Microbial Genome Organization PDF
Document Details
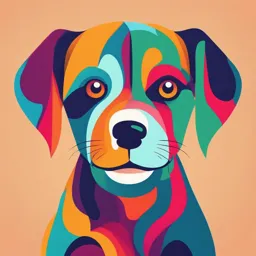
Uploaded by BriskAntigorite
Tags
Summary
This document provides an overview of microbial genome organization, focusing on the structure and function of genomes in prokaryotes and eukaryotes. It details the importance of microbial genetics, emphasizing its role in understanding fundamental biological processes.
Full Transcript
MICROBIAL GENOME ORGANIZATION I. Introduction to Microbial Genetics Microbial Genetics - studies the mechanisms of heritable information in microorganisms including bacteria, archaea, viruses, and some protozoa and fungi. Importance of Microbial Genetics 1. Understand gene function of microorganisms...
MICROBIAL GENOME ORGANIZATION I. Introduction to Microbial Genetics Microbial Genetics - studies the mechanisms of heritable information in microorganisms including bacteria, archaea, viruses, and some protozoa and fungi. Importance of Microbial Genetics 1. Understand gene function of microorganisms 2. Provide a relatively simple system for studying phenomenon & this is useful to higher microorganisms. 3. Used for isolation and multiplication of specific genes of higher organisms - If you have a simpler mechanism/organism, it is much easier to understand → the universal mechanism 4. Provide value added productions (e.g. antibiotics, growth hormones) and help increase these products production by microbial technology. 5. Understanding the genetics of disease-causing microorganisms, especially viruses, will be useful to control diseases. 6. Useful in the study of genetic transfer from one organism to another from one organism to its environment. - Only 1% if an organism genome will be expressed. - Gene - basic unit of heredity; segment of DNA coding for a functional product. RNA is an example of a non-protein gene product: True The higher the number of genes, the higher the complexity: False Chromosome - structure made up of DNA; linear in eukaryotes and circular in prokaryotes. Genome - complete set of genetic info in the cell E.coli - first organism to have its whole genome sequenced. Genotype - genes of an organism. For organisms with more than one set: heterogenous/homogenous. Phenotype - expression of the genes Recombination/Insertion/HGT transfer of genetic information within two cells. II. Prokaryotic Genome Organization chromosomes are Prokaryote relatively simple DNA molecules → 1 DNA (circular) ○ Usually a single nucleic acid molecule ○ Devoid of associated proteins and contains relatively less genetic information. Double-stranded DNA, compacted into a nucleoid Haploid - single copy of chromosome Mostly circular but ○ Some are linear; e.g. Borrelia streptomyces, Rhodococcus ○ Some with a combination e.g. Borrelia burgdorferi Agrobacterium tumefaciens: Crown Bladder Disease - liver/kidney disorder. Instead of pathogen, it’s used as a vector for beneficial effects. B. burgdorferi - pathogen of humans and mammals. Causes lyme disease → vectored by ticks from tick fever since they carry the said pathogen Has to fit in a very tiny space Supercoiling - facilitates compaction of DNA in bacterial genomes. Circular DNA twists and turns, forming loops. Supercoiling is controlled by ○ DNA topoisomerase: relaxes supercoiling ○ DNA gyrase: nucleosome complex; induces negative supercoiling ○ HU - bacterial protein, acts similarly to eukaryotic histones Highly conserved in prokaryotes supercoiling ○ Negative counterclockwise supercoiling ○ Positive clockwise Adding/subtracting twists imposes strain twist - turn DNA to its helical axis. - - Have plasmids ○ Extrachromosomal autonomous ○ Independent, replicon genes Contains needed for replication to happen. ○ May integrate into the chromosome ○ Indispensable CP - circular plasmid LP - linear plasmid Genes essential for bacterial growth, often referred to as housekeeping genes, are carried on the chromosomes and plasmids carry genes associated with specialized functions. Examples of metabolic activities determined by plasmids : Pseudomonas s.p - degradation of camphor, toluene, serene, salicylic acid. Genome Size ○ 50kb - 13mn ○ Restricted ecological niche (e.g. obligate intracellular parasites, endosymbionts) = smaller genes ecological Have requirements. with complex ○ Species developmental cycles. Operons ○ Functioning unit of DNA containing a cluster of genes under the control of a single promoter. ○ Allows for simultaneous regulation of gene clusters as genes share a common promoter and repressor. ○ Lac operon - contains: promoter, operato, structural genes (b-galactose, b-galactoside permease, agalactoside transacetylase) DNA - not transcribed due to a repressor bound to a-operator. - Higher lactose in the environment - lactose will bind to the repressor and change the conformation of the repressor. - RNA polymerase will pass through and transcribe Lac z & Lac a - important for cleaving of lactose into the molecule. Permease - enzyme that catalyzes transport of lactose into the cell. Examples of genome organization of prokaryotes - E.coli K-12 → 1 circular mol → 3.639 mb → 4.399 - Vibrio cholerae II. Eukaryotic Genome Organization Linear arrangement of of DNA Eukaryotic genomes generally longer than prokaryotes but contain more non-coding genes, Haploid to polyploid. Genome size ranges vary from < 10 mb (some fungi) to > 100,000 mb (plants, salamanders, lung fishes, etc.). Most conservative mode of evolution. III. Genome Complexity Complexity of an organism is multifaceted: - Genome size - Genome number - Genome architecture - Evolutionary history - Lifestyles Genome Size - Not a perfect measure - May potentially indicate a higher complexity - Larger genomes give more space to genes, regulatory elements, and non-coding DNA (higher chances of winning) → some exceptions exist, like the parasitic worm Trichinella spiralis (pork worm). Small but intricate genome → 100-200 mB: regular nematode → 64 mb: T. spiralis → has more complex capacity compared to other nematodes. → Intermediate host (pork) – parasite in protective covering – eating uncooked pork – viable pathogen alive – will pass through digestive tract – will survive due to covering – will stay in intestine – produce larvae - lodge in intestinal - larvae would erode and infect blood vessel - gain access to circulatory system – invading muscular fibers – invade collagen fibers and infect more muscles. Gene Number - Not a direct measure - 1 gene = 1 product theory has been debunked. - Organisms with more protein-coding genes might possess greater functional and metabolic diversity. - Presence of pseudogenes and the functional importance of non-coding elements complicate this measure. - Regulatory mechanisms, coding efficiency, unique genes = thus, not linear. Genome Architecture - The totality of non-random arrangement of functional elements (genes, arrangement of genes, regulation regions, etc.) in the genome Replicons - Covalently closed DNA circles (plasmids, bacterial chromosomes_ that can independently replicate → contain genetic information necessary for their own replication. Pathogenicity islands - Specific genes for pathogenic determinants (easier replication) - Often clustered together in the DNA - Make some bacterial species more efficient at causing disease in higher organisms. → Higher virulence, greater damage → pathogenicity: what or who it can infect - Flanked by direct repeats - Contain diverse genes important for pathogenesis - antibiotic resistance - Adherins - Invasins, exotoxins - Genes involved in genetic mobilization Transposons - Transposons/transposable elements (TES) in bacteria - “Jumping genes” - Stretch of DNA that can jump into different places of the genome. - Can cause spread of antibiotic resistance Evolutionary History - Accumulation of more genetic adaptations over time= higher genome complexity compared to ancestors - → Evolution =/ adaptations. Complex evolutionary pressures can also lead to genome streamlining in specific lineages. - Environmental pressure might require more complex genetic mechanisms, potentially reflected in the genome size and structure. Lifestyle - Multicellularity, diverse behaviors, or intricate metabolic pathways might necessitate a more complex genetic toolkit. - Symbiotic relationships - interactions potentially lead to gene exchange and co-evolution with their partners. - Relationships with organisms might affect your genome, depending on the organisms you interact with (lateral gene transfer may occur). THE FLOW OF GENETIC INFORMATION IN BACTERIA I. Replication A cell uses the genetic information contained in the DNA to make its proteins, including enzymes, This information is transferred to the next generation during cell division. DNA can be transferred to cells in the same generation, resulting in new combinations of genes. The replication of bacterial DNA is a highly coordinated process that ensures the accurate duplication of the genetic material. The process begins with the unwinding of the double-stranded DNA molecule at a specific site called the origin of replication. DNA helicase enzymes unwind the DNA, creating a replication fork, and