Photosynthesis - Light-Dependent and Light-Independent Stages PDF
Document Details
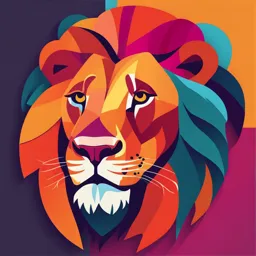
Uploaded by StylizedAppleTree9743
Bogaerts International School
Tags
Summary
This document provides a detailed overview of photosynthesis's light-dependent and light-independent stages. It covers the processes, including the role of chlorophyll, enzymes, and the Calvin cycle, providing a comprehensive understanding of this crucial biological process. The document also highlights the raw materials and energy requirements.
Full Transcript
# Photosynthesis ## Light dependent stage - Sites of the light-dependent phase of photosynthesis and the Calvin cycle within the chloroplast: - Light dependent -> thylakoid membrane - Calvin cycle -> stroma - The terms "dark phase", "light-independent phase", and "Calvin cycle" are used...
# Photosynthesis ## Light dependent stage - Sites of the light-dependent phase of photosynthesis and the Calvin cycle within the chloroplast: - Light dependent -> thylakoid membrane - Calvin cycle -> stroma - The terms "dark phase", "light-independent phase", and "Calvin cycle" are used to describe the fixation of CO₂ resulting in glucose formation. - Dark phase -> misleading because it doesn't require darkness, only no light. - Light independent -> more accurate because it doesn't depend directly on light. - Calvin cycle -> best - All these terms reference the process. - Why chlorophyll detached from chloroplasts fluoresces: - Chlorophyll absorbs light energy but can't transfer the energy to other molecules, so the excited electrons return to their ground state, releasing energy as light (fluorescence) and heat. - Products of light-dependent reactions: ATP, NADPH, O₂, and protons - Photolysis of water: - Source of electrons for PSII - Source of electrons for PSI - Evolution of O₂ during photosynthesis is due to photolysis of H₂O (gives electrons for PSII). - Functions of electron carriers in PSI & PSII: - PSII -> transfer electrons through ETC to generate a proton gradient for ATP synthesis. - PSI -> transfer electrons to NADP⁺, reducing it to NADPH. - Equation for photolysis of H₂O: - 2H₂O + light + PSII -> 4H⁺ + 4e⁻ + O₂ - Equation for ATP in the chloroplast: - ADP + Pi + ATP synthase -> ATP - Equation for NADPH from NADP⁺: - NADP⁺ + 2e⁻ + PSI -> NADPH - Differences between cyclic and non-cyclic photophosphorylation: - Electron pathway - Cyclic: electrons cycle back to PSI. - Non-Cyclic: electrons flow from H₂O to NADP⁺. - Electron source: - Cyclic: no use of H₂O (photolysis) - Non-Cyclic: electrons come from photolysis of H₂O. - Products - Cyclic: ATP - Non-Cyclic: ATP, O₂, NADPH. - Primary function: - Cyclic: makes extra ATP - Non-Cyclic: supports the Calvin cycle (ATP & NADPH production). - What happens to electron energy as it goes from H₂O to NADP⁺? - Electron energy is high when light excites it in PSII and it loses energy while powering proton pumps in ETC. Then, it gains energy when re-excited in PSI and transfers it to NADP⁺, forming high energy NADPH. - Hill reaction: Photolysis of H₂O during light-dependent reactions that creates O₂, protons, and electrons. - Chemiosmotic theory: explains how chloroplasts produce ATP using channel protein ATPase/ATP Synthase: - Light energy powers the movement of electrons through ETC in the thylakoid membrane, creating a proton gradient. - Protons flow back into the stroma through ATP synthase, driving phosphorylation of ADP to ATP. - Source of protons: photolysis of H₂O - Ultimate destination of protons: flow back into stroma through ATP synthase. - Raw materials for light-dependent reactions: H₂O, light energy, NADP⁺, ADP & Pi - Ultimate destination of electrons in non-cyclic photophosphorylation: electrons ultimately reduce NADP⁺ to NADPH. ## Light-independent stage - Carbon fixation: the process by which inorganic carbon from CO₂ is converted into organic compounds, like 3-phosphoglycerate (PGA), during the Calvin cycle. - What is meant by reduction and identify it in the Calvin cycle: - Reduction is the gain of electrons/hydrogen. - In the Calvin cycle, PGA is reduced to glyceraldehyde-3-phosphate (G3P/PGAL) using ATP & NADPH. - Sources of raw materials for the Calvin cycle: - CO₂ (atmosphere) - ATP & NADPH (light-dependent reactions) - Why is the Calvin cycle also known as the light-independent reaction? - It doesn't require light directly. Instead, it uses ATP & NADPH generated in light-dependent reactions to fix CO₂ and synthesize glucose. - The Calvin cycle equation: - 6CO₂ + 18ATP + 12NADPH + 12H⁺ -> C₆H₁₂O₆ + 18ADP + 18Pi + 12NADP⁺ - Parts of the Calvin cycle: - Carbon fixation: CO₂ combines with ribulose-1,5-bisphosphate (RuBP) to form PGA. - Reduction: PGA is reduced to G3P using ATP & NADPH. - Regeneration: some G3P is used to regenerate RuBP, requiring ATP. - Components in the Calvin cycle: - RuBP -> combines with CO₂ in carbon fixation. - PGA -> first stable product of CO₂ fixation. - BPGA -> formed when PGA is phosphorylated using ATP. - PGAL/G3P -> produced when BPGA is reduced by NADPH. - RuP -> intermediate in RuBP regeneration. - Importance of enzyme RuBisCO: - Catalyzes the first step of carbon fixation, combining RuBP with CO₂. - Most abundant enzyme on Earth and crucial for photosynthesis. - How many total molecules of CO₂, NADPH, and ATP are needed for 1 glucose molecule formation? - 6 CO₂ - 18 ATP - 12 NADPH - How is glucose made from the Calvin cycle products? - Calvin cycle products make 2 molecules of G3P per 6 CO₂. The G3P molecules are used to synthesize glucose in the cytoplasm. - Why did photosynthesis evolve before O₂ was present? - As O₂ levels rose, RuBisCO began combining with O₂ rather than CO₂, leading to photorespiration. # Limiting Factors - Limiting factor from a graph: plateau in the graph. - Factors affecting photosynthesis rate: - Light intensity - CO₂ concentration - Temperature - Water availability - Difference in photosynthesis curves for light and shade plants: - Light plants have a higher light saturation point and photosynthetic rate. - Shade plants have a lower light saturation point and slower photosynthetic rate. - Law of limiting factors: When a process is controlled by multiple factors, the rate is determined by the factor closest to its minimum value. - Compensation point for photosynthesis: The light intensity at which the rate of photosynthesis equals the rate of respiration, on a graph where net gas exchange = 0. - Gross vs Net Photosynthesis Rate: - Gross: total CO₂ fixed during photosynthesis. - Net: gross photosynthesis rate minus CO₂ used in respiration. # Respiration ## Electron Transport Chain - Electrochem gradient -> this movement stores energy used for ATP synthase via chemiosmosis. - Proton pump (stores energy in form of electrochem gradient): - Proton pump proteins in membranes actively transport protons across a membrane. - It creates a difference in charge and H⁺ concentration across the membrane. ## Mitochondria - Structure & function of mitochondria: - Function: Produces ATP through aerobic respiration by oxidizing glucose derivatives. - Structure: - Outer membrane -> lets small molecules pass through. - Inner membrane -> houses ETC & ATP synthase. - Cristae (folds) -> increase surface area for ETC. - Matrix -> enzymes for Krebs cycle. - Main stages of aerobic respiration of carbohydrates: - Glycolysis -> in cytoplasm, glucose breaks into 2 pyruvate, producing 2 ATP and 2 NADH. - Link Reaction -> in mitochondrial matrix, pyruvate is converted to 2C acetyl-CoA, releasing CO₂ and generating NADH. - Krebs Cycle -> acetyl-CoA oxidized to CO₂, generating NADH, FADH₂, & ATP. - ETC -> NADH & FADH₂ donate electrons, driving proton pumps & ATP synthesis. - Proteins of respiratory chain: - Complex I (NADH dehydrogenase) -> transfers electrons from NADH to ubiquinone. - Complex II (succinate dehydrogenase) -> accepts electrons from FADH₂. - Complex III (cytochrome b-c₁ complex) -> transfers electrons to cytochrome c. - Complex IV (cytochrome c oxidase) -> transfers electrons to O₂, forming H₂O. - Connection between metabolism of proteins/fats and respiration: - Proteins -> amino acids deaminated to enter the Krebs cycle as intermediates. - Fats -> broken into glycerol (enters glycolysis) and fatty acids (converted to acetyl-CoA via β-oxidation). - Equations: - Respiration: C₆H₁₂O₆ + 6O₂ + 6H₂O -> 6CO₂ + 12H₂O - Aerobic: C₆H₁₂O₆ + 6O₂ → 6CO₂ + 6H₂O + 32ATP. - Glycolysis: C₆H₁₂O₆ + 2NAD⁺ + 2ADP + 2Pᵢ → 2CH₃-CO-COOH + 2NADH + 2H⁺ + 