BHCS2003 Regulation of Gene Expression in Eukaryotes PDF
Document Details
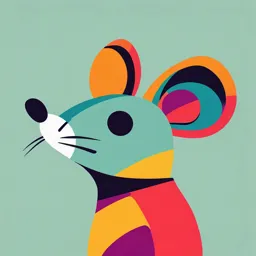
Uploaded by FortunatePlot
University of Plymouth
Tags
Related
- Topic 5 Regulation Gene Expression PDF
- BIOCHEMISTRY REGULATION OF GENE EXPRESSION & MUTATIONS PDF
- Module 3: Gene Expression And Regulation PDF
- L9 - Regulation of Gene Expression PDF
- Biology Chapter 18 Lecture Regulation of Gene Expression PDF
- Gene Expression Regulation in Eukaryotes (Part 2) Lecture Notes PDF
Summary
This document discusses the regulation of gene expression in eukaryotes. It covers various aspects of gene regulation including the structure of chromatin, DNA, and different levels of regulation. This document is a great resource for undergraduate biology students.
Full Transcript
BHCS2003 Genetic Continuity & Diversity Introduction Eukaryotes have thousands of genes...
BHCS2003 Genetic Continuity & Diversity Introduction Eukaryotes have thousands of genes Eukaryote gene expression can be very complex May be great variation in when a gene is being expressed Regulation of Gene Expression (transcribed) and when it is not in Eukaryotes Vast majority of genes in a eukaryote cell are switched off at any one time Eukaryote gene regulation must be able to: Turn off the expression of most genes in the genome Generate thousands of patterns of gene expression with a limited number of regulatory proteins 1 2 Eukaryote gene regulation Eukaryote gene regulation Greater amount of genetic information than in prokaryotes Transcripts of eukaryote genes are processed before and the DNA is complexed with histones and other proteins transport to the cytoplasm to form chromatin Eukaryote mRNA has a longer half-life than in prokaryotes The structure of chromatin is the major on/off switch for gene regulation. If open (decondensed) it is available to be Because mRNA is much more stable, eukaryotes have a transcribed; if closed (condensed) it is not series of translational controls DNA carried on many chromosomes (rather than just one) Most eukaryotes are multicellular with differentiated cell and these are enclosed within a nucleus bounded by a types. Different cell types typically use different genes to double membrane make different proteins, even though each cell contains a complete set of genes Transcription and translation are thus spatially and temporally separated allowing control mechanisms Regulation of eukaryote gene expression can thus (attenuation control) which are not possible in prokaryotes potentially occur at several levels 3 4 Gene Structure of haemoglobin regulation Regulation of gene expression can occur at several stages of the process of going from: DNA → RNA → protein Most eukaryote genes are regulated in part at the transcriptional level Four globin chains (different colours) each with an associated heme group 5 6 1 Chromosomal location of globin genes Haemoglobin synthesis Human haemoglobin is heterogeneous During development genes are differentially expressed producing a series of different globin chains 8 gene loci code for the 6 types of globin chains Genes are located on chromosomes 11 and 16 together Adult haemoglobin HbA (α2β2) ~97%, remainder HbA2 (α2δ2) with a number of pseudogenes (Ψ) Foetal haemoglobin HbF (α2γ2) 50-80% newborn haemoglobin Genes on both chromosomes expressed to produce the 2 Embryonic haemoglobin comprises Hb Portland (ζ2γ2) and Hb types of globin chains in the various forms of haemoglobin Gower I (ζ2ε2) synthesised in embryos 8 weeks 7 8 Eukaryote gene regulation Eukaryote gene regulation Functional architecture of the interphase nucleus Functional architecture of the interphase nucleus Chromatin remodeling Chromatin remodeling Assembly of the basal transcription complex Assembly of the basal transcription complex Control of chromatin structure and rate of transcription Control of chromatin structure and rate of transcription DNA methylation and regulation of gene expression DNA methylation and regulation of gene expression Post-transcriptional regulation of gene expression Post-transcriptional regulation of gene expression The role of non-coding RNAs The role of non-coding RNAs 9 10 Interphase nucleus Interphase nucleus During interphase, the chromosomes are unwound Chromosomes in discrete compartments called territories Chromosome painting (FISH) demonstrates this is not Channels between called interchromosomal domains random but highly organised Chromosome structures are