DNA polymerase enzymes then synthesize the complementary strands, using the original strands as templates. The replication process is semiconservative, meaning that each new DNA molecule contains one original strand and one newly synthesized strand. This ensures that the genetic information is faithfully passed on to the daughter cells during cell division. Bacteria also have mechanisms to maintain the integrity of their DNA, such as DNA repair systems and proofreading activities of the DNA polymerase enzymes. Replication - Genetic information can be transferred between generations of cells. Expression - genetic information is used within a cell to produce the protein needed for the cell to function Recombination - Genetic information transferred between cells of the same generation. Key Concept: DNA is the blueprint for a cell’s proteins and is obtained from a parent cell or from another cell. Starch Hydrolysis Bacteria are able to hydrolyze starch and break them down as a food source. Essentially testing if they produce amylase. ○ Amylase enzyme is a protein and they could be produced if you have the gene or DNA sequence. sequence can be ○ The translated to make the enzyme which allows them to do the metabolic process. → Dictates what metabolic pathway they can do depending on the enzyme and genetics. Recombination Horizontal gene transfer (HGT) Transfer between cells of the same generation Look at the type such as conjugation wherein one can form a sex pili with an adjacent cell and pass DNA from one cell to another.. The Biological Problem Where does a cell’s DNA go when the cell divides? Cells containing genetic info that don't divide produce one daughter cell with DNA and the other doesn’t. Thus, the cell copies (replicates) DNA first before the cell divides. They make an exact copy - two copies split into two cells which are the daughter cells. The offspring: identical Finding the Structure of DNA Watson and Crick published a model of DNA in 1953. Their model used several facts 1. DNA is a linear polymer of 4 types of bases linked by sugar-phosphate groups, 2. The ratio of bases is always A=T, G=C (Chargaff’s ratio). 3. DNA is helical with a helix width and bases spacing that was known from work by Rosalind Franklin. X-ray crystallography ○ Used to determine the helical structure of DNA ○ Crystallizes DNA passed through X-ray with a film refract and produce the said image. ○ Watson and Crick stole the discovery of Rosalind Franklin Knew it was a double helix ○ Franklin got cancer due to the x-ray and died without a nobel prize. specific DNA sequences called promoters and initiates the transcription process. During transcription, the RNA polymerase enzyme unwinds the DNA, exposing the template strand, and then synthesizes a complementary mRNA molecule. The mRNA molecule is then processed, with the removal of any non-coding sequences (introns) and the addition of a 5' cap and 3' poly(A) tail, which help to stabilize the mRNA and facilitate its translation. In bacteria, transcription and translation are coupled, meaning that the translation of the mRNA into protein can begin before the transcription process is complete. This allows for a more efficient and rapid response to changes in the cell's environment or metabolic needs. III. Translation Translation is the process of synthesizing proteins from the mRNA template. This process is carried out by the ribosomes, which are large, complex molecular machines composed of ribosomal RNA (rRNA) and proteins. During translation, the ribosome reads the mRNA sequence and recruits the appropriate transfer RNA (tRNA) molecules, each carrying a specific amino acid. The tRNA molecules recognize the codon (a sequence of three nucleotides) on the mRNA and bring the corresponding amino acid to the ribosome. The ribosome then catalyzes the formation of a peptide bond between the amino acids, building the polypeptide chain. II. Transcription Transcription in bacteria is the process of synthesizing mRNA molecules from the DNA template. This is carried out by the enzyme RNA polymerase, which recognizes As the translation process progresses, the ribosome moves along the mRNA, adding amino acids to the growing polypeptide chain. Once the ribosome reaches a stop codon on the mRNA, the polypeptide chain is released, and the ribosome dissociates from the mRNA. IV. Control of Gene Expression Bacteria have evolved various mechanisms to regulate gene expression in response to intracellular or environmental conditions. This allows them to adapt to changes in their environment and optimize