Er-Yb All-Fiber Laser With a Repetition Rate (PDF)
Document Details
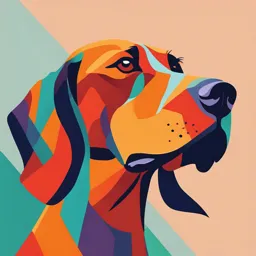
Uploaded by YoungIndianArt7416
2024
Andrei Zverev, Vladimir Kamynin, Vladimir Tsvetkov, Boris Denker, Sergey Sverchkov, Vladimir Vel'miskin, Yuri Gladush, Dmitry Krasnikov, Albert Nasibulin
Tags
Summary
This research paper details the optimization of a short cavity passively mode-locked erbium-ytterbium fiber laser. The laser, featuring a composite active fiber and a hybrid isolator-coupler-multiplexer, incorporates aerosol-synthesized single-walled carbon nanotubes. The study focused on increasing the pulse repetition rate and optimizing the laser's performance.
Full Transcript
Optical Fiber Technology 88 (2024) 104007 Contents lists available at ScienceDirect Optical Fiber Technology...
Optical Fiber Technology 88 (2024) 104007 Contents lists available at ScienceDirect Optical Fiber Technology journal homepage: www.elsevier.com/locate/yofte Er-Yb all-fiber laser with a repetition rate for ultrashort pulses of 300 MHz Andrei Zverev a,* , Vladimir Kamynin a , Vladimir Tsvetkov a, Boris Denker a , Sergey Sverchkov a , Vladimir Vel’miskin b, Yuri Gladush c , Dmitry Krasnikov c , Albert Nasibulin c a Prokhorov General Physics Institute of the Russian Academy of Sciences, Moscow, Russia b Prokhorov General Physics Institute, Dianov Fiber Optics Research Center, Russian Academy of Sciences, Moscow, Russia c Skolkovo Institute of Science and Technology, Moscow, Russia A R T I C L E I N F O A B S T R A C T Keywords: We demonstrate the optimization of a short cavity passively mode-locked (ML) erbium-ytterbium fiber laser. The Ultrashort pulses cavity consisted of a composite active fiber and a hybrid isolator-coupler-multiplexer. To realize the ML regime, Composite fiber aerosol-synthesized single-walled carbon nanotubes were placed in the cavity. During length optimization, the Short cavity pulse repetition rate was increased from 100 to 298 MHz. At the highest repetition rate, the optical pulses had a duration of 3.6 ps and the average output power was 1.6 mW. 1. Introduction Consequently, the former approach is frequently employed to generate USP sources with a high repetition rate and good stability. Fiber lasers have long established themselves as a reliable tool for Lasers with a short length are typically created with a Fabry-Perot research in such areas as medicine, biology, materials, etc. [1,2,3,4,5]. (FP) cavity. In the case of standard active fibers, it was possible to Passive mode-locked (ML) fiber lasers are widely used as sources of obtain ultrashort pulses with a repetition rate of 250 MHz. How ultrashort pulses (USPs) for optical ADCs , materials processing ever, standard active fibers exhibit relatively low amplification, with a systems , supercontinuum generators , etc. Several strategies have gain of no more than 0.03 dB/cm. Consequently, their length within the been developed to achieve passive ML: the use of the nonlinear rotation laser cavity reaches several tens of centimeters, which precludes the of the polarization plane (NRPP) effect , the use of nonlinear loop attainment of the fundamental repetition rate of the USP at a signifi mirrors , saturable absorbers such as graphene , topological cantly higher value than 100 MHz. To address this issue, heavily doped insulators , carbon nanotubes , semiconductor mirrors (SESAM) phosphate fibers have recently emerged as a promising solution, because , etc. the concentration of active ions in these fibers is approximately one In recent years, there has been a growing interest in compact fiber order of magnitude higher than in standard fibers. It results in a sig lasers with sub-GHz and GHz USP repetition rates. These lasers are in nificant reduction in the length of the active fiber within the laser. Based demand for various applications, including terahertz radiation sources on these fibers, repetition rates of more than 10 GHz were obtained in , promising broadband telecommunication systems , in which the FP cavity. However, these fibers exhibit poor splicing charac the data transmission rate is directly proportional to the repetition