Extraction and Separation of Rare-Earth Elements from Rock Phosphate Fertilizer PDF
Document Details
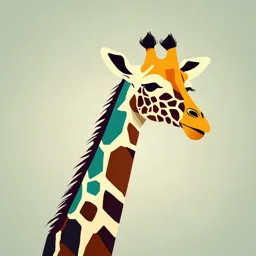
Uploaded by IrreproachableEpilogue
Wayne State University
2025
Chandra M. Tummala, Mohammed Dardona, Sai Praneeth, Ahmed K. Sakr, Dimitrios Kakaris Porter, Timothy M. Dittrich
Tags
Summary
This article details the extraction and separation of rare-earth elements from rock phosphate fertilizer. The study evaluated different methods to recover valuable elements, including precipitation and using a diglycolamide-associated sorbent. The research also considers the economic implications of the process.
Full Transcript
Separation and Puri cation Technology 357 (2025) 130139 Contents lists available at ScienceDirect Separation and Purification Technology...
Separation and Puri cation Technology 357 (2025) 130139 Contents lists available at ScienceDirect Separation and Purification Technology journal homepage: www.elsevier.com/locate/seppur Extraction and separation of rare-earth elements from rock phosphate fertilizer with a diglycolamide-associated organosilica sorbent Chandra M. Tummala , Mohammed Dardona , Sai Praneeth , Ahmed K. Sakr , Dimitrios Kakaris Porter , Timothy M. Dittrich * Department of Civil and Environmental Engineering, Wayne State University, 5050 Anthony Wayne Dr., Detroit, MI 48202, USA A R T I C L E I N F O A B S T R A C T Editor: B. Van der Bruggen Phosphate fertilizers are the main source for heavy metal and hazardous metal enrichment in agricultural soils, which can pose health risks to exposed organisms including humans. Recovery of valuable and potentially Keywords: hazardous metals such as rare-earth elements (REEs) from phosphate fertilizers before land application could Phosphate fertilizers limit the total metal loading of fertilizer-applied agricultural fields while providing an alternate supply of metals Rare-earth elements in the form of a value-added REE concentrate. Laboratory experiments evaluated the potential to extract and N,N,N′,N′-tetraoctyl diglycolamide (TODGA) separate REEs from three different types of rock phosphate fertilizer samples. Total REE concentrations measured Fluorapatites Solid-phase extraction with aqua regia microwave digestions ranged from 300 to 1,200 mg REE kg− 1 rock. More than 80% of the total Critical minerals leached REEs were from 5 elements (La, Ce, Nd, Pr, and Y). Extremely fast extraction kinetics were observed in Chelating ligands acid leaching experiments, with > 90% REE extraction within 15 min in 1.5 M HCl concentrations with a liq uid–solid ratio of 20 mL g− 1. Two methods of REE recovery from leachates were examined: (1) precipitation and (2) solid–liquid extraction with a new diglycolamide-associated sorbent media. A pH increase step (increasing the pH from ~ pH 0 to pH 2.5 with NaOH) precipitated more than 95 % of the REE from the leachate and produced a concentrate powder suitable for further enrichment. Proof-of-concept experiments using a new TODGA-associated organosilica media with filtered leachate or in a resin-in-pulp system demonstrated that REEs can be selectively separated from non-REE ions. Economic analysis of the metal oxide equivalent value (MOEV) identified metal values in phosphate fertilizer as high as $60 per ton. These findings may help reduce REE inputs from phosphate fertilizers to agriculture while also providing for recovery of critical elements that are crucial for many important technologies. 1. Introduction and an uncertain global REE supply, leading to alternative sources and methods for REE extractions gaining increasing importance. The rare-earth elements (REEs) are comprised of the fifteen lantha Alternative REE sources such as coal fly ash[22,5], heavy sands nide series elements plus scandium and yttrium and are important due to [15,32], acid mine drainage, e-waste, phosphogypsum, and their usage in numerous technological and industrial applications phosphate rocks have been previously investigated for potential REE including electric vehicles, phosphors, magnets, metal alloys, catalysts, extraction; however, these sources are associated with challenges such ceramics, and defense applications[30,40]). REEs are considered critical as low REE enrichment, high acid requirements (greater than 4 M to minerals crucial for national security and economic prosperity for effectively leach REEs), supply chain risks, and toxic waste disposal after countries with high-tech economies. As of 2022, greater than 96% REE recovery. of global REE raw production came from five countries: China (70%), Previous research has established that phosphate fertilizers can