the use of their limited resources. One of the primary mechanisms of gene expression control in bacteria is transcriptional regulation. Bacteria use transcriptional regulators, such as repressors and activators, that can bind to specific DNA sequences (called operator or promoter regions) and modulate the rate of transcription. Repressors are proteins that bind to the operator region and block the binding of RNA polymerase, thereby inhibiting transcription. Activators, on the other hand, bind to the promoter region and facilitate the recruitment of RNA polymerase, increasing the rate of transcription. Bacteria also employ post-transcriptional and post-translational mechanisms to control gene expression. These include the use of small regulatory RNAs (sRNAs) that can bind to mRNA molecules and modulate their stability or translation, as well as the regulation of protein activity through mechanisms like phosphorylation or proteolysis. MOLECULAR GENETIC ANALYSIS I. Restriction Site Mapping & Molecular Analysis Restriction Enzymes - Isolated DNA can be cut at specific sites by enzymes called restriction enzymes Isolated from bacteria Recognize and cleave DNA at 4 to 8 specific nucleotides in a palindromic sequence ○ Sequence that reads the same 5’ to 3’ on one strand and 3’ to 5’ direction on complementary strand. If they cut symmetrically between two bases opposite each other on complementary strands → resulting DNA have blunt ends If the endonuclease makes staggered cuts across the two DNA strands leaving each fragment an overhand of unpaired bases → ends of DNA fragments are said to be sticky. - These ends can pair with single stranded overhangs of another piece of DNA cut by the same restriction enzyme. → Different DNA fragments with sequence-specific DNA sequences called a restriction site. Restriction Enzyme Digests - recognizes and cleaves DNA sequence called a restriction site. Background and Nomenclature Where do R.E. come from? Adaptation of bacteria that act as defense mechanism against viruses, known as bacteriophages. Due to addition of methyl groups, R.E. can only recognize and phage DNA R.E. names ○ HindIII, NotI, EcoRI, BamHI, TaqI, HistII ○ First three letters refer to the organism which it was ○ ○ isolated from (e.g. EcoRI; Eco → E.coli) Second letter, if necessary, refers to the bacterial strain from which it was isolated. Roman numeral indicates whether it was the first, second, or 3rd enzyme isolated from a particular organism Basic Principles RE recognizes a series of nucleotides usually 4 base pairs long called a recognition site. At specific nucleotides within the sequence, the enzyme will break the phosphodiester bonds in the DNA backbone Direct recognition sites are usually palindromic (sequence reads the same forwards and backwards) ○ When the palindrome is found a complementary strand of the DNA molecule it is called an inverted-repeat palindrome RE can leave different types of ends once the DNA is cleaved. ○ Sticky ends - leave 3’ and 5’ overhangs ○ Blunt ends - leave no overhangs. The type of end dictates how the DNA fragment is isolated by the KED; will be recombined with other DNA fragments in a process called ligation. Restriction Enzyme Digests Digestive reaction typically consists of ○ Deionized water ○ DNA to be cut ○ Buffers specific to the enzyme used ○ Protein called bovine serum albumin (BSA) → will stabilize the reaction by preventing enzymes from sticking to the sides of the container that houses the digest. R.E. BSA required Buffer Incubation Temp BamH1 Yes X 37 C NotI No Y 37 C SpeI Yes Z 50 C May have different buffers, incubation temperatures, and requirements for BSA. 1. Retrieve the restriction enzyme from the fridge. Keep restriction enzymes on ice or thermoresistant containers to make sure there is optimal activity for future reactions. 2. Microfuge tubes: a volume of sterile nuclease free water that will yield a final reaction volume of 20 microliters. 10x restriction buffer, BSAM 1 microgram of DNA, 2-10 units of enzyme. 1 unit = 1 microgram DNA digested in 1 hr of 37C in 50 microliter. 3. Mix by vortexing, centrifuge briefly at 12000 times g in a microcentrifuge. 4. Incubate at an optimal temperature for restriction enzyme: 37C in a heating block for 1-4 hours. 5. Incubate the reaction mixture at 65 C to heat and activate restriction enzymes. 6. Prolonged incubation times may result in star activity: cutting at sites that are similar but distinct from typical digestion sites. 