (PKG), which can then phosphorylate various substrates and cause various effects.One type of guanylyl cyclase enzyme is a soluble (ie, nonmembrane) receptor. This receptor, abbreviated as sGC, resides in the cytosol and binds a ligand that can diffuse across the cell membrane---nitric oxide. Activated sGC then produces cGMP, which activates PKG, leading to downstream effects. The diffusion of an agonist through the membrane is relatively uncommon, but it can also happen with lipid agonists for other receptors (see Lesson 3.2 and Lesson 9.3).