be the US (14.3%), Australia (6%), Myanmar (4%) and Thailand (2.4%) enriched with enough REEs to be considered a significant potential. REE sourcing is challenging due to their relatively low concen source. When the sulfuric acid wet process is used for phosphate trations in typical ores and high extraction costs. Most global REEs are fertilizer production, valuable and toxic metals in phosphate ores often sourced from bastnasite, monazite, and ion exchange clays. Geopolitical end up in wet-process byproducts like phosphogypsum, a calcium gyp issues and export restrictions have resulted in supply chain challenges sum hydrate. >90% of REEs are lost to the phosphogypsum (byproduct * Corresponding author. E-mail address: [email protected] (T.M. Dittrich). https://doi.org/10.1016/j.seppur.2024.130139 Received 2 August 2024; Received in revised form 14 October 2024; Accepted 15 October 2024 Available online 18 October 2024 1383-5866/© 2024 Elsevier B.V. All rights are reserved, including those for text and data mining, AI training, and similar technologies. C.M. Tummala et al. Separation and Puri cation Technology 357 (2025) 130139 in wet process) with the remainder transferred to phosphate fertilizers. Mineralogical changes before and after leaching were also evaluated to Continuous application of phosphate fertilizers could lead to enrichment help determine the leaching mechanism. REEs were then separated from in agricultural soils, bioaccumulation, and human exposure that may the phosphate leach liquors using three recovery methods including: (1) include health risks. REE recovery from phosphate fertilizers could precipitation, (2) N,N,N′,N′-tetraoctyl diglycolamide (TODGA) sorbent reduce the REE input into agricultural soils and serve as an alternative recovery from filtered leachate, and (3) TODGA sorbent recovery from means to recover REEs from an existing supply chain. There is insuffi unfiltered leachate (resin-in-pulp or RIP), in addition to using the cient basic science to inform the engineering of technology and systems TODGA media with dissolved precipitate. The proof-of-concept TODGA- that can efficiently recover REEs from commercial phosphatic fertilizers organosilica sorbent RIP application was tested where the fertilizer, and further advances are needed to consider phosphate fertilizers as a acid, and sorbent are added at the same time. The sorbent particles were potential alternative source for REE recovery. designed to be larger in diameter than the fertilizer grains, allowing for Phosphate fertilizers are an attractive untapped REE supply option subsequent separation of the sorbent grains. Because the TODGA ligand that could address several challenges. Approximately 21 million tons of exhibits high sorption capacity in concentrated acid solution chemistry phosphate rock was used for phosphate fertilizer production using the (>1 M), this sorbent and fertilizer feedstock are ideal candidates for a wet acid process in the US in 2022. Assuming 0.05% REE con resin-in-pulp system, which may significantly reduce energy consump centrations [38,7], 10,500 tons of REE were available for recovery from tion for rare-earth element recovery. There has been recent attention to US phosphate rock in 2022. This is equivalent to 95% (11,000 metric recovering REEs from phopshogypsum wastes, but little focus has been tons of REE compounds were imported) of US REE imports in the same placed on developing alternative systems using nitric and hydrochloric year (USGS, 2023). acid systems that would eliminate the production of phosphogypsum. Organic phosphate fertilizers, an alternative to conventional phos Three main novel ideas explored in this study are: (1) investigating the phate fertilizers, are phosphate minerals crushed to granules that are use of nitric, hydrochloric, and organic acids, (2) using TODGA media to then directly applied to the land and marketed as slow-release fertilizers. recover REEs from acid system without the need for initial pH increase, Direct land application of the crushed phosphate rock introduces REEs and (3) using a ternary resin-in-pulp system to eliminate the challenging and other metals as potentially soluble nutrients, toxins, or background initial filtration step. ions, depending on the element and subsequent weathering. High REE concentrations (>1,200 mg kg− 1) have been found in phosphate fertil 2. Material and methods izers in Europe and South America[21,28]. REE recovery from phos phate fertilizers could potentially reduce the REE input into agricultural 2.1. Feedstocks soils and could serve as an alternative pathway to recover REEs. Mehlich-3 extraction