Bio 10.1 - 10.2: Pre-Implantation Development PDF
Document Details
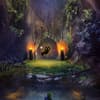
Uploaded by iiScholar
Arizona State University
Tags
Summary
This document provides a detailed overview of pre-implantation development, including the processes of fertilization and blastulation. It covers important concepts like oocyte, sperm and zygote with accompanying diagrams.
Full Transcript
Chapter 10: Pregnancy, Development, and Aging 336 Lesson 10.1 **Pre-Implantation Development** Introduction Embryonic development begins when male and female gametes join to form a zygote (ie, fertilization). Although fertilization typically takes place in a uterine tube within 12--24 hours aft...
Chapter 10: Pregnancy, Development, and Aging 336 Lesson 10.1 **Pre-Implantation Development** Introduction Embryonic development begins when male and female gametes join to form a zygote (ie, fertilization). Although fertilization typically takes place in a uterine tube within 12--24 hours after ovulation, the developing embryo must then travel to the uterine cavity and implant for a viable pregnancy to occur. The developmental steps between fertilization and implantation take place within 12 days after ovulation. This lesson covers **pre-implantation development** from the time of fertilization until the completion of implantation, as well as development of the **placenta** (ie, transient organ which mediates fetal-maternal gas and nutrient exchange). 10.1.01 Fertilization and Blastulation **Fertilization** (ie, joining of male and female gametes to form a zygote) becomes possible with the release of the secondary oocyte from the ovary (ie, **ovulation**). Upon ovulation, the oocyte travels into the abdominal cavity and is drawn into the **uterine (Fallopian) tube** by densely ciliated projections known as **fimbriae**. The ciliated lining of the uterine tube helps propel the oocyte into the uterine cavity. Sperm typically make contact with the oocyte within the uterine tube. Successful fertilization requires the following steps, as outlined in Figure 10.1: 1.**Capacitation:** To reach the oocyte, sperm must undergo a final maturation step in the uterine tube; this step is known as capacitation. Female reproductive tract secretions induce changes in plasma membrane permeability at the sperm head and trigger increased flagellar movement (ie, motility). 2.**Contact with oocyte:** The sperm moves past follicular cells of the oocyte\'s **corona radiata**, toward the **zona pellucida** (ie, thick matrix of glycoproteins between the corona radiata and plasma membrane) to reach the oocyte. Receptors located in the sperm head bind to glycoproteins in the zona pellucida. 3.**Acrosome reaction:** Located within the sperm head, the acrosome is a specialized vesicle filled with hydrolytic enzymes (see Concept 9.2.02). These enzymes are released near the oocyte, leading to zona pellucida degradation, thereby enabling the sperm to reach the oocyte\'s plasma membrane. 4.**Fusion:** Oocyte and sperm plasma membranes fuse, triggering depolarization of the oocyte\'s plasma membrane. 5.**Sperm contents entering the oocyte:** The sperm nucleus, mitochondria, and a pair of centrioles enter the oocyte. However, most sperm mitochondria are destroyed following oocyte entry (see Concept 7.3.06). 6.**Cortical reaction:** Upon sperm fusion, oocyte plasma membrane depolarization leads to increased intracellular calcium (Ca2+) levels, which triggers **cortical granules** (ie, secretory vesicles containing hydrolytic enzymes) to fuse with the oocyte\'s plasma membrane. Hydrolytic enzymes are released into the space between the plasma membrane and the zona pellucida, causing the zona pellucida to lift away from the oocyte and harden. The cortical reaction results in the formation of a protective envelope that blocks additional sperm from entering (ie, **polyspermy**). Chapter 10: Pregnancy, Development, and Aging 337 **Figure 10.1** Fertilization sequence. Following sperm and oocyte plasma membrane fusion, sperm contents can enter the oocyte. The secondary oocyte must then complete [meiosis II](javascript:void(0)), dividing to form a mature ovum and second polar body, which degenerates (see Concept 9.3.02). The nucleus of the mature ovum develops into a **female pronucleus**. Simultaneously, the **male pronucleus** (ie, sperm nucleus) is propelled toward the female pronucleus. Fusion of haploid (1*n*) pronuclei produces a diploid (2*n*) cell known as a **zygote**. Figure 10.2 provides an overview of the events of spermatogenesis and oogenesis, which culminate in fertilization and zygote production. Diagram of a cell cycle Description automatically generated with medium confidence Chapter 10: Pregnancy, Development, and Aging 338 **Figure 10.2** Overview of gametogenesis and fertilization. Immediately following zygote formation, epigenetic modifications (eg, [chromatin remodeling](javascript:void(0)), [DNA](javascript:void(0)) [methylation](javascript:void(0)) changes) occur and allow for developmental [totipotency](javascript:void(0)), the ability to give rise to all embryonic cell types. Notably, some parental epigenetic modifications (eg, imprinted genes) are maintained (see Concept 10.3.02). Within 24 hours after fertilization, the zygote begins a period of rapid [mitotic cell division](javascript:void(0)) known as embryonic **cleavage** (Figure 10.3). During cleavage, cell division proceeds without concurrent cell growth  Chapter 10: Pregnancy, Development, and Aging (ie, daughter cells become progressively smaller compared to the zygote), forming a mass of identical cells