7. Following inactivation, DNA should be run in an agarose gel to ensure that the digest was successful. → Sometimes multiple enzymes have to be used to generate a specific DNA fragment. Check if buffer conditions and incubation temperatures are compatible between two enzymes. If so, double digest may be performed. pieces and then identifying the locations of the breakpoints. → DNA segment that shows the positions of the restriction sites is called the restriction map. Samples: → Use enzymes sequentially if conditions are not compatible. Purify DNA following the initial digest, then do a second digest. → The use of control DNA with known restriction sites allows the enzyme activity to be tested. Applications: 1. Screening Samples: can be used diagnostically to identify samples. → Missing 6kb → Restriction Fragment Length Polymorphisms (RFLPs) → loading digests into specialized chips (placed into a machine called bioanalyzer) - Researchers can examine DNA fragment sizes produced by the digests to determine the authenticity of the sample → Different banding patterns of the same gene from a given species called restriction fragment length polymorphism (RFLPs) 2. Subcloning – isolate a fragment of DNA in one plasmid and insert it into another so the desired fragment can be replicated using bacteria. 3. Detecting SNPs - by employing PCR to introduce genes to very specific locations → Can be used to Determine the presence of single nucleotide differences in alleles. Restriction Mapping - method used to map an unknown segment of DNA by breaking into Solution explanation Single Digests: HpaI: One band at 26 kb indicates a single HpaI site. HindIII: Four bands at 13 kb, 6 kb, 4 kb, and 3 kb indicate multiple HindIII sites. Combination Digest: HpaI and HindIII: Four bands at 7 kb, 6 kb, 4 kb, and 3 kb. Analysis: The HpaI site must be within the largest HindIII fragment (13 kb) because the combination digest does not have a 13 kb band. We know the 6 kb, 4 kb, and 3 kb bands are unchanged in the combination digest, so they are separate fragments with only HindIII sites. The combination of 7 kb and 6 kb equals 13 kb, which suggests that the 13 kb HindIII fragment is split by the HpaI site. This would cut the plasmid at both sites and yield three fragments. The sizes of these fragments would be 0.2 kb, 0.26 kb (0.46 kb 0.2 kb), and 25.54 kb. → Double Digestion with EcoRI and PstI: This would yield three fragments: 0.46 kb, 1.48 kb (1.94 kb - 0.46 kb), and 24.06 kb. Double Digestion with BamHI and PstI: This would yield three fragments: 0.2 kb, 1.74 kb (1.94 kb - 0.2 kb), and 24.06 kb. Steps to create plasmid map:1) Digestion with EcoRI produces a single fragment of 20 Kb. This means that the plasmid is 20 Kb in size and contains only one restriction site for EcoRI. → Digestion with EcoRI: The EcoRI site divides the plasmid into two fragments: one of 0.46 kb and the other of 25.54 kb (since the total size is 26 kb and there's only one EcoRI site). → Digestion with BamHI: The BamHI site would similarly divide the plasmid into two fragments: one of 0.2 kb and the other of 25.8 kb. → Digestion with PstI: The PstI site would yield two fragments: one of 1.94 kb and the other of 24.06 kb. Double Digestion with EcoRI and BamHI: 2) Digestion with BamHI produces 3 fragments. Thus, the plasmid contains three restriction sites for this enzyme. (The first cleavage by the enzyme will linearize the plasmid DNA. The second cleavage will produce two fragments and the third cleavage event will clear one of the fragments in two thus, yielding three fragments in total). According to the fragment sizes, restriction sites will be placed on the plasmid. 3) Digestion with both the restriction endonucleases should produce fragments whose total size should add up to 20 Kb i.e. the size of the plasmid. In this case, the size of three fragments adds up to 17 Kb. This is because there will be two fragments of 3 Kb. 6. The agarose solution is left undisturbed. The gel consists of hydrogen bonded agarose molecules forming a porous matrix to which the DNA molecules move. 7. DNA samples are mixed with a loading buffer and loaded onto the gel. The loading buffer consists of glycerol, which increases the density of DNA samples and helps them settle at the bottom of the well. They also contain dyes, which monitor migrating DNA bands. Agarose Gel Electrophoresis 8. Negatively charged DNA migrates to the positive electrode. Larger DNA molecules move more slowly than smaller molecules allowing size separation. Size depends on agarose concentration. Higher agarose concentration = smaller pore size. Agarose Gel Electrophoresis is a technique commonly used to separate DNA molecules by their size. → Integration: DNA Isolation and Restriction Enzyme Analysis 1. Agarose, a polysaccharide derived from red algae, is added to a buffer such as tris-acetate EDTA (TAE) or Tris-borate EDTA (TBE) and dissolved by heating. → DNA extraction is the removal and the purification of DNA from dells. First, cells are lysed: broken open by a combination of physical and chemical treatment, the treatment of detergents which dissolves cell and nuclear membrane. 