continuously rearranged so Nuclear organisation of that transcriptionally active genes are cycled to the edge of chromosomes and dynamic chromosome territories at the edge of interchromosomal changes in chromatin structure domain channels are key elements in controlling gene expression As a result, pre-mRNA transcripts are likely to be formed on territory borders, to accumulate in the interchromosomal channels, and be made ready for transport to the cytoplasm Nucleus hybridised to probes for Before transcription can begin however, chromatin chromosomes 18 (aqua), X (green), and Y (red) remodeling is needed 11 12 2 Eukaryote gene regulation From DNA to chromosomes Functional architecture of the interphase nucleus Chromatin remodeling Assembly of the basal transcription complex Control of chromatin structure and rate of transcription DNA methylation and regulation of gene expression Post-transcriptional regulation of gene expression The role of non-coding RNAs Chromosome 17 as seen in a G-banded, 400 band preparation 13 14 Chromatin remodeling Chromatin remodeling DNA in chromosomes is combined with histone and non- Histone tails are exposed on surface of the nucleosome histone proteins to form chromatin and can be modified by acetylation, phosphorylation, and methylation to allow access to underlying DNA Some chromosome regions highly condensed and form transcriptionally inert heterochromatin Acetylation of lysine residues allows transcriptional enzymes access to the DNA template Other regions (euchromatin) have a more open structure and genes here can be transcribed Acetylation mediated by acetyltransferases and deacetylation by histone deacetylases Changes in chromatin organisation are required for DNA repair, replication, recombination as well as transcription Both important in the regulation of gene expression Structure of nucleosomes integral to this Following chromatin remodelling, recruitment of coactivators is required Many changes are mediated by chemical modifications of the N-terminal tails of core histones in a nucleosome These help assemble factors necessary for transcription 15 16 Nucleosome structure Eukaryote gene regulation Functional architecture of the interphase nucleus Nucleosome structure showing the protruding Chromatin remodeling histone tails Assembly of the basal transcription complex Sites of posttranslational modifications such as Control of chromatin structure and rate of transcription acetylation and methylation shown for one histone tail DNA methylation and regulation of gene expression All tails contain such sites Posttranscriptional regulation of gene expression The role of non-coding RNAs 17 18 3 Control of transcription Molecular organisation of promoters Eukaryote genes have two types of regulatory Promoters consist of nucleotide sequences that serve as sequences that control their transcription the recognition site for RNA polymerase binding Called promoters and enhancers Needed to initiate transcription and located a fixed distance from site where transcription is initiated Located immediately adjacent to genes they regulate and considered to be part of the genes Promoter regions usually several hundred nucleotides long The promoter at the 5’-end of eukaryotic genes consist of several elements including the TATA box, the CAAT box, and the GC box 19 20 Molecular organisation of promoters Molecular organisation of promoters Eukaryote promoters require the binding of a number of Promoter regions of many genes contain other elements proteins to initiate transcription CAAT box has the consensus sequence CAAT or CCAAT Promoter regions usually within ~100 bp upstream of gene Frequently located 70-80 bp from start site Promoter region of most genes has several elements TATA box is promoter to which RNA polymerase will bind Can function in a 5’-to-3’ or 3’-to-5’ orientation Located ~20 bases upstream of initial transcription point GC box has consensus sequence GGGCGG and often found at about position -110 Consists of an 8-bp consensus sequence (conserved in most genes studied) composed only of AT base pairs often Found in either orientation & often occurs in multiple copies flanked by GC-rich regions The CAAT and GC elements bind transcription factors and Mutations in TATA box can reduce transcription and function more like enhancers (see later) deletions may alter initiation point 21 22 Effect of point mutations in the promoter Molecular organisation of promoters region of