rate teristics with standard optical components and are susceptible to of ultrashort pulses, and high-resolution microscopy , where the degradation in air, which results in elevated intracavity losses and high pulse repetition rate allows the observation of fast dynamic pro compromises the stability of laser systems. In summary, the principal cesses, and others. A high repetition rate can be achieved by a significant disadvantage of FP lasers is the necessity for mechanical connections in reduction of the cavity length or by implementing harmonic mode- the cavities and the resulting bulky elements. These facts significantly locking. The latter approach has several drawbacks, such as reduce the long-term stability and usability of such lasers. instability of the pulse repetition rate and poor repeatability. Although An alternative approach to achieving a high pulse repetition rate recent studies present methods that reduce these drawbacks, their within an all-fiber design is the application of ring cavities. A ring fiber implementation, however, involves the integration of bulk fiber laser operating in the passive mode-locking (ML) mode typically in stretchers into resonators and requires precise system tuning. cludes the following components: wavelength division multiplexing * Corresponding author. E-mail address: [email protected] (A. Zverev). https://doi.org/10.1016/j.yofte.2024.104007 Received 1 August 2024; Received in revised form 24 September 2024; Accepted 10 October 2024 Available online 17 October 2024 1068-5200/© 2024 Elsevier Inc. All rights are reserved, including those for text and data mining, AI training, and similar technologies. A. Zverev et al. Optical Fiber Technology 88 (2024) 104007 (WDM), insulator, and coupler. In 1997, Lerminaux and Maschmeyer glass. The process began with synthesizing the glass when using pre proposed the concept of integrating the WDM, isolator, and tap (WIT) cursor materials such as ErF3 (0.75 mol%), YbF3 (1.25 mol%), La (PO3)3 into a single device. In recent years, compact fiber hybrid (WIT) (11 mol%), LiF (17 mol%), B2O3 (12 mol%), Al2O3 (6 mol%) and P2O5 components have also been developed, which permits a further reduc (52 mol%). The addition of boron and aluminum oxides to the phos tion in cavity length while maintaining an all-fiber design. This increases phate glass improved its thermomechanical properties and moisture the stability of operation, reduces intracavity losses, and allows the stability, became comparable to silicate glasses. The glass synthesis avoidance of the linear cavity scheme typically employed in the creation was carried out at high temperatures (1350 ◦ C) in an inductively heated of USP sources with a high repetition rate [25,26]. platinum crucible under a controlled dry atmosphere for 10 h. Addi In this study, we investigated a ring cavity based on a hybrid WIT in tional desiccant in the form of phosphorus pentoxide was used to combination with a perspective composite erbium-ytterbium-doped maintain a dry atmosphere. The synthesized glass had a low content of fiber (EYDF). Unlike the fibers mentioned in references [23,25], these residual OH-groups. fibers are composed of a silica glass cladding and a phosphate glass core Then we formed the rods from the bulk synthesized glass by drilling doped with rare-earth ions, combining the advantages of both silica and and subsequently etching to remove defects and impurities coming after phosphate fibers (it has high gain, does not degrade in air, and connects mechanical treatment. These rods were placed into silica glass tubes, well with standard optical components). To achieve the ML regime, and the assembly was drawn in a high-temperature induction furnace to we employed polymer-free thin films of aerosol-synthesized single- create the composite fiber preform with a core containing phosphor walled carbon nanotubes (SWCNTs) as a saturable absorber (SA). This osilicate glass. Fiber preforms were made with Heraeus F300 silica glass type of absorber offers broad application possibilities due to variation of tubes, and optical fibers were drawn from these preforms at high tem the optical spectrum