BIO 305 Ch 8 Neurons PPT.pptx
Document Details
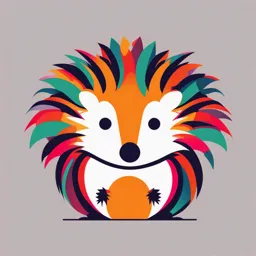
Uploaded by BrainyBlueLaceAgate
Tags
Related
- 4BBY1030 Cell Biology and Neuroscience - Organisation of the Nervous System PDF
- Neuroscience 1 - Introduction to the Nervous System PDF
- Neuroscience 1 - Introduction to the Nervous System PDF
- Fourth Year Nervous System Lecture 1 PDF
- Class 3: The Nervous System Part 2 PDF
- Anatomy of the Nervous System PDF
Full Transcript
Chapter 8 Neurons: Cellular and Network Properties Outline: I. Questions We Want Answered II. Organization of the Nervous System III. Cells of the Nervous System IV. Electrical Signals In Neurons V. Cell-to-Cell Communication in the Nervous System VI. Integration of Neural Information Transf...
Chapter 8 Neurons: Cellular and Network Properties Outline: I. Questions We Want Answered II. Organization of the Nervous System III. Cells of the Nervous System IV. Electrical Signals In Neurons V. Cell-to-Cell Communication in the Nervous System VI. Integration of Neural Information Transfer I. Questions We Want Answered What is ‘nervous tissue’? Where is it in the body, and What is it made of? What exactly is the ‘signal’ sent by neurons? How does ‘disequilibrium’ contribute to the ability of a neuron to function? II. Organization of the Nervous System Nervous Tissue – Neurons with processes secrete neurotransmitters – Glial cells = the glue ▪ Together form the ‘tissue’ = Brain/SC vs. Nerves Central nervous system = Brain and Spinal cord Peripheral nervous system = Nerves – Sensory (afferent) neurons – Efferent neurons: somatic motor and autonomic divisions Input / afferent / sensory SENSORY – MOTOR Processing Integration / decision making – All in = Sensory – All out = Motor Output / efferent / motor The Organization of the Nervous System: Peripheral Divisions Divisions of the Nervous System Central Nervous System (CNS) (brain and spinal cord) Information processing integrates, processes, and coordinates sensory input and motor commands. Peripheral Nervous System (PNS) Sensory information Motor commands within (nervous tissue within efferent division outside the CNS afferent division and the ENS) Somatic nervous Autonomic system (SNS) nervous system (ANS) Parasympathetic Sympathetic division division Smooth muscle Special sensory Visceral sensory Somatic sensory receptors Cardiac receptors receptors monitor smell, monitor internal monitor skeletal muscle taste, vision, organs muscles, joints, Glands balance, and and skin surface Skeletal Adipose hearing muscle tissue Receptors Effectors Not from your textbook! Where are these on your body??7 III. Cells of the Nervous System Neurons carry electrical signals – Functional unit of the nervous system Glial Cells provide support and structure – 2 in peripheral (nerves), and 4 in the CNS – Account for MOST of the cells in the nervous system – Outnumber neurons 5:1 Figure 8.2(f) Neuron Anatomy:(f) Parts of a Neuron The cell body (soma) is the control center Dendrites receive incoming signals Axons carry outgoing signals Figure 8.2 Neuron Anatomy Multipolar efferent neuron Figure 8.2(a)-(e) Neuron Anatomy: Functional Categories Axonal Transport Neurons are LONG and transport materials – Anterograde transport: from cell body to axon terminal – Retrograde transport: from axon terminal to cell body Neural Fatigue = cant send signal; no more neurotransmitter Synapses A synapse is the region where an axonal terminal meets its target cell – Presynaptic cell vs. postsynaptic cell – Synaptic cleft Chemical synapses and signals vs. electrical synapses Not fixed for life, can be rearranged Review: Neuron Anatomy Why do we call neuron axons ‘fibers’? List the parts of a neuron and their function. How does a neuron ‘integrate’ information? Where, and how, do neurons communicate? Where would you find most interneurons? Multipolar neurons? Uni / Pseudo-unipolar neurons? Where are the neurons?? What are all of these other cells?? Glial Cells Provide Support for Neurons Schwann cells (PNS) and oligodendrocytes (CNS) – Wrap around axon and form insulating myelin sheaths ▪ Nodes of Ranvier are gaps in insulation Satellite cells (PNS) – around nuclei of neurons – Ganglion outside of CNS vs. nucleus inside CNS Astrocytes (CNS) - several subtypes, multiple roles Microglia (CNS) - specialized immune cells Ependymal cells - one source of neural stem cells Neuroglia (glia L, glue) ~100 Billion neuroglial cells Outnumber neurons 5:1 Cellular Organization: Neuroglia of the CNS Why don’t we see any Schwann cells? Figure 8.5 (c) Schwann cell forms myelin around a small segment of one axon (d) Myelin Formation in the Peripheral Nervous System Figure 12–6b Schwann Cells, Peripheral Axons, and Formation of the Myelin Sheath in the PNS (Part 1 of 2). Un-Myelinated Axon = Schwann cell binds the axons of many neurons into a bundle = NOT myelinated!! Schwann Schwann cell #1 cell Schwann Schwann cell #2 cell nucleus Neurolemma Axons Schwann cell #3 nucleus Axons b The enclosing of a group of unmyelinated axons by a single Schwann cell. A series of Schwann cells is required to cover the axons along their entire length. 