Chapter 17: Muscular System PDF
Document Details
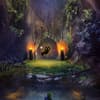
Uploaded by iiScholar
Arizona State University
Tags
Related
- Anatomy and Physiology: Muscular System PDF
- Essentials of Human Anatomy & Physiology PDF
- Essentials of Human Anatomy & Physiology Chapter 6 PDF
- Muscular System Anatomy and Physiology
- Anatomy & Physiology - Muscular System PDF
- Essentials of Human Anatomy and Physiology (2012) Chapter 6 - Muscular System PowerPoint Lecture Slides PDF
Summary
This chapter provides an overview of the muscular system. It details general muscle characteristics, focusing on the interaction of actin and myosin proteins during muscle contractions. The chapter also explores the differences between striated and smooth muscles, along with their structures and functions.
Full Transcript
Automatic ZoomActual SizePage Width100%50%75%100%125%150%200%300%400% Chapter 17: Muscular System 556 Lesson 17.1 **General Muscle Characteristics** Introduction **Muscles** are tissues composed primarily of cells specialized to produce force through the interaction of actin and myosin protein...
Automatic ZoomActual SizePage Width100%50%75%100%125%150%200%300%400% Chapter 17: Muscular System 556 Lesson 17.1 **General Muscle Characteristics** Introduction **Muscles** are tissues composed primarily of cells specialized to produce force through the interaction of actin and myosin proteins. An instance of muscle force production is called a **contraction**. Contraction requires the pumping of ions and powering of the motor protein myosin, both of which increase the energetic requirements of muscle cells. Based on microscopic structural organization, muscle cells (ie, **myocytes**) can be described as striated (ie, cardiac and skeletal muscle) or smooth (Figure 17.1). **Figure 17.1** Types of muscle cells. 17.1.01 Myocytes **Myocytes** are the predominant and defining cell type of muscles. Although myocyte structure varies according to the type of muscle in which myocytes are found, all myocytes share a few notable properties. First, all myocytes are specialized for force production through the interaction of the proteins actin and myosin. Second, force production by myocytes is dependent on elevation of intracellular calcium ion (Ca2+) concentration. Finally, like most [eukaryotic cells](javascript:void(0)), myocytes are nucleated, although the precise number of nuclei per myocyte varies. Certain structures common to eukaryotic cells are sometimes referred to by different names when discussing myocytes (which are often called fibers). For example, the plasma membrane of muscle cells is often called the **sarcolemma**, and muscle cells have a specialized type of endoplasmic reticulum called the **sarcoplasmic reticulum**. Myocyte cytoplasm is sometimes called **sarcoplasm**. Like the cytoplasm of other cells, sarcoplasm has a very low Ca2+ concentration at rest. The concentration of Ca2+ is much higher in the sarcoplasmic reticulum and in the extracellular fluid surrounding each myocyte (Figure 17.2). Chapter 17: Muscular System 557 **Figure 17.2** A portion of a striated myocyte. 17.1.02 Myocyte Structure and Function Relationships All muscles produce force through the interaction of **actin** and **myosin** protein molecules. In muscle, actin molecules are linked together with other actin molecules to form filaments of actin; similarly, myosin molecules are linked together with other myosin molecules to form filaments of myosin. Based on their relative thicknesses, actin filaments are also referred to as **thin filaments**, and myosin filaments are referred to as **thick filaments**. Myosin filaments contain a rod-like central region, composed of myosin tails, as well as heads that extend out from the rod-like region. Connecting the myosin heads to the myosin tails in the central region are thin arms, or hinges (Figure 17.3).  Chapter 17: Muscular System 558 **Figure 17.3** Myosin molecules in a thick filament. Actin and myosin filaments are not organized in the same way in all muscles. In **striated** muscles, actin and myosin filaments are arranged parallel to one another in a way that produces regions of overlapping actin and myosin next to regions without overlap. These two types of regions regularly repeat, with actin filaments aligned with neighboring actin filaments and myosin filaments aligned with neighboring myosin filaments. When viewed under a microscope, this arrangement results in regularly spaced lighter and darker bands called **striations** (Figure 17.4). In other muscles, actin and myosin filaments are organized differently and lack striations. Because these nonstriated muscles appear relatively homogeneous when viewed under a microscope, they are known as **smooth** muscles (Figure 17.4). Striated muscle cells are long cylindrical fibers, whereas smooth muscle cells, or fibers, are often described as spindle-shaped (ie, wide in the middle and tapered at the ends). **Figure 17.4** Striated versus smooth muscle. During muscle contraction, myosin and actin filaments interact with one another (Figure 17.5). Specifically, myosin heads [bind