BIOC3570_F24_Mass_Spec PDF
Document Details
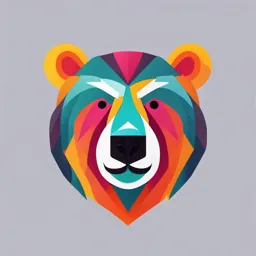
Uploaded by YouthfulGothicArt
Tags
Summary
This document provides a summary of mass spectrometry applications in chemistry, focusing on fundamental principles and analytical techniques. It covers topics such as atomic masses, molar masses, isotopic masses, and the theory behind mass spectrometry, including the use of mass spectrometry in proteomics.
Full Transcript
Mass spectrometry Weighing elephants by seeing how they fly… Mass spectrometry Mass spectrometry (MS) analyses the acceleration of charged analytes in an electrical field under a vacuum Analysis yields the mass to charge ratio (m/z) of the analyte Because there is no friction or diffusion u...
Mass spectrometry Weighing elephants by seeing how they fly… Mass spectrometry Mass spectrometry (MS) analyses the acceleration of charged analytes in an electrical field under a vacuum Analysis yields the mass to charge ratio (m/z) of the analyte Because there is no friction or diffusion under a vacuum, MS results are extremely precise (up to 1 in 106) Because ions are detected directly, MS is also extremely sensitive MS can precisely identify proteins from trace amounts This makes MS an extremely powerful analytical technique that can be used to identify a protein or proteome, identify modifications, or even characterize protein dynamics Applications of protein mass spectrometry Proteomics: Identification of unknowns by proteolytic digestion, peptide mass measurement, and comparison to genome databases Quantitative proteomics: Measuring changes in protein expression Protein sequencing Characterizing splice variants and post-translational modifications Structural biology (native MS; H/D exchange, etc.) MS and isotopes In the ”wet” lab, we weigh very large numbers of molecules in a group In MS, in contrast, we are weighing molecules as individual objects (though peaks arise from summing the contributions of many individual molecules) The high resolution of MS resolves the individual isotopes of analytes Atomic masses Atoms are composed of electrons and a nucleus The nucleus is comprised of neutrons and protons, which together contribute most of the mass of the atom Nuclei are held together by the strong nuclear force, which overcomes the Coulomb repulsion of the protons Each element is found as several isotopes, which have the same number of protons (Z), but different numbers of neutrons (N) Different isotopes are differentiated by A, where A = Z + N e.g. 12C: A = 12; Z = 6; N = 6 14C: A = 14; Z = 6; N = 8 Atomic masses and the nuclear mass defect Atomic mass unit (amu) / 1/12th × mass of 12C atom = 1.6606 × 10-27 kg 12C = 12 (exactly, by definition) Mass of a proton: p = 1.0073 amu Mass of a neutron: n = 1.0087 amu Note that the mass of 6 protons, 6 neutrons and 6 electrons sums to 12.0993, but 12C weighs exactly 12.0000 The mass of atoms is less than the sum of the masses of its constituent particles Einstein explained that the “missing” mass represents the binding energy of the nucleus, according to E = mc2 Most biologically abundant elements are nearly monoisotopic Monoisotopic mass: the sum of the exact masses of the most abundant naturally occurring stable isotope of each atom in the molecule. Biological molecules are made predominantly from light elements (C,H,O,N,S,P) Most of these light elements are nearly monoisotopic: H 99.99% 1H (150 ppm 2H) C 98.9% 12C (1.1% 13C) N 99.6% 14N (0.4 % 15N) O 99.8% 16O P 100 % 31P Sulfur is a notable exception: S: 94.9% 32S + 0.76% 33S + 4.3% 34S + 0.02% 36S Atomic masses The atomic mass is the average of the masses of the isotopes of an element, weighted by their natural abundances. e.g. Cl: 75.8% × 34.97 (35Cl) + 24.2% × 36.97 (37Cl) = 35.45 This value is shown on the Periodic Table Note that natural abundances of isotopes can vary by environment: Exposure to or radiation will produce heavier elements (e.g. 14C) Biological and chemical processes can concentrate certain elements (e.g. 13C is concentrated in the atmosphere by photosynthesis) Molar masses When we calculate the mass of a molecule in chemistry, we sum the atomic masses of all