21 Can Stem Cells Repair Damaged Neurons? If the cell body dies, the neuron dies If axon is severed, then cell body and attached segment survives; severed portion degenerates – If motor neuron, then target muscle results in permanent paralysis – If sensory neuron, then experience loss of sensation from innervated area Regeneration may occur in PNS; less likely in CNS Figure 8.6 Periphery neuron injury Neurogenesis What is neurogenesis? Where is neurogenesis occurring in the human nervous system? – We now know that while most cells in the brain are born during embryonic and early postnatal development, new neurons are generated throughout life and are added to at least two areas of the brain: the hippocampus1 (which is involved in certain types of learning and memory) and the olfactory bulb What behavioral activities can alter neurogenesis? – Learning new tasks (language, drawing), exercising – Sometimes in response to a Stroke (CVA) What impairs Neurogenesis? Stress!!!!! http://www.bioedonline.org/hot-topics/adult-neurogenesis.cfm How to study….. Step 1: Chill Out IV. Electrical Signals in Neurons Recall that… – Neurons are ‘Excitable’ tissue – Able to send ‘electric’ signals – In physiology, the signal is generated by ions Membrane potential is influenced by – Concentration gradient of ions – Membrane permeability to those ions The GHK Equation Predicts Membrane Potential Using Multiple Ions… Goldman-Hodgkin-Katz equation calculates the membrane potential that results from the contribution of all ions that can cross the membrane Don’t need to know this … But notice the ions in the equation!!! Table 8.2 Ion Concentrations and Equilibrium Potentials Do NOT need to know absolute values - Diagram Not from your textbook! Membrane Potential All plasma (cell) membranes produce electrical signals by ion movements – Membrane potential is particularly important to muscle fibers and neurons – Required to cause ions to move – Three types: A. Resting membrane potential –The membrane potential of a resting cell B. Graded potential –Temporary, localized change in resting potential –Caused by a stimulus C. Action potential –Is an electrical impulse, produced by a graded potential –Propagates along surface of axon to synapse 29 Ion Movement Creates Electrical Signals Resting membrane potential determined by – Primarily K+ concentration gradient – Cell’s resting permeability to K+, Na+, and Cl– Changes in a membrane’s permeability result in ion movement – Movement creates an electrical signal – Very few ions move to create large changes in membrane potentials Review: Establishing the Resting Potential All cells have higher [K+] AND [A-] inside ECF always has a higher [Na+] and [Cl-] Chemical driving forces: – K+ out – Na+ in Not from your textbook! Figure 7.8a Review: Establishing the Resting Potential Leak channels = always open Membrane typically more permeable to K+ – More leak channels for K+ – More K+ leaves cell than Na+ enters Inside of cell becomes negative – because I ‘take away’ more positive from inside! Not from your textbook! Figure 7.8b Review: Establishing the Resting Potential Electrical forces develop – Na+ into cell – K+ into cell Due to electrical forces – K+ outflow slows – Na+ inflow speeds Electrochemical Gradients – Combination of CHEMICAL forces + ELECTRICAL forces Not from your textbook! Figure 7.8c Review: Establishing the Resting Potential Steady state develops – Inflow of Na+ is balanced by outflow of K+ Resting membrane potential = –70mV – Closer to EK because more ion channels for K Not from your textbook! Figure 7.8d Maintaining the Resting Potential: Neuron Condition would lead to both gradually reaching equilibrium as ions continued free movement Sodium / Potassium pumps maintains the resting potential Sodium pump returns Na+ & K+ to maintain gradients ▪2 K+ in, 3 Na+ out Not from your textbook! Figure 7.8e Graph of membrane potential changes Potential as measured in ONE SPOT along cell membrane: Both result from very small amount of ions… Gated Channels and Ion Permeability Ion channels are named for the primary ion that passes through them Gated channels control ion permeability – Mechanically gated, chemically gated, voltage- gated ion channels Threshold voltage varies from one channel type to another – Activation rates vary – Inactivation rates vary Figure 12–10a Types