features and demonstrates great promise for mode- peratures. The fibers were finally coated with a polymer coating. The locking due to the easy of placement in the cavity and its ability to specific fiber being investigated in this work had a standard outer overcome the degradation issues associated with conventional CNTs diameter of 125 μm and was designed as a strictly single-mode fiber for embedded in a polymer matrix. So, it is the promising SA for high the telecom wavelength range. It’s worth noting that a previous study repetition rate USP sources. In our research, we successfully demon reported a multimode Er/Yb-doped composite fiber. Due to the strated a laser utilizing such carbon nanotubes, hybrid element and composite structure, this fiber had high optical gain, mechanical resis unique composite erbium-doped fiber for the first time, achieving a tance and a good compatibility with standard silica fibers. In this case, fundamental pulse repetition rate exceeding 250 MHz in all-fiber ring the splicing losses with such a fiber did not exceed 1 dB. The absorption cavity. at wavelengths of 976 nm (pump) and 1535 nm was approximately 1500 dB/m and 170 dB/m, respectively. At the same time, the specific 2. Experimental setup gain of the weak signal (Erbium ASE) was 78.5 dB/m (these parameters were obtained from the study of the fiber’s absorption and gain spectra). The scheme of the composite EYDF laser is shown in Fig. 1. We use The length of the active fiber was 12 cm. The composite fiber was the composite fiber with a phosphate glass core with a high concentra pumped by the laser diode at a wavelength of 976 nm through a hybrid tion of Er/Yb ions and a silica glass cladding as an active medium of the (WIT), that plays the role of a wavelength division multiplexer, an laser. The fabrication of this fiber involved the application of the “rod- isolator and a tap. The coupler split coefficient was 85/15 (15 % yield). in-tube” technique. As a result, the laser cavity consisted of standard single-mode fiber. The initial material being used was Yb-Er-doped phosphate laser To achieve mode-locking, we implemented a method involving the incorporation of a two layer of polymer-free single-walled carbon nanotube (SWCNT) films. These films, approximately 60 nm thick, were placed between the fiber ends in a standard FC fiber connector. The SWCNTs being utilized in the experiment were synthesized using the aerosol (floating catalyst) chemical vapor deposition (CVD) technique [31,32]. During the growth process, SWCNTs were formed on the sur face of iron particles suspended in a CO/CO2 atmosphere, and the resulting thin film was collected on a nitrocellulose filter. Subsequently, the SWCNT films were transferred from the filter to the desired substrate using a simple dry transfer technique [33,34]. One polarization controller (PC) was used for tuning the laser generation mode. The modulation depth of one layer of absorbers, as was shown in , was 0.7 %. In this work, two layers were used, which corresponded to a modulation depth of 1.4 %. 3. Results and discussion We conducted a series of experiments in which we gradually reduced the length of the cavity to 0.6 m, while the active fiber length stayed unchanged. Initially, the length of the cavity was 1.93 m. A stable passive mode- locking was obtained at a pump power of 300 mW. The laser generated ultrashort pulses with a repetition rate of 103.5 MHz and a duration of 4.5 ps (Fig. 2 (a)) with a central wavelength of 1535 nm. The spectrum FWHM was 1 nm (Fig. 2 (b)). The output power was 0.48 mW. With a decrease in the cavity length, stable lasing modes were also obtained with ultrashort pulse repetition frequencies of 129 MHz (cavity length Lr = 1.54 m), 153 MHz (Lr = 1.3 m), 214 MHz (Lr = 0.93 m), 247 Fig. 1. Scheme of an Erbium-doped fiber laser. SWCNT − Connected ferrule MHz (Lr = 0.81 m). The parameters of the obtained generation modes with a transferred SWCNT thin films. PC − polarization controller. are listed in Table 1. 