Bio 16.1-2 Excretion System Structure and Function PDF
Document Details
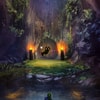
Uploaded by iiScholar
Arizona State University
Tags
Summary
This document describes the structure and function of the excretory system, focusing on the kidneys. It details the anatomy of kidneys, nephrons, and the process of urine formation and excretion. The document explains the role of the excretory system in maintaining homeostasis.
Full Transcript
Enter word / phrase to search UBook text Automatic ZoomActual SizePage Width100%50%75%100%125%150%200%300%400% Chapter 16: Excretion 536 Lesson 16.1 **Excretory System Structure** Introduction Excretion involves disposing of wastes from the body. While both the digestive system (see Chapter 1...
Enter word / phrase to search UBook text Automatic ZoomActual SizePage Width100%50%75%100%125%150%200%300%400% Chapter 16: Excretion 536 Lesson 16.1 **Excretory System Structure** Introduction Excretion involves disposing of wastes from the body. While both the digestive system (see Chapter 15) and the skin system (see Chapter 19) are involved in excretion, this chapter focuses on the specific role the kidneys play in waste excretion and how this waste is removed from the body. This lesson covers the structures of the excretory system. 16.1.01 General Excretory System Structure The **excretory system** is responsible for producing, storing, and excreting urine, the waste solution produced by the kidneys. Urine is produced as blood is filtered in the **kidneys** by individual subunits known as **nephrons**. Liquid urine is funneled from each kidney into paired tubes known as **ureters**, which carry the urine to the **bladder**. The bladder then stores urine for excretion, which occurs when urine travels through the **urethra** as it exits the body during [urination](javascript:void(0)). These excretory system structures are depicted in Figure 16.1. **Figure 16.1** Structures of the excretory system. 16.1.02 Kidney Anatomy The kidneys are anatomically divided into an outer **cortex** and an inner **medulla**, both of which contain portions of **nephrons**, the functional units of the kidney. Nephrons filter blood and selectively excrete or reabsorb the contents of the resulting filtrate (see Concept 16.2.02 for more information on nephron function). Filtrate produced in nephrons exits the renal medulla at **renal papillae**, which are the tips of pyramid-shaped structures known as medullary pyramids. Filtrate flows from the papillae into spaces called A diagram of the internal organs of a person Description automatically generated Chapter 16: Excretion 537 **calyces**, which come together to form the **renal pelvis**. From the renal pelvis, paired **ureters** transport filtrate to the bladder for storage prior to excretion from the body as urine (Figure 16.2). Entering and exiting each kidney near the renal pelvis are the **renal artery** and **renal vein**. Blood flow to the kidneys is discussed further in Concept 16.1.03. **Figure 16.2** Kidney anatomy. 16.1.03 Nephron Structure Within each kidney are numerous functional units known as nephrons (Figure 16.3), and each of these nephrons is composed of a glomerulus and associated tubular segments. The **glomerulus**, found in the renal cortex, is a ball-like network of [capillaries](javascript:void(0)) that receives and filters blood from the renal arteries. This filtered blood, known as filtrate, enters the **tubular segments** of the nephron, where the concentration of nutrients, electrolytes, water, and other substances is [adjusted](javascript:void(0)) to ultimately produce urine. The tubular segments can span both the cortex and the medulla. Surrounding each nephron tubule are capillaries (the **peritubular capillaries**). During urine formation, the peritubular capillaries allow the exchange of water and other molecules between the filtrate and the blood. The capillaries around medullary tubular segments are collectively known as the **vasa recta**.  Chapter 16: Excretion 538 **Figure 16.3** Nephron anatomy. A diagram of a kidney and urinary system Description automatically generated Chapter 16: Excretion 539 Blood enters each kidney via a renal artery, which branches into smaller arterioles. The arterioles entering each nephron are known as **afferent arterioles**, which branch further to form glomerular capillaries. Glomerular capillaries contain large pores, which are the first part of the three-part system (sometimes collectively referred to as the **filtration membrane**) by which blood passing through a glomerulus is filtered to produce filtrate. Glomerular capillary pores are large and serve as a coarse filter that excludes blood cells and large proteins from the rest of the blood components, which pass freely through. Glomerular capillaries are lined by a basement membrane with closely apposed Bowman\'s capsule epithelial cells. The basement membrane and epithelial cells serve as the second and third layers of the filtration membrane, respectively, blocking all but the smallest proteins while allowing passage of water and many small solutes (eg, glucose, ions, urea). Blood components too large to be filtered by the glomerular capillaries remain in circulation, exiting the glomerulus via **efferent arterioles**, as shown in Figure 16.4. **Figure 16.4** Blood filtration at the glomerulus is dependent on particle size. Filtrate passing through the glomerular filtration system is collected by **Bowman\'s capsule**, a cup-shaped extension of the renal tubule that surrounds the glomerulus, and is delivered into a long tubule composed successively of the following segments: 1.