Biology Lecture Notes PDF
Document Details
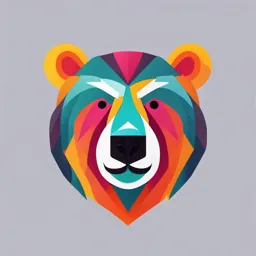
Uploaded by ModestSnail1253
Tags
Related
- Molecular Biology Lecture 1 PDF
- Molecular Cell Biology and Genetic Module (CBG 1103) PDF
- 2023 Molecular Biology and Diagnostics Main Module PDF
- Principles of Biology - An Introduction to Biological Concepts PDF
- Biology 1 – Cells, Molecular Biology and Genetics (Biol 1000) Past Paper PDF
- Basic Genetic & Molecular Biology - Cell Biology (PDML 4813)
Summary
These are biology lecture notes covering topics from DNA and RNA structure and function, through to cell division, meiosis and genetics. Key areas covered include protein synthesis, heredity, and the differences between mitosis and meiosis. There is a discussion of independent assortment and genetic variability.
Full Transcript
Lecture 17.2 What is a nucleic acid? Ribonucleotides: monomers of RNA, sugar is ribose, -OH group is bonded to the 2’ carbon. Deoxyribonucleotides: monomers of DNA, sugar is deoxyribose, H bonded at the 2’ carbon. Both of these sugars have an -OH group bonded to the 3’ carbon. What are the 2 groups...
Lecture 17.2 What is a nucleic acid? Ribonucleotides: monomers of RNA, sugar is ribose, -OH group is bonded to the 2’ carbon. Deoxyribonucleotides: monomers of DNA, sugar is deoxyribose, H bonded at the 2’ carbon. Both of these sugars have an -OH group bonded to the 3’ carbon. What are the 2 groups of nitrogenous bases? Purines: contain 9 atoms in their 2 rings, A&G Pyrimidines: contain 6 atoms in their 1 ring, C (U → found only in ribonucleotides, T → found only in deoxyribonucleotides).5 DNA and DNA strands are directional. Phosphodiester linkages form a sugar-phosphate backbone. Nucleic acids are directional (one end has an unlinked 5’ phosphate group, other end has an unlinked 3’ hydroxyl group) The order of nucleotides forms the primary structure. The sequence is written in a 5’ → 3’ direction, which reflects the order that nucleotides are added to a growing molecule..6 What is the nature of DNAs secondary structure? DNA has a sugar-phosphate backbone. → Chargaff established that the # of purines = # of pyrimidines and there are equal numbers of T’s & A’s, and equal numbers of C’s & G’s. X-ray crystallography predicted a helical structure. What did Watson and Crick determine? 2 strands are held together by hydrogen bonds between purines and pyrimidines, and complementary base pairing. Watson and Crick pairing occurs between A&T, C&G, 1. DNA strands ran in an antiparallel configuration 2. DNA strands form a double helix. → the hydrophilic sugar-phosphate faces the exterior. → nitrogenous base pairs face the interior..7 Complementary base pairing is based on hydrogen bonding. A) Only purine-pyrimidine pairs fit inside the double helix. B) Hydrogen bonds form between G-C pairs, and A-T pairs. C) In double stranded DNA, backbones must run in antiparallel directions..8 DNA strands form an antiparallel double helix. One turn of the helix occurs every 10 bases pairs, hydrophobic interactions in bases causes DNA to twist into a helix. Negatively charged phosphate groups face out, making DNA hydrophilic overall..9 The tertiary structure of DNA. DNA forms more compact 3D structures in cells. → when it is wound too tightly or loosely, it twists to form supercoils. → it wraps around proteins. Compacting DNA. → allows discrete units for cell division. → helps DNA fit inside the nucleus. → contribute to its function..10 DNA functions as an information molecule. DNA can store and transmit biological information. DNA carries the information required for the growth and reproduction of all cells. The language of the nucleic acids is contained in the sequence of the bases..11 DNA replication. DNAs primary structure serves as a template for the synthesis of a complementary strand. → contains the information required for a copy of itself to be made. → requires enzymes in modem cells. DNA replication has 3 steps: 1. The 2 strands are separated by breaking the hydrogen bonds with heat or enzymes. 2. Free deoxyribonucleotides hydrogen-bond complementary bases on the original strand of DNA, also called the template strand. → phosphodiester linkages form to create a new strand called the complementary strand. 