2 A. Zverev et al. Optical Fiber Technology 88 (2024) 104007 Length of cavity1.93 m laser generated ultrashort pulses with a repetition rate of 298.5 MHz (RF 1 spectrum of the signal is shown on the Fig. 3 (d)) and a duration of 1.6 ps 1.0 a) b) at a central wavelength of 1543 nm. The spectrum width was 1.3 nm Intensity, mW Intensity, a.o. 0.8 0.1 (Fig. 3 (c), (d)). The shortwave peak contained less than one percent of 0.6 4.5 ps * 1.53 0.01 the output power. It is also worth noting that increasing the pump power 0.4 0.001 led to the appearance of harmonic mode-locking regimes with doubled 0.2 and tripled pulse repetition frequencies, but they were not stable enough 0.0 -12 -8 -4 0 4 8 12 1E-4 1532 1534 1536 1538 to be recorded. The study successfully demonstrated the possibility of creating high- Time, ps Wavelength, nm pulse repetition rate sources of ultrashort pulses using an active com Fig. 2. Autocorrelation trace (a) and spectra (b) of output radiation. posite fiber. By reducing the passive fiber length and increasing the pump power from 300 to 664 mW, stable lasing states with ultrashort pulse repetition rates ranging from 103 to 247 MHz were achieved. The Table 1 cavity lengths were controlled by direct measurements and additionally Lists the lengths of the cavity, pump power, and parameters of the output ra verified by calculations based on the fundamental pulse repetition rate diation for the implementation of stable modes of usp generation. using the formula: L = v/υ, where L is the resonator length, v is the speed Cavity length, m 1.93 1.54 1.3 0.93 0.81 0.67 of light in the fiber, and υ is the pulse repetition frequency. With USP repetition 103.51 129.79 153.83 214.37 246.94 298.49 approximately the same pulse repetition rate in our work (214 MHz) and rates, MHz in (199 MHz), our system exhibits a lower signal-to-noise ratio (S/ Pump power, mW 300 315 520 664 656 365 N). However, further reduction of splice losses, which were automati Output power, 0.48 0.77 1.1 1.3 2.16 1.64 cally determined by the splicing machine, and minimization of the mW Central 1535.4 1535.3 1535.2 1535.4 1542 1542.8 cavity length led to an increase in pulse repetition rate to 298 MHz and wavelength, nm S/N to 70 dB, whereas in , with an increase in harmonic number, the Spectrum width, 1 1.1 1.7 1.3 1.6 1.3 S/N decreases and does not exceed 60 dB. The discrepancy in the output nm power and pump power necessary to achieve a stable ML can be Duration ACT, ps 6.91 6.57 5.23 6.79 2.4 3.6 attributed to the fluctuations in losses (ranging from 0.2 to 1 dB) S/N, dB 50 60 55 45 75 70 TBP 0.57 0.6 0.73 0.73 0.316 0.38 resulting from the splicing of a standard optical fiber with the active one. Long-term + Table 1 illustrates that the laser can be operated in two wavelength stability regions, namely 1535 and 1542 nm. The presence of such a structure in Self-start + the generation spectrum can be attributed to the transmission of the few- mode active fiber-single-mode fiber complex, which is analogous to the Finally, the total length was reduced to 0.67 m. So, the passive fiber configuration described in. The presence of such a spectral filter in length was of 0.55 m. During cavity length reduction we made new the resonator also limits the generation bandwidth and leads to the splice connections. New splices make it possible to obtain stable ML at a generation of relatively long picosecond pulses. pump power of 365 mW. Output power in this case was of 1.64 mW. The In all cases, the signal spectra exhibited a satisfactory fit with the 1.0 a) 1 Measured b) spectrum Sech2 0.8 fitting Intensity, mW 0.1 Intensity, a.u. 0.6 2.3 ps * 1.53 0.01 30 dB 0.4 0.001 0.2 0.0 1E-4 -9 -6 -3 0 3 6 9 1530 1540 1550 Time, ps Wavelength, nm 20 c) 0 d) 0 Intensity, dB S/N>60 dB Intensity, dB -20 -20 -40 -40 -60 -60 -80 -80 296 297 298 299 300 301 Frequency, MHz Frequency, GHz Fig. 3. (a) – autocorrelation trace; (b) – spectrum; (c), (d) – radio frequency spectrum. 