**Proximal convoluted tubule**: The first of the tubular segments, the proximal convoluted tubule delivers filtrate from Bowman\'s capsule to the loop of Henle. **\ 2. Loop of Henle**: This segment is a hairpin structure composed of two limbs. The descending limb moves filtrate down into the medulla, while the ascending limb transports filtrate out of the medulla and back to the cortex. Some nephrons have long loops of Henle that extend into the inner region of the medulla. These nephrons are known as **juxtamedullary nephrons**. Other nephrons, called **cortical nephrons**, have shorter loops of Henle that extend only into the superficial portions of the medulla (Figure 16.5). 3.**Distal convoluted tubule**: This is a tubular segment that conveys filtrate from the loop of Henle to the collecting duct. 4.**Collecting duct**: Sometimes also referred to as the collecting tubule, this is the tube into which fluid from the distal convoluted tubule flows. One collecting duct may serve several nephrons  **Excretory System Function** Introduction [Homeostasis](javascript:void(0)) or a stable internal environment, is vital to maintain in the body. The [excretory system](javascript:void(0)) (and the kidneys in particular) plays a large role in the maintenance of homeostasis through the regulation of fluid volume and composition, hormone production, and waste removal. In addition, the functions of the excretory system are largely linked to the regulation of urine formation and excretion. This lesson details the various functions of the excretory system in addition to how urine is formed and eliminated from the body. 16.2.01 Kidney Function By contributing to the regulation of osmotic balance, blood pressure, hormone production, waste removal, and blood pH, the [kidneys](javascript:void(0))play a large role in the maintenance of homeostasis in the body. Blood [pH](javascript:void(0)) (ie, [H+ concentration](javascript:void(0))) is maintained within a narrow range (7.35--7.45), primarily through regulatory mechanisms in the kidneys and lungs, and is influenced by carbon dioxide (CO2) levels. Most CO2 [transported](javascript:void(0)) in blood combines with water to form carbonic acid (H2CO3), which rapidly dissociates into bicarbonate (HCO3−) and H+: CO 2 \+ H 2 O⇋ H 2 CO 3 ⇋ HCO ― 3 \+ H \+ This reaction is known as the **bicarbonate buffer system** and shows that CO2 and H+ levels are related in the body. Because it is an [equilibrium](javascript:void(0)) system, the bicarbonate buffer system adjusts when perturbed according to [Le Chatelier\'s principle](javascript:void(0)). Therefore, the system can aid in maintaining blood pH. Various homeostatic regulatory mechanisms (eg, [changes](javascript:void(0)) in ventilation patterns in the lungs) are based on the bicarbonate buffer system. Lower blood CO2 concentrations decrease blood acidity (ie, increase pH) due to decreased H+ production. Conversely, higher blood CO2 concentrations increase blood acidity (ie, decrease pH) due to increased H+ production. In physiological settings, the acid-base response to a loss of H+ is functionally similar to a gain of HCO3- (ie, both result in increased HCO3-), and vice versa. The kidneys typically reabsorb most of the bicarbonate entering the filtrate. However, when extracellular fluid (eg, blood plasma, interstitial fluid) becomes excessively alkaline (high pH), the kidneys excrete HCO3− and retain H+, causing blood pH to decrease, as shown in Figure 16.6. Chapter 16: Excretion 542 **Figure 16.6** Role of the kidneys in acid-base balance when extracellular pH increases. Conversely, when the extracellular fluid becomes excessively acidic (low pH), the kidneys excrete H+ and retain HCO3−, causing blood pH to rise (Figure 16.7). Via these processes and others, the kidneys act with the lungs to maintain blood pH within the required narrow physiological range. **Figure 16.7** Role of the kidneys in acid-base balance when extracellular pH decreases. The kidneys likewise play an essential role in regulating **blood pressure (BP)**, or the force blood exerts on blood vessel walls. BP decreases when blood vessels [dilate](javascript:void(0)) (become wider) or when blood volume decreases. Hormones such as angiotensin II, aldosterone, and antidiuretic hormone (ADH; vasopressin) regulate BP by modulating reabsorption of water and salts by the kidneys (Figure 16.8).  