of Gated Ion Channels. b Voltage-gated ion channel c Mechanically gated ion channel a Chemically gated ion channel Activation gate Na + + ACh binding + –70 mV + site + + + + + + ECF Plasma membrane Cytosol Inactivation Gated Channel closed gate channel Channel closed Channel closed Binding of ACh –60 mV + + + + Applied + + pressure ACh + + + + + + Channel open + + + Channel open Channel open +30 mV + + Pressure + + + removed + + + Channel inactivated Channel closed A voltage-gated Na+ channel that Channel closed responds to changes in the membrane A chemically gated (ligand-gated) potential. At its resting membrane A mechanically gated ion channel, Na+ channel that opens in potential of –70 mV, the channel is which opens in response to response to the presence of ACh closed; at –60 mV, the channel opens; distortion of the membrane. (ligand) at a binding site. at +30 mV, the channel is inactivated. 38 Two Basic Types of Electrical Signals Graded Potentials – Variable strength – Used for short distance communication – Primarily ligand-gated ion channels Action Potentials – Very brief, large depolarizations – Rapid signaling over long distances – Primarily voltage-gated ion channels Graded Potentials Local current flow is a wave of depolarization that moves through the cell Graded potentials lose strength as they move through the cell due to – current leak – cytoplasmic resistance If strong enough, graded potentials reach the trigger zone in the axon hillock and initial segment – Can be Excitatory OR Inhibitory Figure 8.7(a) Graded potentials Figure 12–11 Graded Potentials (Part 1 of 3). 42 Figure 8.7(b)-(c) Graded potentials NO Action Potential! Figure 8.7(b)-(c) Graded potentials Action Potential! Purpose of Graded Potentials Determine whether or not an action potential will occur What changes at the axon hillock / trigger zone? What ‘triggers’ an action potential? Action Potentials = Electric impulse Conduction is the high-speed movement of a action potential along an axon. All-or-none Wave of electrical signal at constant amplitude Generation of an Action Potential Wave of current generated by constant ion flow Figure 8.8 Conduction of an action potential Na+ and K+ Move across the Membrane during Action Potentials Action potential begins when graded potential reaching trigger zone depolarizes to threshold Rising phase of the action potential – Voltage-gated Na+ channels open, Na+ in depolarizes cell Falling phase of the action potential – At peak, Na+ channels close, slower voltage-gated K+ channels open – K+ out repolarizes then hyperpolarizes cell – Voltage-gated K+ channels close, less K+ leaks out of cell – Cell returns to resting membrane potential of -70 mV Figure 8.9 The action potential Know this sequence of events! Figure 8.9 The action potential Axonal Na+ Channels Have Two Gates Activation vs. inactivation gates Action potentials will not fire during the absolute refractory period – The refractory period prevents backward conduction ▪ Potential delay of 1-2 msec between action potentials independent of intensity of trigger Absolute refractory period is due to voltage-gated Na+ channels resetting – Relative refractory period follows an absolute refractory period Limits the rate at which signals can be transmitted Figure 8.10 The voltage-gated Na+ channel Potential as measured in ONE SPOT along cell membrane: Figure 8.14 Conduction of action potentials Figure 8.14 Conduction of action potentials Action Potential – Speed of conduction Axon diameter affects propagation speed of action potentials – The larger the diameter, the lower the resistance and faster the speed Types of axons based on diameter, myelination, and propagation speed – Type A fibers – Myelinated - Large diameter - (120 m/sec) – Type B fibers – Myelinated - Medium diameter - (18 m/sec) – Type C fibers – Unmyelinated - Small diameter - (1 m/sec) Not Responsible for Name (Type A) or speed. Just concept: Larger + Myelin = Faster 57 Figure 8.16 Saltatory conduction Demyelination Disorders Heavy Metal Poisoning Bacterial toxins – Diptheria Multiple Sclerosis fatty myelin sheaths around the axons of the brain and spinal cord are damaged Guillain–Barré syndrome-demyelinating polyneuropathy (AIDP), a disorder affecting the peripheral nervous system. Ascending paralysis, weakness beginning in the feet and hands and migrating towards the trunk Chemical Factors Alter Electrical Activity Variety of chemicals alter the conduction of action potentials by binding to the channel Alterations in ECF concentration of ions also affects effects electrical activity – Ca2+ and K+ – Hyperkalemia brings neuron closer to threshold – Hypokalemia moves neuron further from threshold Review: Graded and Action Potentials What is a graded