3. Complementary base pairing allows each strand to be copied exactly. → produces 2 identical daughter molecules..12 DNA vs. RNA DNA RNA Very stable molecules which does not destroy, even on heating. Very reactive and unstable molecule. Stability due to presence of thymine. Unstable due to the presence of uracil..13 DNA vs. RNA structurally. RNAs secondary structure results form complementary base pairing: A&U, G&C. The bases of RNA typically form hydrogen bonds with complementary bases on the same strand. The RNA strands folds over, forming a hair pin structure: → the bases on one side of the fold align with an antiparallel RNA segment on the other side of the fold. Structurally RNA differs from DNA. RNA (like DNA) has a primary structure consisting of a sugar-phosphate backbone formed by phosphodiester linkages. → 4 types of nitrogenous bases extending from it. The primary structure of RNA differs from DNA in 2 ways. 1. RNA contains ribose instead of deoxyribose. →2’ -OH group on ribose is more reactive than -H. → RNA is much less stable than DNA. 2. RNA contains uracil instead of thymine..14 Tertiary Structure. RNA molecules can also have tertiary structure that forms when secondary structures fold into more complex shapes. RNA is much more diverse in size, shape, and reactivity than DNA (RNA is highly versatile). → information containing molecule. → capable of self-replication. → capable of catalyzing reactions: ribozymes. RNA is capable of self-replication. Information stored in RNA can be used to make copies via complementary base pairing. Must pass through double stranded intermediates. Processes occur today in some viruses. Lecture 18.2 The central dogma of molecular biology. Francis/Crick proposed that the sequence of bases in DNA acted as a code: → DNA is an information storage molecule. → the instructions it contained would somehow have to be read and then translated into proteins. Jacob and Monod suggested that RNA links genes in the nucleus to protein synthesis in the cytoplasm..3 Messenger RNA. Messenger RNA (mRNA) was found to carry information from DNA to the site of protein synthesis. The enzyme RNA polymerase synthesizes RNA. → uses a DNA strand as a template. → copies the code by matching complementary nucleotides. The central dogma of molecular biology summarizes the flow of information in cells. DNA codes for RNA, which codes for proteins: DNA → RNA → Proteins. Genes are stretches of DNA that code for proteins, the DNA sequence codes for the RNA sequence, the RNA sequence codes for the sequence of amino acids in a protein..4 Linking the central dogma to cellular processes. RNA synthesis transcription: the process of using a DNA template to make a complementary RNA. Protein synthesis translation: the process of using the information in mRNA to synthesize proteins. DNA transcription mRNA translation proteins. Information —> information carrier —> active cell machinery. storage.5 The genetic code. The genetic code specifies how a sequence of nucleotides codes for a sequence of amino acids. Gamow predicted that each “word” contains 3 bases. → a 3 base code is the least that could specify the 20 amino acids. A 3 base code is known as a triplet code. A group of 3 bases that specifies a particular amino acid is called a codon..6 The genetic code is: Redundant: all but 2 amino acids are encoded by more than 1 codon. Unambiguous: 1 codon never codes for more than 1 amino acid. Non-overlapping: codons are read 1 at a time. Nearly universal: all codons specify the same amino acids in all organisms (with few minor exceptions) Conservative: if several codons specify for the same amino acid, the first 2 bases are usually identical..7 The secondary structure of DNA. DNA has directionality → the 3’ end has an exposed hydroxyl group attached to the 3’ carbon of deoxyribose. → the 5’ end has an exposed phosphate group attached to a 5’ carbon..9 → because of complementary base pairing, existing strands of DNA can serve as a template for the production of new strands. 3 hypotheses for how parental strands and daughter strands act during replication. 1. Semiconservative replication: parental strands separate, and each is a template for a new strand. Each daughter has 1 old and 1 new strand. 