2ATP. - Fermentation (lactic acid): C₃H₆O₃ + NADH → C₃H₆O₃ + NAD⁺. - Glucose: C₆H₁₂O₆ -> pyruvate -> C₃H₄O₃. - Lactic acid: C₃H₆O₃ + NADH → C₂H₅OH + CO₂ + NAD⁺. - Ethanol: C₃H₆O₃ + NADH -> C₂H₅OH + CO₂ + NAD⁺. - ATP gain in aerobic vs anaerobic respiration: - Aerobic: 32 per glucose molecule. - Anaerobic (fermentation): 2 per glucose molecule as it relies only on glycolysis. - ATP Role: - Stores energy between bonds of its phosphate groups. - Bond is broken by hydrolysis, this releases energy. - Control rate of respiration by ATP & ADP concentration: - High ATP: inhibits key enzymes. - High ADP: stimulates respiration to produce more ATP. - Substrate & final products of: - Glycolysis: S -> glucose; FP -> pyruvate, ATP, NADH. - Link Reaction: S -> pyruvate; FP -> acetyl-CoA, CO₂, NADH. - Krebs cycle: S -> Acetyl-CoA; FP -> CO₂, NADH, FADH₂, ATP. - Respiration chain: S -> NADH, FADH₂; FP -> ATP, H₂O. ## Aerobic vs Anaerobic Respiration - O₂ requirement: - Aerobic: O₂ needed as final electron acceptor. - Anaerobic: O₂ not needed, uses other acceptors or no ETC. - Location: - Aerobic: mitochondria. - Anaerobic: cytoplasm. - End products: - Aerobic: H₂O, ATP. - Anaerobic: lactic acid or ethanol & CO₂ (plants). - ETC: - Aerobic: present, electrons passed to O₂. - Energy yield (ATP): - Aerobic: 32 per glucose molecule. - Anaerobic: 2 per glucose molecule. - Efficiency: - Aerobic: high, 40% of glucose's energy is captured. - Anaerobic: low, energy captured only via glycolysis. - Proton gradient: - Aerobic: pumps H⁺ into intermembrane space, creating proton-motive force. - Anaerobic: no proton gradient. ## ATP Synthase - Protein complex in the inner mitochondrial membrane that produces ATP: - Proton movement: protons move down their gradient from the intermembrane space to the matrix through ATP synthase. - Rotor movement: this movement generates mechanical energy. - ATP production: this rotation drives conformational changes in the F₁ subunit (matrix-facing part), which catalyzes the synthesis of ATP. - Key process: each full rotation generates ~3 ATP molecules. # Applications of Fermentation - Bread: yeast produces CO₂ (dough rises) & ethanol (evaporates). - Wine/beer: Yeast ferments sugars into ethanol & CO₂. - Yoghurt/cheese: bacteria ferment lactose into lactic acid, thickening yogurt and curdling milk for cheese. # Importance of Processes in the Cycle of Matter and Energy - Photosynthesis: converts CO₂ and sunlight into organic molecules and O₂. - Respiration: breaks down organic molecules to produce energy, releasing CO₂. - Fermentation: maintains glycolysis in anaerobic conditions, producing byproducts. # Carbon Cycle - Organic molecules: photosynthesis incorporates CO₂ into organic molecules. - Fossil fuels: decomposed plants/animals store carbon, burning releases CO₂. - CO₂: released during respiration & burning, absorbed by plants in photosynthesis. # Transcription & Translation - Role Of Nucleotides: - Building blocks of RNA. - RNA polymerase uses nucleotides to synthesize complementary RNA strands from a DNA template. - Correct pairing ensures accurate transfer of genetic information. - ATP (nucleotide) powers transcription & translation. ## Transcription - Transcription: The process by which a segment of DNA is copied into RNA (specifically mRNA) by the enzyme RNA polymerase. - The first step of gene expression. - Cistron: a functional unit of DNA that encodes a single polypeptide (protein). It's equivalent to a gene. ## Differences between prokaryotic and eukaryotic protein synthesis: - Location: - Prokaryotes: cytoplasm. - Eukaryotes: nucleus and cytoplasm. - mRNA type: - Prokaryotes: polycistronic. - Eukaryotes: monocistronic. - Transcription & translation: - Prokaryotes: coupled. - Eukaryotes: separate. - RNA Processing: - Prokaryotes: none. - Eukaryotes: extensive (5' cap, poly-A tail, splicing of introns). - Ribosome size: - Prokaryotes: 70S (50S + 30S subunits). - Eukaryotes: 80S (60S + 40S subunits). - Introns and exons: - Prokaryotes: absent. - Eukaryotes: present, introns spliced out. - Start codon recognition: - Prokaryotes: uses a Shine-Dalgarno sequence for ribosome binding. - Eukaryotes: uses a 5' cap for binding. - Translation initiation: - Prokaryotes: initial tRNA carries