Chapter 16: Excretion 543 **Figure 16.8** Regulation of blood pressure by the kidneys. The **renin-angiotensin system (RAS)** is a multiorgan molecular cascade activated when BP (or blood volume) falls (Figure 16.9). A drop in BP causes juxtaglomerular cells in the kidneys to release renin, an enzyme that cleaves the plasma protein angiotensinogen to form angiotensin I. Angiotensin-converting enzyme then cleaves angiotensin I to form angiotensin II. Angiotensin II ultimately raises BP by inducing both the constriction of arterioles (increasing BP without changing blood volume) and the release of aldosterone from the adrenal cortex (increasing BP by increasing blood volume, as described next). [Aldosterone](javascript:void(0)) is released in response to RAS activation or to an increased serum level of K+. Aldosterone acts on nephron distal tubules and collecting ducts to promote the reabsorption of Na+ and the secretion of K+. Increased reabsorption of Na+ increases the osmolarity, or solute concentration, of the renal interstitial fluid. Elevated osmolarity promotes water reabsorption, which ultimately causes blood volume and BP to increase. [ADH](javascript:void(0)) is a hormone released by the [posterior pituitary](javascript:void(0)) when BP falls or when blood osmolarity rises. ADH promotes water reabsorption by increasing the permeability of the distal tubule and collecting duct to water. Release of ADH also induces vasoconstriction, the narrowing of blood vessels. Both of these effects increase BP. The contributions of the RAS, aldosterone, and ADH to BP regulation are shown in Figure 16.9. Diagram of blood pressure Description automatically generated Chapter 16: Excretion 544 **Figure 16.9** The renin-angiotensin system, aldosterone, and ADH act to increase blood volume and blood pressure. The kidneys are vital to **osmoregulation**, or the process of regulating water and ion content, of the blood. During [osmosis](javascript:void(0)), water generally flows from *lower* to *higher* osmotic pressure environments across cell membranes (see Concept 5.2.03). **Osmotic pressure** is the minimum pressure that must be applied to prevent water movement by osmosis; in other words, osmotic pressure describes the extent to which water \"wants\" to cross a semipermeable membrane by osmosis. This pressure is the result of solute concentration differences on either side of the membrane: A *higher* solute concentration creates a *higher* osmotic pressure. Osmosis occurs across capillary walls (ie, semipermeable membranes) into the interstitial fluid and vice versa due to differences in the concentrations of solutes (eg, plasma proteins such as albumin). This water movement across capillary walls also occurs because of osmotic pressure, which in the capillaries is referred to as oncotic pressure. The kidneys help control blood [osmolarity](javascript:void(0)) by balancing the intake of specific molecules with the excretion of specific molecules in urine. For example, increased water consumption decreases blood osmolarity. To maintain homeostasis, higher concentrations of solutes (eg, Na+) are reabsorbed and more water is excreted by the kidneys, producing a larger volume of dilute urine. When blood osmolarity is high, the kidneys respond by increasing water reabsorption and decreasing solute reabsorption. In this way, the concentration of water and solutes in the blood stays relatively constant, as shown in Figure 16.10.  Chapter 16: Excretion 545 **Figure 16.10** The kidneys participate in osmoregulation when blood osmolarity decreases (left panel) or increases (right panel). In addition to being the target of several hormones (eg, aldosterone, ADH), the kidneys produce a hormone called **erythropoietin (EPO)**. EPO stimulates red bone marrow to [produce erythrocytes](javascript:void(0)) (ie, red blood cells) when blood oxygen levels fall. This increased production of erythrocytes leads to a rise in the oxygen-carrying capacity of the blood. Conversely, high levels of oxygen or erythrocytes inhibit the production of EPO, promoting homeostasis. The kidneys filter blood and produce liquid waste (**urine**) that is eventually excreted from the body. The body produces metabolic waste products such as creatinine from muscle metabolism and ammonia (NH3) from metabolism of compounds containing nitrogen. Because ammonia can disrupt extracellular fluid pH, it is [converted to urea](javascript:void(0)) in the liver before being delivered to the kidneys. The kidneys remove metabolic wastes such as urea and foreign substances (eg, drugs, environmental toxins) by collecting these wastes in the filtrate and excreting them in the urine. If the kidneys are unable to excrete waste, the resulting waste accumulation becomes toxic and can cause serious medical complications (eg, increased