2. Conservative replication: the parental molecule serves as a template for an entirely new molecules, 1 daughter has both old strands; the other has both new strands, 3. Dispersive replication: the parent molecule is cut into sections. Each daughter has old and new DNA interspersed..10 DNA polymerization should be endergonic. The monomers are deoxyribonucleoside triphosphates (dNTPs). → have high potential energy because of their 3 closet packed phosphate groups. → they have enough potential energy to make the formation of phosphodiester bonds exergonic..11 Where does replication start? A replication bubble forms when DNA is being synthesized. → forms at a specific sequence called the origin of replication (OOR). Bacteria have 1 and form 1 replication bubble. Eukaryotic cells have many on each chromosome. Each replication bubble has 2 replication forks. → because synthesis is bidirectional. → replication bubbles grow in 2 directions..13 How is the leading strand synthesized? The 3’ end for DNA polymerase to add to is supplied by a primer. → an RNA strand about a dozen nucleotides long. → base pairs with the DNA template strand. → provides a 3’ hydroxyl (OH) group that can combine with a dNTP to form a phosphodiester bond. Primers are made by an enzyme called primase. → a type of RNA polymerase. → does not require a free 3’ end to begin synthesis. DNA polymerase adds dNTPs to the primers 3’ end. Since DNA strands are antiparallel, the synthesis process differs for each strand. → the strand that is synthesized toward the replication fork is the leading strand or the continuous strand. → it is synthesized continuously in the 5’ → 3’ direction..17 Correcting mistakes in DNA synthesis. DNA polymerase matches bases with high accuracy. → correct bases are the most energetically favourable. → correct base pairs have a distinct shape. → inserts an incorrect base about once every 100,000 bases. Repair enzymes remove defective bases and replace them with the correct one. DNA can be damaged by sunlight, x-rays, and many chemicals. Organisms have DNA damage -repair systems. For example, UV light and some chemicals can cause thymine dimers to form. These dimers can produce a kink in the DNA strand. This blocks DNA replication. Lecture 19.2 The Cell Cycle. The cell cycle involves 4 phases: 1. G1 phase (first gap) 2. S phase (synthesis) 3. G2 phase (second gap) 4. M phase (mitosis) The time spent in gap phases allows the cell to grow and replicate organelles..3 Cells alternate between M phase and interphase. 1. A dividing phase called M (mitotic) phase: chromosomes are condensed into compact structures. 2. A non-dividing phase called interphase: chromosomes are uncoiled, cells are growing and preparing, or are fulfilling their specialised functions. Cells spend most of their time in interphase..4 S Phase. The stage in which DNA replication occurs is called the S phase. The cell cycle is the orderly sequence of events that occurs from the formations of eukaryotic cells, through the duplication of its chromosomes, to the time it undergoes cell division..5 The Gap phases. Interphase also includes 2 gap phases, during which no DNA synthesis occurs. G1 is the 1st gap: occurs between the M phase and the S phase. G2 is the 2nd gap: occurs between the S phase and mitosis. The gap phases allow cells to grow and replicate organelles..6 What happens during M Phase? M phase consists of 2 distinct events: 1. Mitosis → the divisions of the replicated chromosomes. 2. Cytokinesis → the division of the cytoplasm..7 M phase. Cells arise through division of pre-existing cells. Observations of newly developing organisms, or embryos, confirmed that plants and animals start life as single celled embryos, grow through a series of cell divisions, and that meiosis produces reproductive cells called gametes, mitosis produces all other cell types = somatic. Mitosis and meiosis are usually accompanied by cytokinesis. → division of the cytoplasm into 2 daughter cells. During mitosis, the genetic material is copied and divided equally between 2 cells → daughter cells are genetically identical to the parent cell and to each other. Meiosis produces cells with half the amount of hereditary material as the parent cell. → daughter cells are genetically different..10 Cancer: Out of control cell division. A complex family of disease caused by cells that grow in an uncontrolled fashion, invade nearby tissues, and spread to other sites in the body. Over 200 types of cancer all arise from cells in which cell-cycle checkpoints have failed. 2 types of defects: 1. Defects that activate the proteins required for cell growth when they should not be active. 2. Defects that prevent tumor suppressor genes from shutting down the cell cycle..11 Properties of cancer cells. 