2. 1 gram/100 mL = 1% agarose solution. 3. To stain the gel, a fluorescent dye such as ethidium bromide is often added to the agarose solution. 4. The solution is poured into horizontal modes. 5. A comb is inserted into the gel cast to create wells to which DNA samples can be loaded. → SDS or sodium dodecyl sulfate is a commonly used detergent that works by solubilizing proteins and lipids that make up these membranes. → DNA must be separated from the other molecules present. Proteinase K added to the reaction will break down peptide bonds and digest contaminating proteins. → Salt is added which stabilizes the negative charged phosphate groups in the backbone of the DNA, and after the addition of ice-cold alcohol, precipitates the DNA out of the solution. → White precipitate is collected by spinning in a centrifuge which settles in the bottom of the tube. → Washing and resuspension. → DNA Fingerprinting – can identify novel patterns in DNA. From smaller techniques of molecular analysis, you can combine techniques to form some DNA sequencing techniques. 2. To remove proteins, enzymes such as proteinase K or peptidase K or lysozyme are added to supernatant - Breaks peptide bonds 3. DNA is recovered from the supernatant through precipitation (addition of alcohol and salt; sodium acetate) - Integration DNA Isolation and Restriction Enzyme Analysis II. DNA Extraction and Polymerase Chain Reaction DNA Isolation DNA is isolated in cells is cut in precise positions for many applications (e.g. recombinant DNA technology) ○ Different types of DNA Extraction are used for different cell types 1. Cell lysis 2. Protein removal 3. DNA recovery 1. Inside eukaryotic cells, DNA is packed within the nucleus. Both cell membrane and nuclear membrane need to be ruptured to isolate DNA mechanically by breaking down cells through grinding/sonication or chemically by using detergents and enzymes to dissolve parts of the CM. - Once released, debris are separated from soluble components using centrifugation. - Supernatant: contains nucleic acids and water-soluble proteins. Isolated precipitate is dissolved in water or a buffer. Removal and purification of DNA from cells. 1. Cells are lysed - broken usually though combination of physical disruptions mixed with chemicals (e.g. detergent) - Dissolve cell in the nuclear membrane - SDS (sodium dodecyl sulfate) - Commonly used - Stabilizes proteins and lipids making up membrane = content more freely in the surrounding solution. 2. DNA must be separated from other molecules present - Proteinase K - break down peptide bonds and digest contaminated proteins; added to solution. - Salt - added to sample, stabilizes negatively charged backbone - Alcohol - after addition of ice cold alcohol, precipitates DNA out of the solution -> DNA Precipitation - White Precipitate - collected and spun in a centrifuge where it settles at the bottom of the tube. DNA Fingerprinting Can identify novel patterns of DNA specific to an individual - - Involve R.E. interact with DNA Recognize specific DNA sequences Once specific sites are identified, they cut the DNA resulting in the strand being cut in 1 or more linear places. If DNA extracted was a Plasmid ○ Circular piece of DNA, often found in bacteria ○ Any cuts will result in linear DNA fragment/s. Different R.E. recognize different DNA sequences - Using a combination of these = distinct fragments. In DNA Fragmenting, we can examine fragments using DNA gel electrophoresis. Gel preparation 1. Powdered agarose with buffer 2. Heated until dissolved 3. Nucleotide stain is added to warm mixture 4. Solution is inserted to form the wells 5. Wall com is inserted to form walls 6. Once solid, gel is transferred to gel box filled with buffer and comb is removed 7. Sequence mixture of dyed DNA fragments of no length; DNA ladder is added to each and dyed DNA samples are loaded to the remaining wells. 