β-globin gene Sequences that make up upstream regulatory elements vary in location and organisation. There are differences in the number, orientation, and location of promoter elements in different genes A majority of mammalian genes have more than one promoter SV40 control region Thymidine kinase Each line represents the transcription level produced by a single nucleotide mutation relative to wild type Dots represent nucleotides in which no mutation was obtained Insulin gene Note that mutations within the specific elements of the promoter have the greatest effect on the level of transcription 23 24 4 A gene with alternative promoters Alternative promoters - dystrophin gene Strachan & Read, 2019 Seven alternative promoters shown: C cortical, M muscle, P Purkinje cells, R retinal brain and cardiac muscle cells, CNS central nervous system, S Schwann cells, G general (widely expressed) 4 alternative promoters (P), first exons (E1) shown as light coloured boxes, plus two downstream exons (E2, E3) Each promoter uses own first exon (shown in red) together with shared downstream exons (numbered and shown in blue) Splice donor (D) and splice acceptor (A) sites shown Protein product from each named for molecular mass Splice junctions made by joining a D to an A site eg Dp427 is a 427 kDa dystrophin protein Thus, exon E1α is spliced onto exon E2 and not exon E1β Strachan & Read, 2019 25 26 Molecular organisation of promoters RNA polymerases and promoters Remember also that eukaryote genes use 3 classes of Unlike prokaryotes, eukaryote chromosomes are chromatin RNA polymerases for transcription with most promoters hidden within the nucleosomes Type I - ribosomal RNAs Chromatin structure has to be altered and opened up Type II - mRNAs and snRNAs Type III - tRNAs, 5S rRNA, several other RNAs Unlike prokaryotes, a set of transcription factors has to be preassembled on the promoter to serve as a platform for eukaryote RNA polymerase to bind Promoter for each polymerase has a different nucleotide sequence and binds different transcription factors 27 28 RNA polymerases and promoters Eukaryote gene regulation To initiate formation of the apparatus, TFIID complex binds Functional architecture of the interphase nucleus to the TATA box via its TBP (TATA binding protein) subunit Chromatin remodeling TFIID is the TBP plus a set of additional factors called TAFs (TATA Associated Factors) Assembly of the basal transcription complex TFIID responds to contact with activator proteins by conformational changes that allow binding of other factors Control of chromatin structure and rate of transcription such as TFIIA and TFIIB DNA methylation and regulation of gene expression RNA polymerase II and additional factors such as TFIIF then bind followed by TFIIE, THIIH, and TFIIJ Post-transcriptional regulation of gene expression The final stage is promoter clearance during which RNA The role of non-coding RNAs polymerase leaves the TATA box and transcription downstream ensues at a minimal basal rate 29 30 5 Enhancers control chromatin structure and Enhancers the rate of transcription Enhancers can be either side of a gene, at some distance from a gene, or even within the gene The induced state, in which transcription is stimulated above the basal level, is less well defined Cis regulators found adjacent to the structural gene that they regulate. (Trans regulators such as binding proteins Involves other areas of the promoter region, enhancers, can regulate a gene on any chromosome) and transcription factors which control assembly of the transcription complex and the rate of promoter clearance Typically interact with multiple regulatory proteins to increase efficiency of transcription or activate the promoter Within enhancers, binding sites often found for positive as well as negative gene regulators (More complex in structure and function compared to the operator regions of prokaryotes) 31 32 Enhancers Enhancers Enhancers distinguished from promoters by: Downstream enhancers found in the human β-globin gene and the chicken thymidine kinase gene 1. Position of enhancer is not fixed – can be upstream, downstream, or within gene it regulates In chickens, an enhancer located between the β-globin and the ε-globin genes works in one direction during embryonic 2. Orientation can be inverted without significantly development and the opposite direction to regulate β-globin affecting it’s action during adult life 3. If an enhancer is experimentally moved