is a standard method to determine plant available Three commercially available organic phosphate fertilizers were elements (such as phosphorus) and can also be used to measure the purchased from suppliers in the United States and included: soft rock associated plant and bioavailable REEs and other metals (Kleinman & phosphate mined from Montana (MT), phosphatic clay (also called Sharpley, 2002). Although phosphate minerals are known to be associ colloidal phosphate) mined from Florida (FL), and sedimentary phos ated with REEs, further research is necessary to justify use as a potential phate rock from Utah (UT). All samples were manually crushed using a alternative source for REE recovery. Based on review of the scientific hand mortar and pestle and sieved to a particle size of ≤ 125 µm before literature, there is little prior research evaluating REE concentration and use in experiments (standard 120 mesh stainless steel sieve; process development to recover REEs from organic phosphate fertilizers. Fieldmaster®). Although leaching with sulfuric acid is effective in phosphoric acid Two reference samples were included for comparison (gypsum fer production, the loss of REEs in the precipitated phosphogypsum and tilizer and copper stamp sands, a legacy crushed copper ore waste environmental disposal issues provide a need for alternative systems. collected from Gay, MI, USA). The samples were sieved and the Mineral acids like hydrochloric acid (HCl) have proven economical and 0.125–0.25 mm size fraction was used for analysis. A flowchart showing effective in leaching REEs from phosphate minerals (Walawalkar et al., the experimental conditions for the following characterization, leach 2016); however, there is a need to develop optimized REE leaching ing, and separation system is shown in Figure S1. processes to efficiently extract REEs. Weak organic acids are a possible alternative, but may result in lower REE extraction efficiencies due to 2.2. Characterization experiments incomplete dissolution or the formation of insoluble polymers when reacted with phosphate minerals. Surface morphology and particle size of the phosphate fertilizers After the leaching process, the next important step is separation of were studied using scanning electron microscopy (JEOL-SEM, JSM- REEs from the leachate. Direct precipitation using hydroxides can be 7600F) with sample grains mounted on carbon tape and sputter used to separate REEs from the concentrated leachate liquors. These coated with gold to reduce possible charging effects. Elemental analysis techniques; however, have drawbacks including poor REE selectivity was conducted using an SEM-EDS setup (EDAX Pegasus, Apex 2). Oxide and limitations in extreme acidic conditions (pH less than 1.5). As the composition was determined by X-ray fluorescence spectrometry (Shi pH is increased above 2, other commonly present elements in the madzu, EDX-7000). Mineral composition was determined using X-ray phosphate feedstocks (like Ca, Fe, and Al) are also precipitated. Thus, diffraction analysis (Bruker, D2 phaser, Billerica, Massachusetts, United the precipitate formed in this step requires further processing to selec States). XRD data patterns were interpreted with correlation software tively extract REEs from other elements. These precipitates can subse (Match!, version 3.2.2, Crystal Impact, Bonn, Germany) and compared quently be dissolved in acidic solutions and REEs can be selectively with the crystal patterns in the International Centre for Diffraction Data recovered using extraction techniques with various ligands via solvent (ICDD) database. extraction or solid–liquid extraction[41,46,37,27,12,11,35]. These techniques include challenges such as poor selectivity for REEs and 2.3. Microwave digestion experiments (total extractable cations) limitations in extreme acidic conditions (pH < 1). The overarching aim of this study is to develop an efficient process to Total potentially extractable metal concentrations were determined extract REEs from phosphate mineral sources and quantify the govern by measuring ions mobilized from rock samples using microwave di ing mechanisms. Characterization and total digestion experiments were gestions with both aqua regia and concentrated nitric acid (Optima performed to determine the mineral and elemental composition of three grade, 67–70 w/w%, ~15.6 M) according to EPA method 3051A for types of organic phosphate fertilizers. Acid leaching experiments were microwave-assisted digestion of crushed crystalline rock material. conducted to measure acid compatibility and leaching kinetics. Crushed fertilizer (0.5 g) was added to