2 types of tumors: 1. Malignant tumors are cancerous and invasive: can spread throughout the body via the blood or lymph, and initiate secondary tumors called metastasis. 2. Benign tumors are noncancerous and non-invasive: many cancers are thought to arise from cells with defects in the G1 checkpoint. Lectures 20&21..2 An overview of transcription. Like DNA polymerases, RNA polymerases perform template directed synthesis in the 5’-5; direction. Unlike DNA polymerases, RNA polymerases don’t require a primer to begin transcription. → bacteria have 1 RNA polymerase. → Eukaryotes have at least 3 distinct types: RNA polymerase I, II, and III..3 Transcription. RNA polymerase synthesizes an mRNA version of the instructions stored in DNA. → uses RNA polymerase. → matches complementary bases to one strand of DNA. → RNA synthesized in the 5’-3’ direction. Only 1 strand of DNA is the template, called the template strand, the other strand is the non-template strand or the coding strand. Matches the sequence of mRNA (except U for T).6 Translation: Ribosomes are the site of protein synthesis. In translation, the sequence of bases in an mRNA is converted to an amino acid sequence in a protein. → Ribosomes catalyze translation of the mRNA sequence into protein..7 How does an mRNA codon specify an amino acid? 2 hypotheses regarding how amino acid sequence is coded by a sequence of nucleotides: 1. mRNA codons and amino acids interact directly. 2. Crick proposed that an adapter molecule holds amino acids in place while interacting with a codon. The adapter molecule was later found to be a small RNA called tRNA. → tRNAs bind to amino acids. → transfer them to the growing polypeptide..8 What do tRNAs look like? tRNAs are relatively long: 75-85 nucleotides long. They can form a secondary structure by folding into a stem and loop. → a CCA sequence at the 3’ end is the binding site for amino acids. Aminoacyl tRNA is a tRNA linked to its amino acid. The loop at the opposite end forms the anticodon. → has a sequence of 3 nucleotides. → can base-pair with the mRNA codon..11 The structure of ribosomes and their function in translation. 3 tRNAs are bound to the corresponding codon in the mRNA. The tRNAs fit into 3 sites in the ribosome: 1. The A is the acceptor site for an aminoacyl tRNA. 2. The P is the peptidyl site where a peptide bond forms. 3. The E is where tRNAs without amino acids exit the ribosome. The protein grows by one amino acid with each repeat of the 3 steps. Amino acids are always added to the carboxyl end (c-terminus) of the polypeptide. Translation has 3 phases: 1. Initiation: begins near the AUG start codon. The small ribosomal subunit binds to the mRNA. → At the ribosomal binding site about 6 bases from the start codon, mediated by initiation factors, the 1st tRNA is called the initiator tRNA, it carries modified methionine. 2. Elongation: the initiator tRNA is in the P site, the E&A sites are empty. An aminoacyl tRNA binds to the codon in the A site. The amino acid on the P-site tRNA is transferred to the amino acid on the A-site tRNA. 3. Termination: when the A-site encounters a stop codon, a protein called a release factor enters the A-site. →Resembles tRNAs in size and shape, but does not carry an amino acid. Hydrolyzes the bond linking the P-site tRNA to the polypeptide chain. The newly synthesized polypeptide, tRNAs, and ribosomal subunits separate from the mRNA. Lecture 22..2 Proteins needed for Mitosis: Cohesins: form rings that hold sister chromatids together. Microtubules: move chromosomes to the poles. Kinetochore Proteins: sites where microtubules connect to chromosomes. Nuclear Lamins: Intermediate fibers that form an interface between the chromosome and inside the nuclear envelope. Codesins: ring shaped and condense the DNA..2 To complete mitosis, a cell must have completed 3 tasks: In a replication, cell growth, and microtubule organizing center (MTOC) replication. Mitosis (M phase) is a continuous process with 5 subphases: 1. Prophase: chromosomes condense, first become visible in the light microscope. The spindle apparatus forms, produces mechanical forces that move replicated chromosomes during early mitosis, and pull chromatids apart in late mitosis. → The spindle apparatus is made of microtubules, formed from MTOCs, which define the 2 poles of the spindle apparatus, plus ends of microtubules growing out from each pole. Polar microtubules extend from each spindle pole and overlap with each other. → In animal cells, MTOCs are centrosomes, each containing a pair of centrioles. 