to another Upstream activator sequences known in yeasts location in the genome, or if an unrelated gene is Within gene enhancer regulates the immunoglobin heavy place near an enhancer, the transcription of the chain gene and is located in an intron adjacent gene is enhanced Enhancer is only active in tissues where immunoglobulin genes are being expressed which indicates tissue-specific gene expression can be modulated through enhancers 33 34 Enhancers Enhancers Enhancers are necessary for full level of transcription DNA forms a loop to bring together an enhancer and promoter elements Responsible for time- and tissue-specific gene expression Their interaction with RNA Stimulate transcription at a distance. How?? polymerase II initiates transcription First, transcription factors bind to enhancers and alter the configuration of chromatin Second, by bending or looping the DNA, they bring enhancers and promoters into direct contact in order to form complexes with transcription factors and polymerases Looping of DNA requires a special In the new configuration, transcription is stimulated to a class of DNA binding proteins known higher level increasing the overall rate of RNA synthesis as architectural proteins 35 36 6 Cooperative interactions Cooperative interactions In a complex organism, transcription levels have to be regulated over a wide range Levels finely adjusted by the clustering of cis-acting elements into enhancers Having several transcription factors (same or different) together often has a synergistic effect Presence of multiple bound sites can catalyse the formation Regulatory proteins bind to same face of DNA double helix of an enhanceosome, a large protein complex DNA bent by architectural proteins allowing regulatory Transcription activated to very high levels only when all the proteins to touch each other proteins are present and touching each other in the right way Transcription factors can then recruit a coactivator (CBP) which binds to them and to RNA polymerase II 37 38 True activators and antirepressors True activators This second class of transcription factors that bind to True activators are modular proteins with at least 2 domains enhancer sites can act as positive factors to increase transcription or as negative factors to decrease it One (DNA-binding domain) binds to DNA sequences in the enhancer Positive class can be divided into two types: Second (trans-activating domain) activates transcription through protein-protein interaction binding to RNA True activators function by contacting elements polymerase or other transcription factors at the promoter of the transcription complex at the promoter and activating transcription DNA-binding domains have distinctive 3-D structures or motifs and there a number of major types including: Antirepressors function by altering the chromatin structure to allow other factors to bind helix-turn-helix (HTH) zinc finger basic leucine zipper (bZIP) 39 40 True activators Antirepressor transcription factors A helix-turn-helix or homeodomain As described earlier, chromatin structure is the on/off switch in which (a) 3 planes of the α-helix for gene activity of the protein are established and (b) these domains bind in the Antirepressors remodel chromatin which is altered by two grooves of the DNA molecule different processes The first is catalysed by an ATP hydrolysis dependent remodeling complex of which SWI/SNF is an example These can physically shuffle nucleosomes along the DNA A zinc finger in which (a) cysteine Some complexes can swap variant histone molecules into and histidine residues bind to a Zn++ atom which then (b) loops or out of nucleosomes altering stability or function the amino acid chain out into a fingerlike configuration 41 42 7 Chromatin remodeling complex Chromatin remodeling Changing subunits can change patterns of gene expression Second mechanism is histone modification catalysed by one of the many histone acetyltransferase enzymes (HAT) Acetylation of histones lessens the attraction between basic histone protein and acidic DNA HATs are targeted to genes by specific transcription factors Remodeling of chromatin containing a gene entails interaction embryonic stem cells neural progenitor cells post-mitotic neurons between a remodeling complex and a HAT The BAF complex always contains the same number of subunits from What can be opened can be closed - deacetylases can remove the same sets of families, but different family members