microwave digestion tubes (CEM, 2 C.M. Tummala et al. Separation and Puri cation Technology 357 (2025) 130139 MARSXpress, modified polytetrafluoroethylene-TFM, 55 mL) with a Ho, Eu, Yb, Er, Dy, Sm, Gd, Pr, Ce, Nd, La and Y. The concentration of the nonreactive liner (CEM, MARSXpress disposable Teflon 55 mL liners). acid (6 M), solid–liquid ratio (1:20), temperature (microwave and Aqua regia was prepared by combining one part nitric acid and three ambient room), and REE release time (1 min to 24 h) are important parts hydrochloric acid (by volume) using 16 M nitric and 12.1 M hy variables that affect the metal ion leaching efficiencies. Of particular drochloric acid (Supelco, ACS grade). Aqua regia or nitric acid (10 mL) interest are the leaching behavior of REE and critical mineral (CM) el was added to the fertilizer in each liner and vials were tightly capped ements. We analyzed cation concentrations for 29 out of 50 CMs, which and placed in the microwave digestor (CEM, Mars 5, MARSXpress). The includes REEs (see complete list in Table S1) in addition to Sb, Be, Bi, Cs, temperature was ramped up from room temperature to 170 ◦ C in the F, Ge, C, Hf, In, Ir, Li, Nb, Pd, Pt, Rh, Rb, Ru, Ta, Te, Sn, Ti, W and Zr first two minutes and then held constant at 170 ◦ C for 10 min. Samples elements not measured due to either low concentration or difficulties in were cooled overnight, transferred to centrifuge tubes (50 mL poly ICP-MS analysis. A list of potentially toxic metal ions was also organized propylene, Corning®), and centrifuged (IEC international centrifuge, HT from these data (Ni, Cu, Zn, Cd, Pb, As, and Co) based on the Association centrifuge) at 5,031 relative centrifugal force (RCF) for 15 min. The of American Plant Food Control Officials (AAPFCO) and the California supernatant was filtered using 0.22 µm syringe filters (25 mm poly Department of Food and Agriculture (CDFA) limits. Batch leaching ex propylene, Choice) and prepared for inductively-coupled plasma mass periments were conducted to also provide data to identify optimal acid spectrometry (ICP-MS) analysis in 2% nitric acid (Fisher Chemical, leaching parameters to enable high REE leaching efficiencies and Optima grade). Cation concentrations were measured via ICP-MS (Agi transfer toxic and valuable ions to solution. lent Technologies, 7850). All experiments were conducted in duplicate Results from the initial 35 element leach screening with 6 M acids and included sample blanks (digestion protocol without rock materials) were used to determine the optimal recovery conditions to extract REEs, to account for potential measurement errors and possible contamina CMs, and toxic metals from phosphate fertilizers and led to the decision tion. Digestion methods have been previously verified with National to use HCl leaching for further extraction analysis. Institute of Standards (NIST) coal fly ash certified reference material REE leaching efficiencies were calculated based on equation 1: (CRM) 1633c. Leachingefficiency(%) = CL C−D 1 × 100 (1) 2.4. Rock phosphate fertilizer leaching experiments where CL is the concentration of the element in the leached solution, and CD is the concentration of the element in the aqua regia microwave Microwave digestion results were considered the maximum leach digested solution. able concentration; however, high energy consumption makes micro wave digestion unfavorable for scale up. To address this limitation, 2.5. Proof-of-concept REE recovery leaching experiments were also conducted with a range of extractants at room temperature to determine the overall leachability of over 35 ele Three recovery methods were tested to recover REEs from phosphate ments from the phosphate rocks and other feedstock materials without leachate liquors: (1) precipitation, (2) TODGA sorbent recovery from added heat. Three fertilizer sample types were leached with three filtered leachate, (3) TODGA sorbent recovery from unfiltered leachate mineral acids (6 M HCl, 6 M HNO3, and 6 M H2SO4), three organic acids (resin-in-pulp or RIP), in addition to using the TODGA media with dis (6 M acetic acid, 6 M formic acid, and 6 M propionic acid), Mehlich-3 solved precipitate. extractant solutions (0.2 M glacial acetic acid, 0.25 M ammonium ni trate, 0.015 M ammonium fluoride, 0.013 M nitric acid, 0.001 EDTA, pH 2.5.1. TODGA-organosilica sorbent synthesis and characterization 2.5), and high-purity water. A solid-to-liquid ratio of 1:20 g mL− 1 (1 g of The synthesis and characterization of a new N,N,N′,N′-tetraoctyl fertilizer in 20 mL acid) was used and an aliquot of sample was prepared diglycolamide (TODGA)-organosilica sorbent