constituent atoms We can do this because, with enormous numbers of molecules in our sample, each isotope should be present at its average abundance in each possible position e.g.: Benzene: C6H6 (molar mass = 78.11) MW = 6 x 12.011 + 6 x 1.008 = 78.11 Exact monoisotopic masses In mass spectrometry, we are measuring the masses of individual molecules The weight is the sum of the exact isotopes present in this molecule E.g. if benzene is made from 12C and 1H then: 12C 1H = 6 × 12.00 + 6 × 1.0078 = 78.0468 6 6 This value is the exact (monoisotopic) mass Note that the monoisotopic mass differs from the molar mass (recall benzene molar mass = 78.11) For convenience we often refer to their nominal mass: nearest integer mass e.g. 12C61H6 = 78 MS and isotopes Bradykinin Melittin Ubiquitin C50H73N15O11 C131H229N39O31 C378H629N105O118S1 Isotopic peaks of amino acids (or their polymers, proteins) are different by 1 Da) The components of a mass spectrometer: MS experiment 10-3 - 10-8 mbar ionization mass ion source analyzer detector collision mass cell analyzer Proteins are challenging MS targets For MS, the analyte needs to be in the gas phase (and then transferred to a vacuum), and it needs to be charged For small molecules, bombarding the sample with electrons or gases knocks electrons off The resulting charged species that are prone to rearrange or fragment, but analysis takes rearrangements into account Proteins are very challenging because 1) they do not easily vaporize and 2) fragmenting proteins randomly into small pieces creates an intractable mess Getting proteins to vaporize intact has been compared to the challenge of getting elephants to fly Two solutions have been found to this dilemma: matrix assisted laser desorption ionization (MALDI) and electrospray ionization (ESI) 540 - 542 Ion source #1: Electrospray ionization Electrospray ionization (ESI) is an alternative ion source A dilute solution of the analyte is buffered to provide electrical conductivity This solution is sprayed from the tip of a metal capillary to which a high potential (kV) is applied. The combination of vacuum and electrostatic repulsion causes droplets to evaporate and repeatedly fragment The end products are the naked analyte molecules carrying a large net charge ESI is a continuous ionization method that is routinely coupled to an HPLC as a separation step Electrospray ionization (ESI) generally samples are separated using an HPLC prior to injection into the MS Biological Mass Spectrometry, Kaltashov and Eyles, 2012 Net charge in ESI In electrospray produces gas phase intact ionized macromolecules for mass analysis in a mass spectrometer The positive-ion-mode is dominant – produces positively-charged analyets. It is not uncommon to hear that the extent of multiple charging of unfolded polypeptides in ESI MS must be limited by the number of available ionizable sites. This is wrong! Both polycationic and polyanionic species could be generated from a protein solution at any given pH. The extent of multiple charging is indeed determined by the protein geometry in solution, not the number of available basic sites. Biological Mass Spectrometry, Kaltashov and Eyles, 2012 SLIDE FROM THE ELECTROPHORESIS CHAPTER SDS-PAGE is routinely used to monitor the steps in a protein purification. Purification of the human ATR. SDS-PAGE was used to assess the purification. lane 1, molecular mass markers; lane 2, soluble cell-free extract from the E. coli expression strain; lane 3, ammon. sulfate precipitate; lane 4, hydroxyapatite eluate; lane 5, Anion exchange fraction. Leal et al., Human ATP:cobalamin adenosyltransferase and its interaction with methionine synthase reductase, J. Biol. Chem. 279: 47536-47542, 2004. ESI spectrum of a denatured protein Electrospray ionization of a molecule M produces a series of ions that are multiply charged: The ESI mass spectrum of a 37 kDa protein (human serum transferrin) Note that the spectrum has a large number of peaks that all represent the same molecule in different charge states These ions differ in (m/z) – but in a systematic manner. Adjacent peaks differ by 1 charge unit and 1 proton mass. These m/z values are valid observations, but