potential? – Where do they occur? – What is the function of a graded potential? What is the key difference between ion channels for graded and action potentials? Where do action potentials occur? – What is their purpose? – Describe why we say an AP is ‘All-or-None’. What is the purpose of myelin? – What would happen to muscle activity if all of your motor neurons lost myelin? V. Cell-to-Cell Communication in the Nervous System Neurons communicate at synapses – Electrical synapses – less common ▪ Pass electrical signals through gap junctions – Chemical synapses ▪ Use neurotransmitters that cross synaptic clefts Neurotransmitters bind to specific receptors – Ionotropic receptors are also called receptor-channels – Metabotropic receptors are G protein-coupled receptors for neuromodulators Acetylcholine (ACh) Binds cholinergic receptors – Nicotinic receptors ▪ Ionotropic – Ion channels: Na+ and K+ ▪ On skeletal muscles and in autonomic division of PNS and CNS – Muscarinic receptors ▪ Metabotropic – G protein–coupled receptors ▪ In CNS and on target cells for autonomic parasympathetic division of PNS Amines Adrenergic/noradrenergic neurons secrete norepinephrine – Adrenal medulla secretes epinephrine Adrenergic receptors – bind norepinephrine & epinephrine – G protein–coupled receptors – a and b classes Figure 8.18 A chemical synapse Figure 8.19(a) Synaptic Communication: Neurotransmitter Release Figure 8.19(b) Synaptic Communication: Neurotransmitter Termination Figure 8.20 Synthesis and recycling of acetylcholine Stronger Stimuli = MORE Neurotransmitter A single action potential results in the release of a constant amount of neurotransmitter Action potentials code duration and magnitude in the frequency of action potentials produced – Tonic activity – Burst activity Neurons can send 100-200 AP’s / second!! Figure 8.21 Coding the strength of a stimulus Review: Neurocrines, Receptors, Synapse What is the function of having the SAME neurocrine bind to MULTIPLE receptors? What is a synapse? Where are they? What triggers the release of a neurotransmitter? – Why does a neurotransmitter move across the synapse? – What can a neurotransmitter do on a post-synaptic membrane? – What happens to the neurotransmitter after it binds to a receptor? VI. Integration of Neural Information Divergence vs. convergence Synaptic plasticity – A change of activity at the synapses – Occurs primarily in CNS – May be short-term or long-term – May be enhance or reduce synaptic activity Figure 8.22(a)-(b) Divergence and Convergence 1 to many Many to 1 Multiplier Effect Thalamus Sympathetic NS – ‘fight or flight’ Or Diaphragm Figure 8.22 (c) Synapses on a Cell Body, (d) Purkinje Cells ~85 Billion neurons ~200,000 synapses EACH Postsynaptic Responses Slow synaptic potentials involve G-protein coupled receptors and second messengers. Fast synaptic potentials involve opening of ion channels. – An excitatory postsynaptic potential (EPSP) is depolarizing. – An inhibitory postsynaptic potential (IPSP) is hyperpolarizing. Figure 8.23 Fast and Slow Postsynaptic Responses Pathways Integrate Information from Multiple Neurons Spatial summation – Two or more neurons simultaneously fire and have an additive effect – Postsynaptic inhibition ▪ Release of neurotransmitter is inhibitory instead of excitatory Temporal summation – Summation occurring when graded potentials overlap in time and having an additive effect Synaptic activity can be modified – Modulatory neuron terminates on the presynaptic cell and modulates the release of neurotransmitter – Presynaptic facilitation favors the release of neurotransmitter – Presynaptic inhibition prevents the release of neurotransmitter Figure 8.24(a)-(b) Integration of synaptic Signaling Figure 8.24(c)-(d) Integration of synaptic Signaling Figure 8.24(e) Integration of synaptic Signaling Summation: Cancelling Effects Figure 7.13d Figure 8.24(f)-(g) Integration of synaptic Don’t need to know details Long-Term Potentiation (LTP) Alters Synapses Activity at a synapse induces sustained changes in quality or quantity of connections. – Potentiation similar to facilitation – Depression similar to inhibition Probably be responsible for acquired behaviors Glutamate is key element in potentiation. – AMPA receptors and NMDA receptors Disorders of synaptic transmission are responsible for many diseases – Parkinson’s disease, schizophrenia, and some depressions Figure 8.25 Long-term potentiation This is how your brain learns new things. Why do you think its so ‘hard’ to learn new things??? Review: Neural Integration Do all signals across a synapse cause an action potential on the post-synaptic membrane? How could you inhibit a neuron from generating an action potential? Can you teach an old dog a new trick?