3 A. Zverev et al. Optical Fiber Technology 88 (2024) 104007 sech2 function, thereby indicating the generation of soliton pulses. Data availability Nevertheless, the occurrence of frequency modulation in the pulses, as evidenced by a time-bandwidth product (TBP) exceeding 0.315, is Data will be made available on request. explained by intracavity losses and dispersion interactions between optical components. References Following polarization and current tuning, self-starting was observed for all presented repetition rates. The signal-to-noise ratio exceeded 45 M.S. Kopyeva, S.A. Filatova, V.A. Kamynin, A.I. Trikshev, E.I. Kozlikina, V. V. Astashov, V.B. Tsvetkov, Appl. Sci. 12 (8) (2022) 3825, https://doi.org/ dB for all cavity lengths, reaching 75 dB at 246.9 MHz. These observa 10.3390/app12083825. tions indicate that the achieved ML regimes are highly stable. B. Shiner, (2005, March). Fiber lasers for material processing. In Critical Review: In contrast to numerous fiber lasers with pulse repetition rates Industrial Lasers and Applications (Vol. 5706, pp. 60-68). SPIE. 10.1117/12.601648. Y. Chen, Z. Chen, T. Li, M. Qiu, J. Zhang, Y. Wang, H. Zhang, ACS Nano 17 (13) exceeding 100 MHz, our laser employs an all-fiber design, providing (2023) 12903–12914, https://doi.org/10.1021/acsnano.3c05007. higher mechanical stability and better manufacturability. Furthermore, Z. Chen, J. Li, T. Li, T. Fan, C. Meng, C. Li, H. Zhang, Natl. Sci. Rev. 9 (8) (2022) our experiment demonstrates the potential of the presented fiber. In this nwac104, https://doi.org/10.1093/nsr/nwac104. Z. Chen, C. Meng, X. Wang, J. Chen, J. Deng, T. Fan, H. Zhang, Laser Photonics setup, the absolute losses are estimated to be 2.5 dB. According to our Rev. (2024), https://doi.org/10.1002/lpor.202400035, 2400035. estimates, 4 cm of active fiber is sufficient to compensate for these losses S. Kobtsev, S. Smirnov, S. Kukarin, S. Turitsyn, Opt. Fiber Technol. 20 (2014) 615, and generate pulses at higher pump power. Considering the splice https://doi.org/10.1016/j.yofte.2014.07.009. G.C. Valley, Opt. Express 15 (5) (2007) 1955–1982, https://doi.org/10.1364/ points, the length of the SMF fiber can be reduced to 15 cm. Thus, the OE.15.001955. expected maximum pulse repetition frequency could exceed 1 GHz. H. Kalaycıoğlu, P. Elahi, et al., IEEE J. Sel. Top. Quantum Electron. 24 (3) (2017) Interestingly, the pump power values at high pulse repetition rates are 1–12, https://doi.org/10.1109/JSTQE.2017.2771745. relatively low. In most known laser systems, the pump power exceeds G. Sobon, et al., Opt. Mater. Exp. 4 (1) (2014) 7–15, https://doi.org/10.1364/ NLO.2013.NM3A.6. 500 mW. Further optimization of the fiber geometry and welding pro V.J. Matsas, T.P. Newson, D.J. Richardson, D.N. Payne, Electron. Lett. 28 (1992) cess will enhance efficiency and laser performance. It would also be 1391, https://doi.org/10.1049/el:19920885. interesting to combine our approach with the method from , which I.N. Duling, Opt. Lett. 16 (1991) 539, https://doi.org/10.1364/ol.16.000539. J. Sotor, G. Sobon, J. Tarka, I. Pasternak, A. Krajewska, W. Strupinski, K. also achieves stable pulse sequences with an SSL of around 60 dB, to M. Abramski, Opt. Express 22 (2014) 5536, https://doi.org/10.1364/ further enhance the stability of the laser operation in the harmonic oe.22.005536. mode-locking regime. K. Yin, B. Zhang, L. Li, T. Jiang, X. Zhou, J. Hou, Photon. Res. 3 (2015) 72, https:// doi.org/10.1364/prj.3.000072. S.A. Filatova, V.A. Kamynin, I.V. Zhluktova, A.I. Trikshev, N.R. Arutyunyan, M. 4. Conclusions G. Rybin, E.D. Obraztsova, D.T. Batov, V.S. Voropaev, V.B. Tsvetkov, Quantum Electron. 49 (2019) 1108, https://doi.org/10.1070/qel17162. S. Kivisto, O.G. Okhotnikov, IEEE Photon Technol. Lett. 23 (2011) 477, https://doi. The feasibility of developing highly compact, robust, and stable org/10.1109/lpt.2011.2109945. sources of ultrashort pulses with repetition rates ranging from 100 to A. Lohner, P. Kruck, W.W. Rühle, Appl. Phys. B 59 (1994) 211, https://doi.org/ 300 MHz has been demonstrated. These sources are based on composite 10.1007/bf01081172. P.W. Smith, A.M. Weiner, IEEE Circuits Devices Mag. 4 (3) (1988) 3–7, https://doi. active fibers, WIT, and SWCNT. At a maximum pulse repetition rate of org/10.1109/101.942. 298 MHz at a central radiation wavelength of 1543 nm, the pulse E.O. Potma, D.J. Jones, J.