known as **blastomeres**. After 3--4 days, the embryo enters the **morula** stage. The morula, roughly the same size as the original zygote, consists of 16--32 blastomeres. During the transition from zygote to morula, the developing embryo travels from the uterine tube towards the uterine cavity. By days 4--5, the rapidly dividing embryonic cells reorganize into a **blastocyst**, which contains a hollow, fluid-filled cavity known as the **blastocoel**. The blastocyst contains two distinct cell populations: an **inner cell mass**, which contains cells that develop into the embryo, and a **trophoblast**, the outer cell layer that develops into extraembryonic tissues (eg, placenta). **Figure 10.3** Early embryonic development from zygote to blastocyst. **Concept Check 10.1** For successful fertilization of the oocyte by the sperm, a series of steps must occur in sequence. Using the following list, provide a rationale for why unsuccessful completion of each step could lead to a failed fertilization sequence: 1.Capacitation 2.Acrosome reaction 3.Cortical reaction [**Solution**](javascript:void(0)) Diagram of a cell structure Description automatically generated  Chapter 10: Pregnancy, Development, and Aging 340 10.1.02 Implantation and Placental Development For development to continue beyond the blastocyst stage, the blastocyst must implant into the uterine lining (Figure 10.4). By 6--7 days after fertilization, surges in progesterone and estrogen trigger the secretory phase of the uterine cycle, and the endometrial lining is primed to support **implantation** of the blastocyst (see Concept 9.3.03). Developing trophoblastic cells (ie, cells from the outer portion of the blastocyst) adhere to the endometrial surface, causing the blastocyst to burrow into the endometrial lining. Endometrial cells proliferate and surround the blastocyst, now known as the **embryo**, sealing it off from the uterine cavity. Until the placenta is completely formed, the endometrium supports and nourishes the developing embryo. **Figure 10.4** Blastocyst implantation. The process of implantation is usually complete by day 12. If fertilization does not occur, the corpus luteum typically degenerates, and declining progesterone and estrogen levels trigger menstruation. However, when successful implantation occurs, the developing trophoblastic cells begin to secrete the hormone **human chorionic gonadotropin (hCG)**, and the corpus luteum is maintained while the placenta develops. The **placenta** forms over the first 3 months of pregnancy to facilitate nutrient, gas, and waste exchange between the maternal circulation and the developing embryo. An extraembryonic membrane known as the **chorion** is derived from the trophoblast following implantation. **Chorionic villi**, fingerlike projections that invade the endometrium, form from the chorion and become vascularized. The chorion and chorionic villi form the bulk of the placenta (Figure 10.5). The corpus luteum continues to secrete estrogen and progesterone for approximately the first 9 weeks of pregnancy, effectively inhibiting the initiation of another menstrual cycle. However, the developing placenta gradually takes over secretion of hCG, estrogens, and progesterone to support the pregnancy, leading to corpus luteum degeneration. Diagram of a cell cycle Description automatically generated Chapter 10: Pregnancy, Development, and Aging **Figure 10.5** Developing placenta and extraembryonic structures. The embryo is supported by several extraembryonic structures derived largely from the blastocyst inner cell mass before and during placenta formation (Figure 10.5): **Yolk sac:** a region where maternal-fetal nutrient and gas exchange occurs prior to placenta formation and the main site of embryonic blood cell production prior to the fetal period. **Allantois:** formed from the yolk sac, a structure that mediates fluid and waste exchange between the embryo and yolk sac. The umbilical cord is eventually formed from the yolk sac and allantois. **Amnion:** a tough membrane filled with amniotic fluid that surrounds the embryo. Once fully developed, the placenta receives blood from both the maternal and the fetal circulatory systems, although these two systems do not typically intermix (see Concept 10.4.02). Automatic ZoomActual SizePage Width100%50%75%100%125%150%200%300%400% Chapter 10: Pregnancy, Development, and Aging 342 Lesson 10.2 **Post-Implantation Development** Introduction Successful implantation of the blastocyst signals the beginning of the embryonic period of development. **Organogenesis** (ie, the development of embryonic tissues and organs) begins with **gastrulation**, a period of rapid growth and reorganization of embryonic cells. By the end of gastrulation, the three primary germ layers are established, from which all embryonic tissues and organs are formed. Shortly thereafter, mesodermal tissue induces the overlying ectoderm to become neural tissue, in a process known as **neurulation**. By the end of the embryonic period, the development of all major body systems is well underway and continues throughout the remainder of the pregnancy. This lesson covers the major events of post-implantation development during the embryonic period. More information about the fetal period, along with other aspects of pregnancy and parturition, can be found in Lesson 10.4. 