are found in the acetate from histone tails complex in different cell types Insulator elements are short DNA sequences that bind specific These differences result in different patterns of gene expression leading proteins and act as barriers to prevent the spread of remodeling to functional differences into nearby genes Strachan & Read, 2019 43 44 Eukaryote gene regulation DNA methylation Functional architecture of the interphase nucleus Alteration of chromatin conformation is one way in which eukaryote gene expression is regulated Chromatin remodeling Another mechanism is that of DNA methylation – adding or removing methyl groups from the DNA bases Assembly of the basal transcription complex Usually involves addition of methyl groups to cytosine Control of chromatin structure and rate of transcription About 5% of cytosine residues are methylated, but can be DNA methylation and regulation of gene expression tissue specific (varies between ~2% and 7%) Low amounts of methylation are associated with high levels Post-transcriptional regulation of gene expression of gene expression and vice-versa The role of non-coding RNAs In mammals, the inactivated X chromosome has a higher level of methylation than the active X 45 46 X inactivation Eukaryote gene regulation Calico cats are females heterozygous for the Functional architecture of the interphase nucleus alleles O (orange fur) and o (black fur) Inactivation of the O-bearing X chromosome produces a black patch; inactivation of the o- Chromatin remodeling bearing X chromosome results in orange patch White areas result from a separate gene Assembly of the basal transcription complex A mechanism for dosage compensation Control of chromatin structure and rate of transcription Inactivation occurs during an early stage of development and is random (either maternal or paternal X silenced) DNA methylation and regulation of gene expression Inactive X state is propagated to all progeny cells Post-transcriptional regulation of gene expression Such a heritable alteration, in which DNA sequence itself is unchanged, is called epigenetic inheritance The role of non-coding RNAs (In the germ line, the second X becomes reactivated) 47 48 8 Post-transcriptional regulation Alternative splicing pathways for mRNA Regulation of gene expression can occur at many points along Alternative splicing can generate different forms of a protein the pathway from DNA to protein so that expression of one gene can give rise to a family of related proteins Transcriptional control the most widely used Post-transcriptional regulation also occurs in many species Estimated >90% of human genes are alternatively spliced Nuclear RNA transcripts modified prior to translation, introns Alternative splicing can change the ligand recognised by a removed, exons spliced together, mRNA modified by addition receptor or an adhesion protein, the cellular localisation of a of a cap at the 5’ end and a poly-A tail at the 3’ end, message protein, its phosphorylation, or recognition of a ligand by an complexed with protein, and exported to the cytoplasm enzyme Each of these processes offers opportunities for regulation The number of different messages from one gene may exceed 1000 and the number of distinct proteins in humans Two important ones are (a) alternative splicing to give multiple is estimated to be between 100,000 and one million RNAs, and (b) regulation of mRNA stability 49 50 Alternative splicing pathways for mRNA Alternative splicing pathways for mRNA Example in the bovine preprotachykinin gene (PPT) Alternative splicing of the initial RNA transcript of the Produces 2 neuropeptides (P and K) which belong to preprotachykinin gene (PPT) a family of neurotransmitters called tachykinins Exons are either numbered or represented by letters P or K Have different physiological roles P largely restricted to nervous system tissues K is found mainly in the intestine and thyroid When the K exon is excluded, α-PPT mRNA is produced, and only the P neuropeptide is synthesised The inclusion of P and K exons leads to β-PPT mRNA, which after translation, yields both P and K tachykinin neuropeptides 51 52 Alternative splicing pathways for mRNA Regulation of mRNA stability With alternative splicing, how many different polypeptides After mRNA precursors are processed and transported, can be derived from the same pre-mRNA? they enter the pool of cytoplasmic mRNA molecules from which messages are recruited for translation α-tropomyosin