media has recently been for ICP-MS analysis after 24 h. The 1:20 ratio was selected to maximize reported to have high capacity for REEs in coal fly ash leachate and was REE extraction percentage, as the smaller ratio could lower the REE tested for REE recovery from the phosphate leach solutions reported yield. Mehlich-3 is a standard extraction solution used to determine above[25,26]. The chemical formula of organosilica-TODGA is shown in plant available nutrients from soil samples. This method offers the Figure S2. TODGA (C36H72N2O3, 99% purity, Technocomm Limited, UK) ability to differentiate between soluble and insoluble and unavailable was dissolved in methanol (Fisher Chemical, OPTIMA®), contacted with phosphate ions tightly incorporated in the mineral matrix that would not organosilica (60–80 mesh, ABS materials, USA) for 20 min, and then affect nutrient availability, mobile toxic metals, or recoverable ions of methanol was removed via vacufuge to produce 20 wt% TODGA- value in soil pore water conditions, which are mildly acidic from car organosilica. 2.1 g of sorbent were prepared for testing with a mass bonic and organic acids (Mehlich, 1984). Batch extraction experiments balance error of < 0.5% and TODGA-impregnated organosilica was were conducted to determine plant available metals present in the fer characterized before use. tilizers with Mehlich-3 solution and mineral samples using a liquid–solid ratio of 20:1. 2.5.2. REE recovery via precipitation/redissolution and TODGA- Based on the results from the 35 element acid leaching experiments organosilica sorbent with different 6 M acids, HCl was selected for further investigation to Sodium hydroxide was added to phosphate fertilizer leachate to in produce samples for the REE recovery experiments. REE leaching ex crease the pH and precipitate REEs as hydroxide complexes. Precipitates periments were conducted by adding 10 mL of acid (0.25–6 M for HCl) were filtered and dried at 50 ◦ C for 48 h, then added to HCl (6 ml of 4.0 to 0.5 g phosphate fertilizers in centrifuge tubes (polypropylene, 15 mL). M HCl solution; 1:20 solid to liquid, 15 mL centrifuge tubes). Tubes were Samples were placed on a rotator (Fisher Scientific™) and rotated (30 agitated for 15 s (vortex stirrer; 3000 rpm) and rotated for 24 h at 30 rpm, 137.5◦ inclination) for 24 h. The samples were prepared for ICP-MS rpm. The same procedure was applied to all three fertilizer leachate analysis using the same procedure as the digestion experiments precipitates. described above (filter after 24 h and dilute in 2% nitric acid). For the For batch sorption experiments, TODGA adsorbent media (50 mg) release experiments, sample aliquots were collected at regular intervals was added to the dissolved precipitate solution (5 mL) in the centrifuge ranging from 0, 10, 20, 40, 60, 120, 240, 480, 720, and 1440 min and tubes (15 mL, polypropylene) and rotated (30 rpm, 24 h). Duplicate were prepared for ICP-MS analysis. The final pH of the solution was samples were filtered after 24 h with 0.2 µm syringe filters (Basix, PTFE) measured for all leaching experiments at 24 h (after filtration) using a and diluted to 1:1000 using 2 % HNO3 in 15 ml centrifuge tubes for ICP- pH meter (Fisher Scientific, Accumet®, AE 150). All the experiments MS measurements (Agilent, 7850). were conducted in duplicate. Elements analyzed included Ca, Al, Fe, Mg, Sr, Zn, Cr, Ba, Mn, V, U, Cu, Ni, Cd, Ga, As, Pb, Th, Ag, Co, Lu, Tm, Tb, Sc, 3 C.M. Tummala et al. Separation and Puri cation Technology 357 (2025) 130139 2.5.3. REE recovery from filtered leachate with TODGA-organosilica 2.6. Analytical tools (ICP-MS, pH meter, and electrode) sorbent Based on results of the 0.25 M− 6 M HCl leaching experiments, Inductively-coupled plasma mass spectrometry (ICP-MS) was used to adsorption experiments were conducted with filtered 1.5 M HCl Utah measure the concentration of 46 cations in leachate solutions. ICP-MS fertilizer leachate. Fertilizer solid residue was filtered from the leachate samples were filtered (0.22 µm syringe filter, 25 mm polypropylene, with 0.22 µm syringe filters (Choice, polypropylene) and the leachate Choice) and diluted to 1:1000 with 2 % nitric acid (Optima grade, Fisher was used to conduct batch adsorption experiments, where TODGA- chemicals) in 15 mL centrifuge tubes within 30 min of collection to organosilica media (50 mg) was added to filtered leachate (5 mL), prevent solution instability from affecting measured concentrations. filtered after 24 h, and measured via ICP-MS. All experiments were run All cation concentrations were measured via ICP-MS (Agilent, 7850) in duplicate. using a