10.2.01 Gastrulation At the time of implantation, the blastocyst consists of two major cell types: trophoblast cells and the inner cell mass (ICM). Before gastrulation, the ICM cells are organized into two cell types: the **epiblast** (upper layer) and **hypoblast** (lower layer). A bilaminar (ie, two-layered) disk, consisting of a portion of the epiblast and the hypoblast, eventually becomes the embryo proper. The cells of the epiblast form the embryo and amnion, and the cells of the hypoblast form a portion of the chorion and the yolk sac, structures that are discussed in more detail in Concept 10.1.02. During week three of development, ICM cells undergo a period of rapid growth, differentiation, and cellular rearrangement known as **gastrulation**, establishing the three **primary germ layers** from which all embryonic tissues and organs arise: ectoderm, mesoderm, and endoderm. Gastrulation begins with the formation of a groove known as the **primitive streak** within the epiblast which establishes the anterior-posterior axis of the embryo. Along the primitive streak, cells that will form the internal organs migrate into the interior space of the embryo, and cells that will form the skin and nervous system migrate along the outer surface. The process of gastrulation is illustrated in Figure 10.6. Chapter 10: Pregnancy, Development, and Aging 343 **Figure 10.6** Gastrulation. The **blastopore**, or first point of invagination during gastrulation, forms as endodermal cells invaginate at the posterior end of the primitive streak. In mammals, the blastopore eventually becomes the anal opening of the embryo. As development continues, further migration of endodermal cells creates a primitive gut cavity called the **archenteron**. As the three primary germ layers are formed, the relatively flat embryonic disk also undergoes significant changes in size and shape. Because of asynchronous rates of growth and cell movement, the embryo folds into a three-dimensional form in which ectodermal cells are largely found on the exterior of the embryo, endodermal cells are found on the interior, and mesodermal cells are found in between (Figure 10.6). Gastrulation results in a three-layered embryo called a **gastrula**. Following gastrulation, the three primary germ layers have different fates, as depicted in Figure 10.7: The **ectoderm** develops into the skin and nervous system. The **mesoderm** gives rise to muscle, bone, and other connective tissues. The **endoderm** forms the lining of the digestive tract and other internal organs.  Chapter 10: Pregnancy, Development, and Aging 344 **Figure 10.7** Germ layer derivatives. A more comprehensive list of germ layer derivatives is included in Table 10.1. Note: Although it is unlikely that every germ layer derivative must be memorized, past exams have included highly specific questions regarding germ layer derivatives, with particular attention to counterintuitive examples (eg, adrenal medulla is derived from ectoderm, whereas adrenal cortex is derived from endoderm). A diagram of a human body Description automatically generated Chapter 10: Pregnancy, Development, and Aging 345 **Table 10.1** Detailed derivatives of the three primary germ layers. 10.2.02 Organogenesis and Neurulation The formation of specific [tissues](javascript:void(0)) and organ systems begins after primary germ layer establishment. During **organogenesis**, progressive cell differentiation turns the relatively undifferentiated primary germ layers into functional organs and organ systems. Exposure to signaling molecules from an underlying mesodermal structure called the **notochord** induces overlying ectodermal cells to form neural tissues in a process called **neurulation**. Three ectodermal cell types arise during neurulation: **epidermal** cells (eg, the outer layer of the [skin](javascript:void(0))), **neural crest** cells (eg, precursor cells of the [peripheral nervous system](javascript:void(0)) \[PNS\], [melanocytes](javascript:void(0))) and **neural plate** cells (eg, precursor cells of the central nervous system \[CNS\]). At this stage of embryogenesis, the embryo is called a **neurula**. Once ectodermal cell types are specified, neurulation continues in the neural plate via the following steps (Figure 10.8): 1.Thickening and elongation of the neural plate in response to additional signals from the underlying mesoderm. 2.Upward movement of the edges of the neural plate to form **neural folds** on either side of a central **neural groove**. 3.Migration of lateral edges of the neural folds toward the midline of the embryo, fusing to form a **neural tube** that sits inferior to an overlying ectodermal layer. Neural crest cells **delaminate** (ie, detach) from the neural tube as the tube closes and these cells migrate to various regions throughout the embryo.  Chapter 10: Pregnancy, Development, and Aging 346 **Figure 10.8** Neurulation. Neural crest cells differentiate into a number of diverse cell types, most notably the neurons and [glia](javascript:void(0)) of the PNS (eg, sensory and autonomic ganglia, adrenal medulla, Schwann cells), melanocytes (pigment cells), [calcitonin-producing thyroid cells](javascript:void(0)), and facial connective tissue. After neural tube formation, organogenesis continues with the segmentation of mesodermal tissues adjacent to the neural tube into **somites**, clusters of muscle and connective tissue precursor cells found in the back (eg, precursors to vertebrae, ribs, dermis). Concurrently, tissues derived from nearby mesoderm and endoderm give rise to the remaining organs and organ systems. See Concept 10.2.01 for more details about the structures derived from each germ layer. A progression of the stages of pre- and post-implantation development is shown in Figure 10.9. A diagram of the structure of the human body Description automatically generated