blood nitrogen levels due to lack of urea excretion). The details of urine formation by nephrons are covered in Concept 16.2.02. A diagram of a normal and response to osomalaria Description automatically generated Chapter 16: Excretion 546 16.2.02 Urine Formation Within each kidney are functional units known as **nephrons**, which perform blood **filtration** and concentrate urine. During urine formation, nephrons facilitate the removal of some solutes and waste products from the blood into filtrate (ie, fluid filtered into the nephron) as well as the **reabsorption** of useful solutes back into the blood from the filtrate (Figure 16.11). Waste products not filtered in the glomerulus are transferred from the blood to the filtrate in nephron tubules (**secretion**). **Renal clearance** is a measure of the ability of the kidneys to remove substances from the bloodstream to be excreted. **Figure 16.11** Filtration, secretion, and reabsorption are the processes involved in urine formation. Blood enters the kidneys via the renal arteries, which branch into smaller [arterioles](javascript:void(0)) and, ultimately, capillaries of the glomerulus. Glomerular capillaries are lined by a basement membrane that allows passage of water, many solutes (eg, glucose, ions, urea), and very small proteins into the filtrate. The filtrate is collected by Bowman\'s capsule, which surrounds the glomerulus. Materials not filtered by the glomerulus due to their larger size (eg, blood cells, large proteins) exit the glomerular capillaries. Additional details about blood flow and filtration in nephrons can be found in Lesson 16.1. The degree of vascular constriction of afferent and efferent arterioles controls the rate of blood flow into and out of the glomerulus, thereby affecting the volume of fluid filtered through the kidneys per unit time, known as the **glomerular filtration rate (GFR)**. These effects are detailed in Figure 16.12.  Chapter 16: Excretion 547 **Figure 16.12** Effect of dilation and constriction of afferent and efferent arterioles on glomerular filtration rate. Within the glomerulus, several pressures affect the movement of fluid from the glomerular capillaries into Bowman\'s capsule. The [hydrostatic pressure](javascript:void(0)) of glomerular blood (GBHP) tends to push fluid out of the capillaries, while the hydrostatic pressure of Bowman\'s capsule fluid (BCHP) tends to push fluid into the capillaries. Because GBHP is higher than BCHP, there is a driving force for fluid to flow from the capillaries into Bowman\'s capsule. Oncotic pressure (see Concept 16.2.01) also affects fluid movement in the glomerulus. The oncotic pressure of the filtrate in Bowman's capsule (BCOP) tends to pull fluid from the capillaries, while the glomerular blood oncotic pressure (GBOP) tends to pull fluid into the capillaries. Because larger proteins (eg, albumin) are not filtered and remain in the glomerular capillaries, GBOP is higher than BCOP. Therefore, net oncotic pressure tends to decrease fluid flow out of the capillaries, opposing the net hydrostatic pressure. The pressures affecting fluid movement in the glomerulus are summarized in Figure 16.13. A diagram of a human body Description automatically generated Chapter 16: Excretion 548 **Figure 16.13** Pressures in the nephron glomerulus. Filtrate travels from Bowman\'s capsule to the **proximal convoluted tubule (PCT)**, where important nutrients (eg, amino acids, vitamins, salts, glucose, water) are reabsorbed from the nephron and incorporated back into the blood through the peritubular capillaries surrounding the PCT. Waste products not filtered by the glomerular capillaries are actively secreted from the peritubular capillaries into the nephron. Next, filtrate flows into the **loop of Henle**, a hairpin structure composed of two limbs. The descending limb extends down into the relatively salty medulla (inner portion of the kidney) and is highly *permeable* to water but *impermeable* to NaCl. The ascending limb, which travels from the loop\'s lowest point in the medulla back toward the cortex (outer portion of the kidney), is *impermeable* to water but *permeable* to NaCl. These differential permeability characteristics in the two limbs act together to form a concentration gradient within the loop of Henle that maximizes water reabsorption, as described next. Water moves from areas of low solute concentration to areas of high solute concentration. Accordingly, because salt concentration in the medulla is *high*, water is passively reabsorbed (via osmosis) from the  Chapter 16: Excretion 549 filtrate flowing through the descending limb into the salty medulla, where the water is taken up by blood vessels (vasa recta). As the water is [passively reabsorbed](javascript:void(0)) via osmosis into the medulla, the filtrate becomes more concentrated. The ascending limb transports filtrate from the medulla and into the cortex. NaCl is first passively reabsorbed into