2. Prometaphase: Nuclear envelope breaks down, microtubules attached to chromosomes at kinetochores. Structures that form at the centromere, 2 from an opposite side of each chromosome. Microtubules that attach to chromosomes are called kinetochore microtubules. chromosomes are pushed and pulled by microtubules until they reach the middle of the spindle. 3. Metaphase: Formation of the mitotic spindle is completed. chromosomes are aligned up on the metaphase plate; an imaginary plate between the two spindle poles. Each chromosome is held by kinetochore microtubules from opposite poles. Astral microtubules hold spindle poles in place. 4. Anaphase: cohesins holding sister chromatids together split, sister chromatids are pulled by the spindle fibers toward opposite poles of the cell, this creates 2 identical sets of daughter chromosomes. 2 Forces pull chromosomes apart → kinetochore microtubules shrink, motor proteins out the polar microtubules push the 2 poles of the cell away from each other. 5. Telophase: A new nuclear envelope begins to form around each set of chromosomes. The chromosomes begin to condense. mitosis is complete when 2 independent nuclei have formed. Cytokinesis typically occurs immediately after mitosis, the cytoplasm divides to form 2 daughter cells..8 Introduction to meiosis. During sexual reproduction, reproductive cells called gametes unite to form a new individual. This process is called fertilization. → Gametes are called sperm and egg in animals. Meiosis is a nuclear division that leads to halving of chromosome numbers. → Gametes must contain half the chromosome number. → At fertilization, the full chromosome number is restored. Every organism has a characteristic number of chromosomes. Sex chromosomes determine the sex of the individual. In many animals, females have 2 X chromosomes, and males have an X and Y chromosome. Autosomes are non sex chromosomes..10 The concept of ploidy. A karyotype identifies the number and types of chromosomes present in a species. Many organisms, including humans are diploid. → they have 2 homologs on each chromosome. → they have 2 alleles of each gene. Other organisms are haploid, meaning they only have 1 of each type of chromosome, and they have just 1 allele of each gene..12 An overview of meiosis. Just before meiosis begins, each chromosome in the diploid (2n) parent cell is replicated. When replication is complete, each chromosome has 2 identical sister chromatids. → they remain attached along most of their length. → the 2 attached sister chromatids are still considered a single replicated chromosome..13 Meiosis consists of 2 cell divisions. 1. Meiosis I → the homologs of each chromosome pair are separated into 2 daughter cells: each daughter cell has 1 set of chromosomes, a diploid parent produces 2 haploid daughter cells. 2. Meiosis II → the sister chromatid of each chromosome separate into 2 daughter cells: each of the 2 daughter cells from meiosis I divies, resulting in 4 haploid cells..16 The phases of meiosis I. Meiosis is a continuous process with 5 distinct phases: 1. Early prophase I: the nuclear envelope begins to break down, chromosomes condense, the spindle apparatus begins to form, the homologous pairs come together in a pairing process called synapsis, resulting in a bivalent structure. 2. Late Prophase I: the 2 homologs become attached to microtubules from opposite poles of the spindle apparatus. The homologs begin to separate. They remain attached at many points called chiasmata. Exchange or crossing over between homologous non-sister chromatids occurs, producing chromosomes with a combination of maternal and paternal alleles. 3. Metaphase I: the paired homologs line up at the metaphase plate. Alignment of the homologs is random. 4. Anaphase I: the paired homologs separate and migrate to opposite ends of the cell. 5. Telophase: the homologs finish migrating to the poles of the cell. Then the cells divide in the process of cytokinesis..20 Meiosis I: A recap. Meiosis I results in daughter cells with only 1 chromosome of each homologous pair. They are haploid, but still contain replicated chromosomes. They have a random assortment of maternal and paternal chromosomes due to crossing over, random distribution of maternal and paternal homologs to daughter cells, and random fertilization of sperm and eggs..21 The phases of Meiosis II. No chromosome replication occurs between meiosis I and II. Like meiosis I, meiosis II is a continuous process, but with 4 distinct phases: 1. Prophase II: the spindle apparatus forms, 1 spindle fibre attaches to the centromere of each sister chromatid. 