formyl-methionine. - Eukaryotes: same but methionine. ## Transcription Process in Eukaryotes - Initiation: RNA polymerase binds to the promoter region, unwinding DNA to start RNA synthesis. - Elongation: RNA polymerase moves along the template strand, adding complementary RNA nucleotides to the growing RNA strand. - Termination: RNA polymerase reaches a termination signal, releasing the newly formed RNA molecule. ## Transcription Unit - Transcription unit: a stretch of DNA transcribed into RNA, including the promoter, coding sequence, and terminator. It's recognized by specific sequences, like the TATA box in eukaryotes. ## Template vs Non-template strand - Template strand: refers specifically to the DNA strand that RNA polymerase reads to synthesize RNA. It is complementary to the mRNA and serves as a guide for RNA synthesis. - Non-template strand: the opposite strand, not directly involved in RNA synthesis but contains the same sequence as the mRNA. It mirrors the mRNA sequence but the template is used for transcription. ## Why is the non-template strand called the coding strand? - The coding strand's nucleotide sequence matches the sequence of the transcript produced during transcription, except that RNA has U in place of T. Its sequence will be the same as that of the mRNA. ## What direction is transcription? - Template strand read: read by RNA polymerase in the 3' to 5' direction during transcription. - Pre-mRNA strand assembled: assembled in the 5' to 3' direction as RNA polymerase adds nucleotides to the 3'-hydroxyl (-OH) end of the growing RNA chain. ## Transcription factors - Transcription factors: proteins that bind to specific DNA sequences near a gene's promoter to regulate transcription. - Essential for controlling gene expression, guiding cell differentiation, and responding to signals by activating or repressing specific genes. - Without them, RNA polymerase cannot efficiently initiate transcription. ## Pre-mRNA: - Pre-mRNA (precursor mRNA): the initial RNA transcript produced during transcription in eukaryotes. Contains exons (coding regions) and introns (non-coding regions), and serves as the unprocessed form of mRNA that undergoes further modifications. ## Process of Transcription in Eukaryotes - Introns and exons: - Exons are coding segments of a gene that remain in the final mRNA and determine the amino acid sequence of a protein. - Introns are non-coding sequences that are transcribed into pre-mRNA but removed during splicing, allowing exons to join and form mature mRNA. - RNA polymerase II: the enzyme responsible for synthesizing pre-mRNA by using a DNA template strand. It unwinds the DNA, reads the template strand (3' to 5' direction), and synthesizes RNA in the 5' to 3' direction. - Splicing: removes introns from pre-mRNA and connects exons to create mature mRNA. Carried out by spliceosomes (large complex of proteins and small nuclear ribonucleoproteins (snRNPs) that recognize specific splice sites and catalyze intron removal. - Initiation, elongation, and termination: - Initiation: transcription begins when transcription factors bind to the promoter area, recognizing specific sequences that serve as binding sites. - Elongation: RNA polymerase moves along the DNA strand, adding complementary RNA nucleotides (A, U, G, or C) to the growing RNA strand (elongates in the 5' to 3' direction). - Termination: transcription ends when RNA polymerase transcribes a termination signal, the pre-mRNA is released, and RNA polymerase dissociates from DNA. ## Transcription in Eukaryotes vs Prokaryotes - Location: - Eukaryotes: nucleus. - Prokaryotes: cytoplasm (no nucleus). - DNA is housed: - Eukaryotes: the nucleus. - Prokaryotes: cytoplasm. - Transcription and translation: - Eukaryotes: transcription and translation are separate processes. - Prokaryotes: transcription and translation occur simultaneously (coupled). ## Modification of mRNA - 5' Cap: a modified guanine is added to the 5' end of pre-mRNA. Functions protect from degradation and aids in ribosome binding during translation. - Poly(A) tail: a sequence of adenine (A) is added to the 3' end of pre-mRNA. Functions: stabilizes mRNA and eases export to the cytoplasm. - Splicing: introns are removed, exons joined. These changes ensure