helps regulates muscle contraction mRNA molecules have a characteristic half-life The α-tropomyosin gene in rats has 14 exons, 6 of which make up 3 pairs that are alternatively spliced Lifetimes vary widely: some are degraded within minutes, others last hours, months or years (mRNAs in oocytes) Only one of each pair ends up in the finished mRNA, resulting in 10 different forms of α-tropomyosin, many of mRNA stability is intrinsic to the sequence of mRNA which are expressed in a tissue-specific manner A mRNA molecule contains an open reading frame coding The muscle form of troponin T regulates the calcium for a protein, but also untranslated regulatory sequences needed for contraction Can include stability sequences, an address sequence (to This gene produces 64 known forms of the protein from a locate mRNA in a particular cellular compartment), and single pre-mRNA by alternative splicing instability elements (either general or cell-type specific) 53 54 9 Regulation of mRNA stability Eukaryote gene regulation Translation level control - uses the message to control its stability Functional architecture of the interphase nucleus Example in the synthesis of α- and β-tubulins, the subunit components Chromatin remodeling of microtubules Synthesis is stimulated at low cellular Assembly of the basal transcription complex concentrations and inhibited at high concentrations Control of chromatin structure and rate of transcription How does this autoregulation work ? First 4 amino acids are a recognition element to which regulatory factors DNA methylation and regulation of gene expression bind – the concentration of the subunits may serve this function Post-transcriptional regulation of gene expression The protein-protein interaction invokes an RNase which degrades the tubulin mRNA shutting down tubulin biosynthesis The role of non-coding RNAs Translation-coupled mRNA turnover also proposed as a regulatory mechanism for other genes – histones, lymphokines, cytokines 55 56 RNA genes GENCODE Unexpected finding in the post-genome era was the Class Number number and variety of non-coding RNAs (ncRNAs) Protein-coding genes 19411 At least 85% of the genome known to be transcribed! RNA genes ncRNAs used to be thought of as accessories needed to Making long ncRNA 20310 process genes to make proteins – tRNA, mRNA, and rRNA, but many other classes of ncRNAs Making small ncRNA 7565 Pseudogenes 14716 May undergo cleavage to become mature gene expression products and may be chemically modified Processed 10657 Unprocessed 3564 Single stranded RNAs much more flexible than ds DNA and can adopt different shapes according to base Other 258 sequence enabling them to do different jobs including roles in control of gene expression Version 46, May 2024 57 58 Noncoding RNA (ncRNA) MicroRNAs A class of gene regulation mediated by small, non-coding RNAs, including microRNAs MicroRNAs are thought to control expression at the post- transcription level by degrading or repressing target mRNAs MicroRNAs encoded by own set of genes and require a complex set of proteins for formation and functioning At least 1850 microRNA genes in the human genome Expressed at high levels in cells and dynamically regulated in response to physiological and environmental factors Two panels on left show ubiquitously expressed RNAs important in protein production and export. Each type of cell likely to have a specific microRNA Middle panel includes RNAs involved in DNA replication. Diverse classes of RNA regulate gene expression. Ubiquitous RNAs that have general roles, but many classes of RNA regulate specific environment defined by unique set of microRNAs genes and are restricted in expression. Others silence transposable elements in germ cells. 59 60 10 MicroRNAs Processing of microRNAs Each microRNA has the potential to regulate a large 1.Transcribed in the number of target genes, possibly >200, which implies >30% nucleus of protein-coding genes may be regulated by microRNAs 2.Cleaved into pre- miRNAs by Drosha Thus, the microRNA environment can modulate levels of enzyme complex and protein expression by dampening the translation of exported into the cytoplasm thousands of mRNAs 3.Internal loop cleaved Can also influence mRNA stability by Dicer complex and nonfunctional strand degraded MicroRNAs have important roles in regulation of apoptosis, proliferation, differentiation, development and metabolism 4.Mature miRNA (21-24 nt) complexed with Ago2 protein to