to](javascript:void(0)) and pull upon actin filaments. This pulling generates force along the myosin and actin filaments, and if this force exceeds any opposing forces, the actin filaments slide along the myosin filaments. This sliding movement shortens the muscle cell along the length of the interacting thick and thin filaments. However, the length of each actin and myosin filament remains *unchanged*. Instead, it is the sliding of the two filament types along one another that causes the shortening, as shown in Figure 17.5. Striated muscle force production is greatest when actin-myosin overlap is maximal. A close-up of a human body Description automatically generated  Chapter 17: Muscular System 559 **Figure 17.5** Arrangement of actin and myosin filaments during relaxation and contraction of striated muscle. The banding pattern of striated myocytes is related to the presence of multiple functional units along the length of **myofibrils**, which are tiny cylindrical bundles of interacting thick and thin filaments surrounded by sarcoplasmic reticulum within a myocyte. These functional contractile units are called **sarcomeres** and are arranged in series along the length of a striated muscle cell. Each sarcomere has thick filaments centered within it. Myosin heads extend out from most of the thick filaments but are absent in the middle of the sarcomere. Actin filaments extend from each end of the sarcomere but do not reach the middle. As a result of this configuration, sarcomeres contain several regions (Figure 17.6): The **I band** at each end of a sarcomere consists of only actin (thin) filaments. The **H band** in the center of the sarcomere consists of only myosin (thick) filaments. The **A band** fully encompasses the H band and extends further to include the regions of actin and myosin overlap; therefore, the A band marks the length of the myosin filaments. The **M line** marks the center of each sarcomere. The **Z line** is the boundary between neighboring sarcomeres. A large, elastic protein called **titin** is bound to the Z line and helps maintain the ordered arrangement of actin and myosin filaments within the sarcomere. Chapter 17: Muscular System 560 **Figure 17.6** Components of a sarcomere. In contrast to striated muscle cells, smooth muscle cells lack sarcomeres, and thick filaments in smooth muscle cells have myosin heads along their entire length. This allows optimal actin-myosin interaction (ie, optimal force production) in smooth muscle cells over a much greater length than in striated muscle cells. In addition, smooth muscle thick and thin filaments are not aligned in parallel with their respective neighboring filaments but are oriented in multiple directions. Structural differences between striated and smooth muscle cells lead to important functional differences. Relative to smooth muscle cells, striated myocytes are specialized for relatively rapid, intermittent contractions (eg, beating of the heart or lifting of an object). Smooth muscle cells are specialized for slower and more sustained contractions (eg, constriction of a blood vessel or contraction of an intestinal segment, Figure 17.7). Smooth muscle contractions are much more energetically efficient than striated muscle contractions, as discussed later in this concept.  Chapter 17: Muscular System 561 **Figure 17.7** Relative rates of force development in skeletal, cardiac, and smooth muscle. An energetic cost is associated with muscle contraction, and different strategies exist to match energetic supply and demand in striated and smooth muscles. The energetic costs of muscle contraction are primarily due to the movement of actin filaments by myosin and the maintenance of ionic gradients. [ATP hydrolysis](javascript:void(0)) by myosin ATPases alters the position of myosin heads, correctly positioning them for the force-generating interaction between actin and myosin during the power stroke of the cross-bridge cycle (described in more detail in Concept 17.1.04). Movement of ions across cellular membranes is necessary for muscle activation, force development, and relaxation. ATP-requiring pumps such as the plasma membrane [Na+/K+-ATPase](javascript:void(0)) and the sarcoplasmic reticulum Ca2+-ATPase (SERCA) use energy released during ATP hydrolysis to pump ions against their concentration gradients. In striated muscles, more active, fatigue-resistant muscle cells exhibit more capillaries and greater concentrations of O2-binding myoglobin and mitochondria. These characteristics reflect an increased reliance upon aerobic energy production to meet the energetic demands of repetitive muscle contraction. [Like hemoglobin](javascript:void(0)), myoglobin is a heme-containing protein that imparts a reddish color to cells in accordance with its concentration. The heme in myoglobin binds and releases oxygen according to cellular concentrations of oxygen and certain other molecules, thereby acting to stabilize oxygen supply for respiration. Striated muscles that are only infrequently or briefly contracted can rely upon less energetically efficient, but much faster, means of replenishing cellular ATP stores. These faster alternatives include [substrate-](javascript:void(0)) [level phosphorylation](javascript:void(0)) via glycolysis or other high-energy phosphates (eg, [creatine phosphate](javascript:void(0)), also known as phosphocreatine, Figure 17.8). A diagram of a normal curve Description automatically generated Chapter 17: Muscular System 562 **Figure 17.8** Anaerobic and aerobic ATP production in skeletal muscle cells. In contrast to striated muscle, smooth muscle achieves energetic balance and avoids fatigue by minimizing energetic demands through efficient force production. As a result, smooth muscle typically has less myoglobin and fewer mitochondria than striated muscle, despite prolonged force production in smooth muscle. 