we are much more interested in the protein mass than m/z Fortunately, this complex spectrum (manifold) can be deconvoluted mathematically to yield the mass of the molecule ESI mass analysis Several different charge carriers are observed in ESI, the most common are protons (esp. after HPLC) and alkali metal cations (particularly Na+ and K+). The m/z value of ions can be calculated as If the charging is mostly due to protonation (e.g. after HPLC separation and desalting, the equation above simplifies to M is the mass of the (neutral) protein n is net charge and is an integer The unit for m/z is Thompson (Th), in honour of J. J. Thomson Biological Mass Spectrometry, Kaltashov and Eyles, 2012 Direct calculation of charge from isotopic clusters n+ charge state The spacing between the isotopes of a protein is 1 Da. Therefore, 𝑚 𝑀+𝑛 𝑚 = 𝑟𝑒𝑎𝑟𝑟𝑎𝑛𝑔𝑒 ⇒ 𝑀 = 𝑛 × −𝑛 𝑧 𝑛+ 𝑛 𝑧 𝑛+ M = 22 x 1689.6 -22 = 37,149.2 Da Biological Mass Spectrometry, Kaltashov and Eyles, 2012 Deconvolution of ESI mass spectra where resolution is not sufficient to resolve isotopes Consider any two successive charge states (n and n+1) of a protein : ESI-MS Intensity (n+1)+ n+ m/z 𝑚 𝑀+𝑛 𝑚 = 𝑟𝑒𝑎𝑟𝑟𝑎𝑛𝑔𝑒 ⇒ 𝑀 = 𝑛 × −𝑛 𝑧 𝑛+ 𝑛 𝑧 𝑛+ 𝑚 𝑀+𝑛+1 𝑚 𝑚 = 𝑟𝑒𝑎𝑟𝑟𝑎𝑛𝑔𝑒 ⇒ 𝑀 = 𝑛 × + −𝑛−1 𝑧 𝑛+1+ 𝑛+1 𝑧 𝑛+1+ 𝑧 𝑛+1+ The left side are the same therefore the right sides must be 𝑚 𝑚 𝑚 𝑛× + −𝑛−1=𝑛× −𝑛 This formula calculates n (charge) 𝑧 𝑛+1+ 𝑧 𝑛+1+ 𝑧 𝑛+ 𝑚 −1 𝑚 𝑚 𝑚 𝑧 𝑛+1+ −1=𝑛×[ − ] ⇒ 𝑛= 𝑚 𝑚 𝑧 𝑛+1+ 𝑧 𝑛+ 𝑧 𝑛+1+ − 𝑧 𝑛+ 𝑧 𝑛+1+ Example: The observed ESI mass spectrum of the 37 kDa protein human serum transferrin consists of a series of peaks. Calculate the exact mass. Consider two sequential peaks, e.g.: n+ = 1689.6 Th ; n+1+ = 1616.2 Th 𝟏𝟔𝟏𝟔. 𝟐 − 𝟏 𝟏𝟔𝟏𝟓. 𝟐 𝐧= = = 𝟐𝟐 (remember, n is an integer) 𝟏𝟔𝟖𝟗. 𝟔 − 𝟏𝟔𝟏𝟔. 𝟐 𝟕𝟑. 𝟒 M = 22 x 1689.6 -22 = 37,149.2 Da In practice, an accurate value is obtained by performing the same calculation for each peak and averaging the results. Electrospray MS: application to PTMs glycoforms Mass (Da) Bondarenko et al., J. Am. Soc. Mass Spectrom. 20: 1415-1424, 2009. Intact IgG (0.9 g) was analyzed using electrospray An average of 1000 scans for 5 min was used to obtain good signal:noise (a) ESI mass spectrum of IgG antibody, theoretical mass = 147,250 Da (G0). (b) More detailed view of a section of ESI mass spectrum note the presence of multiply charged ions with 54, 55, and 56 protons (c) Deconvoluted ESI mass spectrum of the IgG. Note that the different glycoforms differ in mass by ~162 Da, or the mass of one hexose (minus water) 542 - 547 Ion source #2: MALDI For Matrix Assisted Laser Desorption/Ionization (MALDI) the protein is embedded in a solid matrix This matrix is crystals of a small aromatic acid The matrix material is water soluble, UV absorbant, and has low vapour pressure E.g. sinapinic acid absorbs strongly at N2 laser sinapinic acid (found in emission frequency (337 nm) wine) Protein samples at ~1 pM (10-12 M) are dissolved with matrix material, then spotted on a metal plate, and allowed to dry MALDI sample preparation 542 - 547 The UV–Vis absorption spectra and structures of popular MALDI matrices nitrogen laser (337 nm) (a, solid black trace) 2-(4-hydroxyphenylazo)–benzoic acid (HABA), (b, solid gray trace) a-cyano- 4-hydroxy cinnamic acid (aCHCA), (c, dashed black trace) 2,5-dihydroxybenzoic acid (DHBA), and (d, dashed gray trace) 3,5-dimethoxy-4-hydroxy cinnamic acid (sinapinic acid or SA). Biological Mass Spectrometry, Kaltashov and Eyles, 2012 MALDI The dried matrix absorbs light from a UV laser, causing it to vaporize The protein, largely unaffected by the laser, is carried into the gas phase by evaporating matrix Protons transferred from the acidic matrix material gives the protein a positive charge The electrostatic field then accelerates these charged species into the mass analyzer Biological Mass Spectrometry, Kaltashov and Eyles, 2012 MALDI TOF MALDI ionization is most commonly used with a TOF analyzer MALDI generates ions with small charges – mostly +1, sometimes 2+ This means that proteins have an m/z of either M+H or (M+2H)/2 TOF is able to resolve these very high m/z ions better than most methods The matrix creates a lot of noise at m/z