the medulla as the filtrate travels up the ascending limb. As the limb nears the cortex, NaCl is [actively transported](javascript:void(0)) out of the filtrate and into the medulla, maintaining the medulla\'s high salt concentration and facilitating continued water reabsorption in the descending limb. Because water follows NaCl, the saltiness of the medulla promotes continued water reabsorption from the descending limb (and the collecting duct). This full **countercurrent multiplication system** is depicted in Figure 16.14. **Figure 16.14** Countercurrent multiplication in the loop of Henle. From the loop of Henle, filtrate enters the **distal convoluted tubule (DCT)**, where aldosterone and antidiuretic hormone (ADH; vasopressin) promote the reabsorption of water. Aldosterone is released from the adrenal cortex in response to increased serum potassium (K+) and low blood pressure. In response to aldosterone, nephrons reabsorb sodium (Na+) and secrete K+; however, more Na+ is reabsorbed than K+ is secreted. This generates an ion gradient between the filtrate and the fluid Chapter 16: Excretion 550 surrounding the nephron, causing water to be reabsorbed into the interstitial fluid (and eventually the bloodstream) via osmosis. When blood osmolarity increases (eg, through the actions of aldosterone), ADH is secreted from the posterior pituitary. ADH increases the permeability of the DCT and collecting duct to water, causing even more water to be reabsorbed. The actions of aldosterone and ADH promote an increased blood volume and blood pressure and cause urine to become more concentrated, decreasing the final excreted urine volume. Additional waste products are secreted into the DCT from the peritubular capillaries. Figure 16.15 summarizes the effects of aldosterone and ADH on urine formation. **Figure 16.15** Effect of aldosterone and ADH on urine formation. Finally, in the **collecting duct**, ADH and aldosterone act in the same way they do in the DCT, further concentrating urine and reducing its volume. Based on the state of the body, the amount of water reabsorbed varies; for example, when the body is hydrated, less water is reabsorbed and the volume of excreted urine is greater. Conversely, when the body is dehydrated, more water is reabsorbed, reducing urine volume and further concentrating the urine exiting the body. Reabsorbed water reenters the circulation via the peritubular capillaries. Once concentration in the collecting duct is finalized, urine is emptied into the ureters for eventual excretion. Figure 16.16 summarizes the location and function of each section of the nephron.  Chapter 16: Excretion 551 **Figure 16.16** Reabsorption and secretion of substances along the nephron. **Concept Check 16.1** After purchasing a new reusable water bottle, a student finds that he is drinking much more water each day than he was previously. Which portion(s) of the nephron are most impacted by the student\'s lifestyle change? How would these portion(s) respond to the student\'s increased water intake? A diagram of a structure Description automatically generated  Chapter 16: Excretion 552 [**Solution**](javascript:void(0)) 16.2.03 Urine Elimination In the excretory system, **urine** is produced in the nephrons within each **kidney**, as detailed in Concept 16.2.02. This urine from the kidneys is then funneled into each of the two **ureters**, the tubes connecting the kidneys to the **bladder**. Urine accumulates in the bladder until it exits the body via the urethra in a process called **urination** (Figure 16.17). **Figure 16.17** Components of the excretory system. Urination is under the control of both [smooth and skeletal muscle](javascript:void(0)) along the urinary tract (the system for removing urine from the body). Specifically, a layer of smooth muscle called the **detrusor muscle** lines the bladder and is relaxed while urine is stored in the bladder. The detrusor muscle is under involuntary control by the [autonomic nervous system](javascript:void(0)). While urine is being stored, two sphincter muscles remain contracted so urine cannot exit the body. The proximal sphincter muscle is known as the **internal urethral sphincter (IUS)**. The IUS is composed of smooth muscle and is under involuntary control, like the detrusor muscle. The distal sphincter, or the **external urethral sphincter (EUS)**, is composed of skeletal muscle and is under voluntary control by the somatic nervous system (Figure 16.18). During urination, activity within stretch receptors in the bladder leads to contraction of the detrusor muscle to push urine out of the bladder and into the urethra. At the same time, the IUS relaxes to open the urethra and allow urine through. For urine to be excreted from the body, IUS relaxation must be paired with voluntary relaxation of the EUS. Relaxation of the IUS alone will not allow urine excretion. A diagram of the internal organs of a person Description automatically generated