8. Connected to power source DNA is not typically visible during electrophoresis. ○ Migration of (-) to the (+) side; cathode, anode ○ Smaller pieces move more quickly than larger fragments 9. Once complete, gel is exposed to UV to visualize nucleotide strain in DNA samples Presence can be confirmed based on relative location of lateral bands. Polymerase Chain Reaction Primers - short stretches of nucleotide sequence to initiate DNA synthesis → help identify unique part of genome → single stranded → Forward and reverse primers: the direction is always 5’ to 3’. Forward Primers: will attach to the antisense. Reverse Primers: will attach to the sense strand at the 3’ portion. Problem A DNA with the sequence : 5’CGCATCGATCATGCCCTGA….AGCCCA TTAGATGCC3’. Needs to be amplified using PCR What is the sequence the best reverse primer will have? 5’GGCATCTA3’ 1. Heat mixture to denature protein 2. Reaction is cooled and primers will anneal to homologous regions 3. Once bound, the reaction is heated at the optimal temperature to bind the polymerase. 4. The polymerase recognizes the primer DNA complex and begins synthesis of the new strands using DNTPs in the solution 5. Reaction is heated and proceeds as described across additional cycle → following the 3rd cycle There are eight copies of the gene 6. After the 4th cycle: 16 copies; 5th cycle: 32 copies etc. → After 30 cycles, there are 1,073,741,824 III. DNA Sequencing and Phylogenetic Analysis Sanger Sequencing - Retaining entire nucleotide sequence of an organism. Also called di-deoxynucleotide sequencing. - Pure template sequence is PCR amplified in the presence of appropriate primers and dNTPs, as well as modified bases called di-deoxynucleotides (ddNTPs) → lack a hydroxyl group of deoxynucleotides which limit their ability to form phosphodiester bonds with adjacent nucleotides. → each deoxynucleotide is also labeled with a different fluorescent label to make its detection easier. sequencing from different species or group of individuals → Also known as High throughput sequencing → High proprietary technology → Sequences DNA and RNA in a rapid and cost-effective manner. → can be used for population genetics and clinical studies → fast and cost-efficient Most popular: Reversible Terminator sequencing Others: Pyrosequencing, sequencing by ligation Steps → Fragmentation - Genomic DNA is cut into smaller fragments of 100-1000 base pairs → Ligation of DNA Adapters adapters called Special oligonucleotide sequences with primer sets then ligated to both ends of DNA fragments 1. Denature template DNA into a single-stranded DNA 2. AS primers bind to region of inherit, DNA polymerase starts at a new nucleotide to the DNA strand and occasionally operates a ddNTP instead of a dNTP - Terminates DNA amplification - DNA fragment of varying lengths = each termination with a ddNTPs DNA fragments are run on a capillary gel to separate them on the basis of size Emission spectra of each of these fragments are analyzed through software Next-Generation Sequencing - collective name for group of technologies that can facilitate the relatively rapid generation of → Binding to Chip (Flow Cell) - The single-stranded DNA fragments are loaded to specialized chips called a flow cell with short ○ Precoated oligonucleotide sequences DNA fragments bind to the chip through their adapters. → Denaturation and DNA Amplification - in the amplification stage, DNA polymerase is used to synthesize DNA of a complementary strand. → Washing - The double stranded DNA is denatured and the original template washes off → Bridge Building Remaining complementary strand bends over and forms a bridge-like strand using the second adapter to the other end. DNA polymerase can then synthesize the complementary strand of the bent DNA strand in a process called Bridge amplification Double stranded is again denatured to form a single stranded DNA DNA polymerase continues cycles of bridge amplification, synthesizing thousands of copies of the DNA fragment and resulting to clonal clusters → Cleaving of reverse strand - all reverse complementary strands are then washed off the chip leaving only forward DNA strands immobilized on the chip. → Sequencing Begins with DNA polymerase, adding modified dNTPs with four different fluorescent labels ○ Also contains three prime blocking mostly called reversible terminator ○ Only allows for the addition of one dNTP at a time After each round of dNTp addition to the growing strand, chip is imaged and the emission wavelength from each DNA cluster is used to identify the base The sequence of fluorescent images of the flow cell capture each dNTP addition is used to determine the sequence of DNA fragments Phylogenetic Tree - depict evolutionary relationships among organisms. The relationships