All these effects may occur by regulating expression of form RNA-induced signaling molecules such as growth factors, cytokines, silencing complex transcription factors, and proapoptotic & antipoptotic genes (RISC) 61 62 Long noncoding RNAs Gene regulation by lncRNAs HOTAIR and Xist complex with Polycomb-group proteins to modulate heterochromatin methylation In addition to the primary transcript, most genes produce Represses transcription many unprocessed RNAs These may be derived from sequences near the promotor, within the introns, or at the 3’ end of the gene Transcribed in either the sense or antisense orientation LncRNAs (>200 nt) function to affect gene regulation by a variety of mechanisms More than 19,000 lncRNA genes are known 63 64 Gene regulation by lncRNAs Gene regulation by lncRNAs Tethering of a lncRNA to the promotor of a gene can Transcriptional activation can be achieved by recruitment of repress transcription in different ways transcription factors to the promotor by the lcnRNA May recruit a cofactor to the promotor where it prevents the binding of transcription factors May form a triple helix with promotor DNA preventing binding of transcription factors 65 66 11 And what about i-motifs ? References First visualised in living cells in 2018, now more than 50,000 Gibson (2015) A primer of human genetics, chapters 2 & 8 i-motif sites have been mapped in the human genome Griffiths et al (2020) Introduction to genetic analysis, 12th ed., chapter 12 I-motifs form when stretches of cytosine bases pair with each Hartl & Ruvolo (2019) Genetics: analysis of genes and genomes, 9th ed., other creating a four-stranded twisted structure protruding chapter 12 from the DNA double helix Klug et al (2020) Concepts of genetics, 12th ed., chapter 17, 18 &19 Not a random distribution Pierce (2020) Genetics: a conceptual approach, 7th ed., chapter 17 Concentrated in key functional areas of the Strachan & Lucassen (2023) Genetics and genomics in medicine, 2nd ed., genome chapters 2 & 6 Including regions that Strachan & Read (2019) Human molecular genetics, 5th ed., chapters 1 &10 control gene activity Martinez et al, 2024 67 68 References References Djebali et al (2012) Landscape of transcription in human cells. Nature, Amaral et al (2008) The eukaryotic genome as an RNA machine. 489, 101-108 Science, 319, 1787-1789 Kim et al (2008) Alternative splicing: current perspectives. BioEssays, 30, Baek et al (2008) The impact of microRNAs on protein output. Nature, 38-47 455, 64-71 Magistri et al (2012) Regulation of chromatin structure by long noncoding Bonasio et al (2010) Molecular signals of epigenetic states. Science, RNAs: focus on natural antisense transcripts. Trends in Genetics, 28, 389- 330, 612-616 396 Bonifer (2013) Why detailed model gene studies in higher eukaryotes are Martinez et al (2024) Human genomic DNA is widely interspersed with i- still necessary. Immunology, 139, 158-160 motif structures. The EMBO Journal, PREPRINT Chen & Riggs (2011) DNA methylation and demethylation in mammals. Memczak et al (2013) Circular RNAs are a large class of animal RNAs Journal of Biological Chemistry, 286, 18347-18353 with regulatory potency. Nature, 495, 333-338 Clamp M et al (2007) Distinguishing protein-coding and noncoding genes Morris & Mattick (2015) The rise of regulatory RNA. Nature Reviews in the human genome. PNAS USA, 104, 19428-19433 Genetics, 15, 423-437 69 70 References The Henry Stewart Talks Biomedical & Life Sciences Collection Olovnikov et al (2012) Small RNA in the nucleus: the RNA-chromatin ping-pong. Current Opinion in Genetics & Development, 22, 164-171 Go to Electronic Library → Find Databases → B → Pasquinelli (2012) MicroRNAs and their targets: recognition, regulation, Biomedical and Life Sciences Collection and an emerging reciprocal relationship. Nature Reviews Genetics, 13, 271-282 Perez-Ortin et al (2013) Eukaryotic mRNA decay: methodologies, 2,000+ lectures by leading world experts pathways, and links to other stages of gene expression. Journal of Available at https://hstalks.com/biosci/ Molecular Biology, 425, 3750-3775 Pink et al (2011) Pseudogenes: pseudo-functional or key regulators in health and disease. RNA, 17,792-798 Eisenberg, JC (2014) Heterochromatin, epigenetics and gene expression Ponting & Hardison (2011) What fraction of the human genome is Gibbons, R (2014) Chromatin genes and disease functional? Genome Research, 21, 1769-1776 Dean, A (2007) The beta-globin locus 71 72 12