2. Metaphase II: replicated chromosomes line up at the metaphase plate. 3. Anaphase II: sister chromatids separate, resulting daughter chromosomes begin moving to opposite sides of the cell. 4. Telophase II: chromosomes arrive at opposite sides of the cell, a nuclear envelope forms around each haploid set of chromosomes, each cell then undergoes cytokinesis..24 Mitosis vs. Meiosis The key diff. between the 2 processes → homologs pair in meiosis, but not mitosis, because homologs pair in prophase I, they separate during anaphase I, resulting in a reduction division Mitosis produces 2 diploid daughter cells that are genetically identical to parent cells. Meiosis produces 4 haploid daughter cells that are genetically distant from each other and front the parent cell..27 The role of independent assortment and crossing over. The separation of homologous chromosomes during meiosis I can result in a variety of combinations of maternal and paternal chromosomes called the principle of independent assortment. Crossing over produces new combinations of alleles on the same chromosomes, combinations that did not exist in each parent. Genetic recombinations from crossing over and from independent assortment increase the genetic variability of gametes produced by meiosis, beyond that produced by random assortment of chromosomes. Lecture 23..4 How did Mendel control mating? Peas normally self-fertilize ( self-pollinate). Male organs produce pollen grains, which makes sperm. Female organs produce eggs. A flower's pollen falls on the female organ of that same flower. Mendel could prevent self pollination by removing the male organs from a flower; he used pollen from one flower to fertilize another, this is called a cross, or cross pollination..5 What traits did Mendel study? Mendel worked with pea varieties that differed in 7 easily recognizable traits: seed shape, seed color, pod shape, pod color, flower color, flower pot and position, and stem length. An individual's observable traits comprise its phenotype. Mendel's pollution had 2 distinct phenotypes for each of the 7 traits. Pea plants produce offspring identical to themselves when self-fertilized. He used these plants to create hybrids. → He mated 2 different pure lines that differed in 1 or more traits. Mendel's first experiments involved crossing pure lines that differed in just 1 trait. Mating parents with two different phenotypes for a single trait is called a monohybrid cross. → The adults in the cross were the P generation, The offspring were the F1 generation (first filial)..9 The monohybrid cross When Mendel crossed plants with round seeds and plants with wrinkled seeds, all F1 Offspring had round seeds. This contradicted the hypothesis for bleeding inheritance. The genetic determinant for wrinkled seeds seemed to have disappeared. Mendel allowed the F1 progeny to self-pollinate. The wrinkled seed trait reappeared in the F2 Generation in a 3:1 ratio (round:wrinkled)..10 Dominant and recessive traits. Mendel called the genetic determinant for wrinkled seeds recessive, and the determinant for round seeds dominant. Mendel repeated these experiments with each of the other traits. In each case, the dominant trait was present in a 3:1 ratio over the recessive trait in the F2 generation. Lecture 24.4 Predicting offspring genotypes and phenotypes with a Punnett square. 1. Write each unique gamete genotype for 1 parent along the top of the diagram. 2. Write each unique gamete genotype for the other parent down the left side of the diagram. 3. Fill each box with the gamete genotypes above and to the left of that box. 4. Calculate the proportions or rates of each offspring genotype and phenotype..9 Meiosis explains Mendel's principles. The genes for different traits assort independently of one another at meiosis I because they are located at different non-homologous chromosomes, they assort independently of one another. This phenomenon explains Mendel's principle of independent assortment..15 The rule of crossing over. Morgan proposed that gametes with new, recombinant genotypes, were generated when crossing over occurred during prophase of meiosis I. Linked genes are inherited together unless crossing over occurs. When crossing over takes place, genetic