take the form of a tree Tips - represent extant or lying taxa Branches - denote evolutionary changes between ancestors and descendants such as change in DNA sequence or evolution of characteristic Sister Taxa - groups that share an immediate common ancestor are their closest relatives Node - most common recent ancestor Basal Node - correspond to most common recent ancestor of all organisms in the tree Group organisms that are descendants of a common ancestor Monophyletic group or clade - when a group contains the most recent common ancestor and all of its descendants. Paraphyletic - includes a common ancestral species and some of its descendants. Polyphyletic - does not share an immediate common ancestor. Relationships can be determined morphological or genetic features. from Maximum parsimony - assumes the least amount of change between organisms. Maximum likelihood - not all changes are equally likely; can be based on the most likely scenario from observed organisms. IV. Multi-locus sequence typing (MLST) unambiguous procedure characterize bacterial isolates into known groups. Identify alleles they possess from established housekeeping genes and designing isolates to sequence types accordingly For each different kind of bacterium you have a specific MLST scheme → Most commonly used for disease epidemiology to track outbreaks, → Does not look at the total sequence similarity bin strains - Rather: each sequence for a given locus is screened for identity with already known sequences of that locus → If that sequence is different, it is considered a new allele and is assigned an allele number → 7 housekeeping genes studied: each strain is characterized by a profile of 7 allele numbers →Study population genetics and reconstruct microevolution of epidemic bacteria and other microorganisms. PHAGE GENETICS Bacteriophages Virus, “deadliest being” Most abundant and widely distributed organisms on earth Discovered by Fredrick William Twort and Felix d'Hérelle (20th Century). Specifically infect bacteria Structure of a phage Head or capsid ○ Contains genetic material (nucleic acid) ○ Shape is icosahedral Tail ○ Contractile ○ Injects the nucleic acid to target bacteria Lytic Cycle Attachment ○ Attach tail fibers to host cell Penetration ○ Open cell wall using lysozyme ○ Injects nucleic acid to host Biosynthesis ○ Production of phage DNA and proteins Assembly/Maturation ○ Assembly of phage particles into one complete infectious virus: virion Release ○ Break cell wall to release phage ○ Endolysin e.g. lysozyme ○ Bacteriophages in Microbial Genetics Mainly used as a model to explore the mechanisms of life and led to the birth of modern molecular biology One classical example is the demonstration of a central biological question in the 20s: The Hershey-Chase Experiments (1952) → The Hershey-Chase Experiments (1952): In 1952, Alfred Hershey and Martha Chase conducted a series of experiments to confirm that DNA is the genetic material in living organisms. The experiments involved infecting bacteria with bacteriophages, which are made of DNA and protein, and labeling the protein and DNA with radioactive isotopes. The results showed that the DNA entered the bacterial cell, but most of the protein did not, and that the protein remained attached to the cell membrane. This led Hershey and Chase to conclude that genetic information is DNA, not proteins Lysogenic Cycle Attachment Penetration/Entry Integration ○ Phage DNA recombines with bacterial chromosome ○ Prophage - bacteriophage genome integrated into the host genome Cell Division Phage DNA copied along with bacteria When is it Lytic/lysogenic ○ Phage: host ○ UB ○ Chemicals ○ Spontaneous Considered for development of strategies to treat infections Lagged behind because of the discovery of antibiotics ○ Effective ○ Greater breadth and potency ○ E.g. Penicillin led to mass production However discovery and overuse of antibiotics led to antibiotic resistance and the development of superbugs. Antibiotics: carpet bombing Phages: high specificity; personalized guided missiles. How does antibiotic resistance develop? Do bacteria adapt to phages as well? Bacteriophages Made a comeback Emergence of multi-antibiotic resistant bacteria and low rate of new antibiotic discovery brought the new urgency to develop phage-based therapies. Phage therapies against: E.g. Pseudomonas aeruginosa and Acinetobacter baumannii Applications of Phage Therapies 1. Treatment of bacterial infections and wounds. 2. Skincare products 3. Preventing biofilms on medical devices. 4. Anti-tumor 5. Veterinary medicine