that mRNA is stable, properly processed, and ready for translation into a protein. ## Differences between transcription in pro and eukaryotes - Prokaryotes: - No introns - No nucleus - Transcription and translation are coupled - Polycistronic mRNA - Eukaryotes: - Has introns - Requires RNA processing - Transcription occurs in the nucleus, translation occurs in the cytoplasm - Monocistronic mRNA ## Differences between polycistronic and monocistronic transcripts: - Polycistronic: One mRNA codes for multiple proteins (common in prokaryotes). - Monocistronic: One mRNA codes for a single protein. ## Differences between DNA replication and transcription - Replication: the entire DNA molecule is copied to produce two identical strands. - Transcription: only specific genes are transcribed into RNA. It involves a single DNA strand. ## Translation in Eukaryotes - Translation: the process where mRNA is used to build proteins. - Initiation: - Ribosome binds to mRNA. - Finds the start codon (AUG). - Initiator tRNA carrying methionine binds to the start codon. - Large ribosomal subunit binds to smaller to form a complete ribosome. - Elongation: - Ribosome moves along mRNA, reading codons. - Adds correct amino acids to the growing protein chain. - Termination: - Reaches a stop codon. - Protein is released. - Ribosome disassembles. ## Importance of Codon - Codons: 3-nucleotide sequences on mRNA that specify which amino acid will be added. - They ensure accurate translation of genetic information into proteins. ## Importance of Start Codon - Signals the start of translation. - Specifies the amino acid methionine (Met). ## Importance of Stop Codon - UAA, UAG, UGA: signal the end of translation. - They don't encode amino acids but recruit release factors to terminate protein synthesis. ## Degenerate Codons - Genetic code is degenerate, meaning multiple codons can encode the same amino acid. - Provides a buffer against mutations. ## Segment of Nucleotides - If coding strand sequence is 5'-ATGCGTACG-3', template strand is 3'-TACGCATGC-5'. - If the coding strand is 5'-ATGCGTACG-3', mRNA strand sequence is 5'-AUGCGUACG-3'. ## Coding vs Non-coding & Template vs Non-template - Coding (non-template): has the same sequence as the mRNA (except T is replaced with U in RNA). - It's not directly used in transcription but carries the genetic code. - Non-coding (template): serves as the template for RNA synthesis. Used by RNA polymerase to produce a complementary RNA sequence. ## mRNA direction - mRNA is synthesized in the 5' to 3' direction by RNA polymerase. - RNA polymerase reads the DNA template strand in the 3' to 5' direction. ## Operons - Operons: clusters of genes in prokaryotes that are transcribed into a single mRNA. - They allow coordinated expression of genes that function in the same pathway. ## Regulatory gene - Regulatory gene: encodes for a protein or RNA that regulates the expression of structural genes (e.g., a repressor). ## Promoter - Promoter: DNA sequence where RNA polymerase binds to initiate transcription. ## Operator - Operator: DNA segment that acts as a switch, binding regulatory proteins like repressors. ## Repressor - Repressor: protein that binds to the operator to prevent transcription. ## Structural gene - Structural gene: encodes proteins or RNA for specific metabolic pathways. ## Inducible Operon - Inducible operon: normally 'off' but can be turned 'on' in response to a specific inducer. - Example: Lac operon - Lac operon is activated by lactose, which inactivates a repressor, allowing transcription of genes for lactose metabolism. ## Repressible Operon - Repressible operon: normally 'on' but can be turned 'off' when a specific corepressor is present. - Example: His operon, tryptophan operon. - Trp operon is repressed when tryptophan levels are high, as tryptophan acts as a corepressor, enabling the repressor protein to bind to the operator. ## Promoter - A specific DNA sequence located near the start of a gene that serves as the binding site for RNA polymerase and transcription factors to initiate transcription. It contains conserved elements that help guide the transcription machinery. - Recognized by transcription factors, which bind to it and recruit RNA polymerase to the correct site.