17.1.03 Muscle Excitation The force-producing interaction between actin and myosin filaments requires activation (ie, it is not always \"on\"), and this activation occurs differently in different muscle types. Usually, activation takes the form of an electrical signal, a wave of depolarization that spreads along and excites the myocyte membrane. Differences in muscle activation are related to whether contraction of a muscle is under conscious control and whether myocytes within the muscle are electrically connected with each other. **Involuntary**  Chapter 17: Muscular System 563 **muscles** (ie, smooth and cardiac) carry out automatic functions, while **voluntary muscles** (ie, skeletal) are involved in conscious movement or force production. Some involuntary muscles have specialized intercellular connections called **gap junctions**. These connections allow the muscle to act as a **syncytium**, or a collection of connected cells effectively sharing cytoplasmic molecules via the pores formed by gap junctions (Figure 17.9). Gap junctions allow the contraction of all cells in a muscle at the same time by electrically connecting the cells. All cardiac muscle cells have gap junctions, allowing for synchronous contraction of the cells in each heart chamber, as do most smooth muscle cells (eg, intestinal smooth muscle cells). **Figure 17.9** Gap junctions in cardiomyocytes (a functional syncytium). As mediators of automatic, subconscious functions, involuntary muscles are innervated by nerve fibers of the [autonomic nervous system](javascript:void(0)) (one branch of the [peripheral nervous system](javascript:void(0))). In cardiac muscle and certain smooth muscles, the action potentials that cause contraction occur without input from the nervous system (ie, some of the cells spontaneously depolarize). However, neural regulation influences the frequency and intensity of contractions. In other smooth muscles, a nervous impulse is necessary for contraction initiation. In addition to neural regulation, involuntary muscle contractions can be regulated by certain [hormones](javascript:void(0)). For example, [epinephrine](javascript:void(0)) can promote contraction or relaxation in smooth muscle depending on the type of receptor the epinephrine binds. In addition, some smooth muscle cells can be activated by chemical signaling, in which ligand binding to a receptor activates muscle contraction without depolarization. Voluntary (ie, skeletal) muscles allow conscious control of body movement, position, and processes. Although defined by their ability to be voluntarily activated, voluntary muscles can also be activated involuntarily, such as during a [reflex response](javascript:void(0)). Likewise, during repetitive activities such as breathing or walking, rhythmic contractions can be involuntarily maintained as a special type of reflex movement but can also be influenced by conscious input. Similarly, conscious control of a few voluntary muscles mainly involves a brief interruption of prolonged contraction (eg, conscious relaxation of a sphincter muscle). Chapter 17: Muscular System 564 Skeletal muscles are innervated by the somatic nervous system. Although all [synapses](javascript:void(0)), including those in cardiac and smooth muscle, involve a connection between a neuron and a target cell, the interface between a somatic motor neuron and a skeletal muscle fiber is a specialized synapse known as a **neuromuscular junction (NMJ)**. An NMJ between a motor neuron and a skeletal muscle fiber is illustrated in Figure 17.10. **Figure 17.10** A neuromuscular junction. An NMJ converts motor neuron action potentials into muscle fiber action potentials through a series of steps, as shown in Figure 17.10: 1.The arrival of an [action potential](javascript:void(0)) at the axon terminal of a presynaptic motor neuron triggers release of acetylcholine (ACh) from synaptic vesicles into the synaptic cleft via exocytosis. 2.Some ACh diffuses across the synaptic cleft to bind ligand-gated ACh receptors (channel proteins) embedded within the sarcolemma of the postsynaptic muscle fiber (any unbound ACh either diffuses away or is degraded by the enzyme [acetylcholinesterase](javascript:void(0))). 3.ACh binding leads to channel opening.  Chapter 17: Muscular System 565 4.Na+ flows down its electrochemical gradient and into the cell through the open channels, resulting in depolarization of the sarcolemma and generation of an action potential that propagates along the muscle fiber in all directions. 