recombination occurs. Genes are more likely to cross over when they are far apart from each other. The percentage of recombination offspring can be used to estimate the relative distance between genes. Frequency of crossing over can be used to create a genetic map. → a diagram showing relative proportions at genes, along a particular chromosome. Lecture 25..2 How many alleles can a gene have? Mendel worked with genes that have 2 alleles. When there are more than two alleles of a gene in a population, it is called a multiple allelism. →May have dozens of alleles of a single gene. Ex, humans have 3 common alleles for ABO blood types. IA, IB, i → Each codes for a version of an enzyme that adds polysaccharides to membranes of red blood cells..3 Codominance and incomplete dominance Alleles of a gene are not always dominant or recessive. Some alleles display codominance. → Neither allele is dominant or recessive to the other. → Heterozygotes display the phenotype of both alleles. Ex, ABO blood types. → both IA & I B are dominant to I, yet IA & I B heterozygotes produce both polysaccharides resulting in the AB blood type. Other alleles display incomplete dominance. → Heterozygotes have an intermediate phenotype. For example, pureline plants with red flowers (R+R+) crossed to pureline plants with white flowers (R-R-). Mendel would predict heterozygous offspring with red flowers (R+R-), however, R+R- offspring have pink flowers..12 Pedigrees can differentiate autosomal recessive and autosomal dominant traits. If a phenotype is due to an autosomal recessive allele, individuals with the trait must be homozygous. Unaffected parents of an unaffected individual are likely to be heterozygous for that trait. Carriers are heterozygous individuals who do not have a disease but carry a recessive allele for it. A recessive phenotype shows up in offspring only once both parents have that recessive allele. For example, sickle cell disease. Characteristics: Males and females are equally likely to be affected. Affected offspring often have unaffected parents. Unaffected parents of affected offspring are heterozygous (carriers) Affected offspring are homozygous. If both parents are heterozygous, about a ¼ of the offspring will be affected. Traits often skip generations..13 Autosomal dominant traits. If a trait is autosomal dominant, homozygous and heterozygous individuals will display the trait. →one heterozygous parent will pass it on to about 50% of his or her offspring. → any child with the trait must have a parent with the trait. For example, Huntington disease. “BB” if all children have the trait. “Bb” if few children have the trait. Characteristics: Males and females are equally likely to be affected. Affected offspring have at least 1 affected parent. Affected offspring are heterozygous if only one parent is affected. Unaffected offspring are homozygous recessive. If one parent is heterozygous, about ½ of the offspring will be affected. Trait does not skip generations..15 X-linked recessive traits. X-linked recessive traits are common. Men (XY) will exhibit the trait if they inherited from their mothers. Women (XX) will exhibit the trait if they are homozygous. Usually skips a generation → An unaffected male can pass it only to his daughters, daughters will pass the allele to about half their sons. Ex, red-green color blindness. Characteristics: Males are affected more frequently than females. Trait is never passed from father to son. Affected sons are usually born to carrier mothers. About ½ of the sons of a carrier mother will be affected. All daughters of an affected male and an unaffected non-carrier female are carriers. Trait often skips generations..16 X-linked dominant traits. X-linked dominant traits are rare. → an unaffected male passes the trait to all his daughters but none of his sons, daughters receive his only X chromosome. a female carrier will pass the trait to half her daughters and half her sons. → both genders receive one of her ex chromosomes. Ex, vitamin D resistant rickets (hypophosphatemia). Except for maleness, there are no known human Y-linked traits. Characteristics: Male and female are equally likely to be affected. All daughters of an affected father are affected, but no sons. Affected sons always have affected mothers. About ½ of the offspring of an affected mother will be affected. Affected daughters can have an affected mother or father. Trait does not skip generations.