-X. Cheng, X.S. Xie, J. Ye, Opt. Lett. 27 (2002) 1168, duration was 2.3 ps, and the output power and signal-to-noise ratio https://doi.org/10.1364/ol.27.001168. A. Martinez, S. Yamashita, Opt. Express 19 (2011) 6155, https://doi.org/10.1364/ reached 1.64 mW and 70 dB, respectively. oe.19.006155. A.I. Trikshev, V.A. Kamynin, V.B. Tsvetkov, P.A. Itrin, Quantum Electron. 48 CRediT authorship contribution statement (2018) 1109, https://doi.org/10.1070/qel16839. V.A. Ribenek, P.A. Itrin, D.A. Korobko, A.A. Fotiadi, APL Photonics 9 (5) (2024), https://doi.org/10.1063/5.0195623. Andrei Zverev: Writing – review & editing, Writing – original draft, Y.-S. Jang, J. Park, J. Jin, Sci. Rep. 13 (2023), https://doi.org/10.1038/s41598- Methodology, Investigation, Data curation, Conceptualization. Vladi 023-30532-z. R. Thapa, D. Nguyen, J. Zong, A. Chavez-Pirson, Opt. Lett. 39 (2014) 1418, https:// mir Kamynin: Writing – review & editing, Writing – original draft, doi.org/10.1364/OL.39.001418. Methodology, Investigation, Data curation, Conceptualization. Vladi C. Lerminaux, R.O. Maschmeyer, SPIE Proc. (1997), https://doi.org/10.1117/ mir Tsvetkov: Writing – review & editing, Writing – original draft, 12.264144. X. Wei, S. Xu, H. Huang, M. Peng, Z. Yang, Opt. Express 20 (2012) 24607, https:// Methodology, Investigation, Data curation, Conceptualization. Boris doi.org/10.1364/OE.20.024607. Denker: Writing – review & editing, Writing – original draft, Resources, J. Zheng, S. Yang, X. Nie, L. Li, J Russ Laser Res 42 (2021) 82, https://doi.org/ Conceptualization. Sergey Sverchkov: Writing – review & editing, 10.1007/s10946-020-09932-3. A.D. Zverev, V.A. Kamynin, S.A. Filatova, V.G. Voronin, V.B. Tsvetkov, B. Resources. Vladimir Vel’miskin: Writing – review & editing, Writing – I. Galagan, S.E. Sverchkov, B.I. Denker, V.V. Vel’miskin, Y.G. Gladush, E. original draft, Methodology, Investigation, Data curation, Conceptuali M. Khabushev, D.V. Krasnikov, A.G. Nasibulin, Optik 249 (2022) 168218, https:// zation. Yuri Gladush: Writing – review & editing, Writing – original doi.org/10.1016/j.ijleo.2021.168218. D. Galiakhmetova, Y. Gladush, A. Mkrtchyan, F.S. Fedorov, E.M. Khabushev, D. draft, Resources, Methodology, Conceptualization. Dmitry Krasnikov: V. Krasnikov, A.G. Nasibulin, Carbon 184 (2021) 941–948, https://doi.org/ Writing – review & editing, Resources. Albert Nasibulin: Writing – 10.1016/j.carbon.2021.08.032. review & editing, Supervision. G. Karlsson, F. Laurell, J. Tellefsen, B. Denker, B. Galagan, V. Osiko, S. Sverchkov, Appl. Phys. B 75 (2002) 41, https://doi.org/10.1007/s00340-002-0950-4. O.N. Egorova, S.L. Semjonov, V.V. Velmiskin, Y.P. Yatsenko, S.E. Sverchkov, B. Funding I. Galagan, B.I. Denker, E.M. Dianov, Opt. Express 22 (2014) 7632, https://doi.org/ 10.1364/oe.22.007632. A. Moisala, A.G. Nasibulin, D.P. Brown, H. Jiang, L. Khriachtchev, E.I. Kauppinen, This work was supported by the Ministry of Science and Higher Chem. Eng. Sci. 61 (2006) 4393, https://doi.org/10.1016/j.ces.2006.02.020. Education of the Russian Federation under Grant 075-15-2022-315, and E.M. Khabushev, D.V. Krasnikov, O.T. Zaremba, A.P. Tsapenko, A.E. Goldt, A. carried out on the basis of the World-Class Research Center Photonics. G. Nasibulin, J. Phys. Chem. Lett. 10 (21) (2019) 6962–6966, https://doi.org/ 10.1021/acs.jpclett.9b02777. A. Kaskela, A.G. Nasibulin, M.Y. Timmermans, B. Aitchison, A. Papadimitratos, Y. Tian, Z. Zhu, H. Jiang, D.P. Brown, A. Zakhidov, E.I. Kauppinen, Nano Lett. 10 Declaration of competing interest (2010) 4349, https://doi.org/10.1021/nl101680s. The authors declare that they have no known competing financial interests or personal relationships that could have appeared to influence the work reported in this paper. 4 A. Zverev et al. Optical Fiber Technology 88 (2024) 104007 S. Kobtsev, A. Ivanenko, Y.G. Gladush, B. Nyushkov, A. Kokhanovskiy, A. S. Kivistö, T. Hakulinen, A. Kaskela, B. Aitchison, D.P. Brown, A.G. Nasibulin, O. S. Anisimov, A.G. Nasibulin, Opt. Express 24 (2016) 28768, https://doi.org/ G. Okhotnikov, Opt. Expr. 17 (4) (2009) 2358–2363. 10.1364/oe.24.028768. M. Liu, Y. Qi, S. Yang, Z. Bai, B. Yan, J. Ding, Z. Lu, Appl. Phys. B 128 (10) (2022) 190, https://doi.org/10.1007/s00340-022-07913-9. 5