5.At certain locations along muscle fibers, the sarcolemma extends deep into the cells, forming invaginations known as transverse tubules (t-tubules). Spreading of the action potential into t-tubules brings the depolarizing current close to the sarcoplasmic reticulum, a specialized endoplasmic reticulum that plays an essential role in skeletal muscle contraction (described in more detail in Concept 17.1.04). 17.1.04 Excitation-Contraction Coupling Excitation of a muscle cell triggers a series of steps that lead to calcium ion (Ca2+) release and contraction in a process referred to as **excitation-contraction coupling** (Figure 17.11). In striated muscles (ie, skeletal and cardiac muscle), [depolarization](javascript:void(0)) triggers the release of Ca2+ from the [sarcoplasmic reticulum](javascript:void(0)) and from extracellular fluid into the cytosol. In skeletal muscle, Ca2+ released only from the sarcoplasmic reticulum is required for contraction, whereas in cardiac muscle, Ca2+ entering from outside the cell plays an important role as well. **Figure 17.11** Excitation-contraction coupling in skeletal muscle. The increase in intracellular Ca2+ triggers the **cross-bridge cycle**, in which force is produced by actin-myosin interactions (Figure 17.12). In striated muscles, the cross-bridge cycle occurs as follows: 1.At rest (ie, before intracellular Ca2+ rises), myosin heads are loosely associated (ie, weakly bound) with actin and bound to ADP and Pi. Actin filaments are bound to troponin and tropomyosin. **Troponin** is a small protein complex associated with **tropomyosin**, an elongated protein wrapped around actin filaments. At rest, tropomyosin prevents the strong actin-myosin binding required for force generation. Chapter 17: Muscular System 566 2.In response to increased cytosolic Ca2+ concentration during muscle excitation, Ca2+ binds troponin, causing a conformational change to tropomyosin described by the **sliding filament model**. This conformational change fully exposes myosin binding sites on actin filaments, allowing myosin heads to strongly bind actin filaments and form a **cross-bridge**. 3.Cross-bridge formation triggers the **power stroke**, in which myosin heads pull strongly on actin, and the eventual dissociation of Pi and ADP from myosin heads. If the force exerted by the myosin heads on actin is greater than any opposing forces, the actin filament will slide past the myosin molecule to which the actin is bound, and the muscle fiber will shorten. 4.Following the power stroke, new ATP molecules bind myosin, which leads to the temporary dissociation of actin and myosin cross-bridges. 5.ATP hydrolysis results in ADP and Pi once again being bound to myosin heads and weak binding between myosin heads and actin. This process continues cycling until the intracellular Ca2+ concentration falls when muscle activation ceases, leading to muscle relaxation. The decline in Ca2+ is primarily due to pumping of Ca2+ back into the sarcoplasmic reticulum by sarcoplasmic reticulum Ca2+-ATPase (SERCA). **Figure 17.12** The cross-bridge cycle of muscle contraction.  Chapter 17: Muscular System 567 Smooth muscle excitation-contraction coupling resembles that in striated muscle, with a few important differences. In smooth muscle, Ca2+ binds to the protein **calmodulin (CaM)**, rather than troponin, to regulate the cross-bridge cycle, and myosin phosphorylation plays an essential role. The general process in smooth muscle occurs as follows (Figure 17.13): 1.Increased intracellular Ca2+ concentration leads to the formation of Ca2+/CaM complexes. 2.Ca2+/CaM complexes bind to the enzyme **myosin light chain kinase (MLCK)**, thereby increasing MLCK activity. 3.MLCK phosphorylates a protein called **myosin regulatory light chain (RLC)**, a part of the myosin molecules composing the thick filament. 4.RLC phosphorylation by MLCK facilitates the formation of actin-myosin cross-bridges and force development. As in striated muscle, cross-bridge cycling can continue as long as the intracellular Ca2+ concentration remains elevated. 5.Dephosphorylation of RLC by the enzyme **myosin light chain phosphatase** promotes eventual cross-bridge detachment. However, this detachment sometimes occurs very slowly after dephosphorylation, allowing smooth muscle to enter a state in which force is sustained due to continued actin-myosin interaction. This *latch* state is one means by which smooth muscle is able to maintain force in a very energetically efficient manner without fatiguing. 6.Smooth muscle relaxation occurs when the intracellular Ca2+ concentration is lowered by removal of Ca2+ from the cytosol into either the sarcoplasmic reticulum or the extracellular fluid. This reduces Ca2+ binding to CaM, a situation analogous to lowered intracellular Ca2+ concentration promoting striated muscle relaxation by reducing Ca2+ binding to the troponin complex. Chapter 17: Muscular System **Figure 17.13** Smooth muscle excitation-contraction coupling. A diagram of a cell Description automatically generated