previously reported ICP-MS method. An eight-point calibra tion was made for the concentration range between 0 to 200 ppb (1, 5, 2.5.4. REE recovery from ternary resin-in-pulp system (fertilizer, acid, 10, 25, 50, 100, 150, 200) for 46 elements (Ag, Al, As, B, Ba, Be, Ca, Cd, TODGA sorbent) Ce, Co, Cr, Cs, Cu, Dy, Er, Eu, Fe, Ga, Gd, Ho, K, La, Lu, Mg, Mn, Na, Nd, For the resin-in-pulp method, leaching with strong acid and selective Ni, P, Pb, Pr, Rb, S, Sc, Se, Sm, Sr, Tb, Th, Tl, Tm, U, V, Y, Yb, Zn) using sorption of REEs was conducted simultaneously for 24 h (fertilizer, acid, ICP-MS standard solutions (Inorganic Ventures, ICPMS-71A, High-Pu TODGA sorbent in ternary system). Fertilizer (1 g) and TODGA media rity Standards, 10 M48-2, Inorganic Ventures, MSY-10PPM, and High- (0.2 g) were added to mineral acid (20 mL; 6 M HCl, 6 M HNO3, and 6 M Purity Standards, 100–57–1–1250). 200 ppb indium was used as an H2SO4) in centrifuge tubes (50 mL, polypropylene) and rotated (30 rpm internal standard. for 24 h). The suspension was filtered with syringe filters (0.2 µm, Basix, PTFE) and analyzed for cations via ICP-MS. 2.7. Metal oxide equivalent value (MOEV) calculations The extractable metal oxide equivalent value (MOEV) was calculated by using the mass of extracted REEs, CMs, and other valuable metals and Fig. 1. SEM and EDS characterization of phosphate fertilizers for: a, d) Montana fertilizer samples; b, e) Florida fertilizer samples; c, f) Utah fertilizer samples. Carbon peaks were likely due to the carbon tape used to attach grains to the sample holder, while the gold peaks were due to the sputter-coated gold used to reduce charging effect during SEM-EDS analysis. 4 C.M. Tummala et al. Separation and Puri cation Technology 357 (2025) 130139 current spot prices of the equivalent oxide values (in the form of in phosphate fertilizers are generally correlated with the presence of individually-separated high purity (ISHP) oxide powders). Spot prices fluorapatite minerals, which are enriched with REE minerals during from the Shanghai Metal Market (SMM, February 2, 2024) were used for their formation. Utah fertilizer has approximately 4 times higher value calculations (Table S1). REE content than average coal fly ashand 136 times higher than the average value in beach sands. 3. Results and discussions The maximum leachable metal concentration numbers were then compared to the room temperature extraction results for 6 M concen 3.1. Phosphate fertilizer characterization trations of mineral acids, organic acids, Mehlich-3 extraction solution, and water to determine the efficacy of each acid type and to select one The elemental and mineral composition of commercial phosphate acid system for further system development. The results are shown in fertilizer depends on the parent geology of the phosphate rock ore source Fig. 3 and illustrate several important key points: (1) microwave aqua and can vary widely between locations. The three phosphate fertilizers regia and concentrated (12 M) HCl yield similar recovery amounts, (2) 6 used in this study were manufactured from phosphate rock mined at M HCl and 6 M HNO3 at ambient room temperature extracted >80% of different locations in the United States (FL, UT, and MT) and from the extractable TREE, (3) 6 M sulfuric acid leached < 20% of extractable different mineral types (colloidal clay, soft rock, and hard rock, TREE, and (4) 6 M organic acids (formic, acetic, and propionic), respectively). Surface morphology, particle size, elemental composition, Mehlich-3, and water leached < 1% of extractable metals. and mineral composition measured by SEM, SEM-EDS, and XRF are The concentration of light REE (LREE − La to Eu plus Sc) was found shown in Fig. 1. to be higher than heavy REE (Gd to Lu plus Y) in these fertilizers, and SEM images confirm particle diameter of the crushed material was more than 80% of the TREE present in the phosphate fertilizer were from below 152 µm with irregular, sharp-angled grains for the Montana and La, Ce, Nd, Pr, and Y (Fig. 4a). Mineral acids (nitric, hydrochloric, and Florida samples, while a portion of the particles in Utah are round. High- sulfuric) were found to leach REEs more effectively than the organic intensity peaks of calcium, phosphorus, aluminum, and silicon were acids tested. 6 M HCl and 6 M HNO3 leached more than 85% of the TREE observed in the SEM-EDS analysis (Fig. 1). This is consistent with present in all three phosphate fertilizers in ambient room temperature phosphate rock-derived fertilizers associated with fluorapatite minerals conditions without microwave treatment (Fig. 3). H2SO4 only leached. Low-intensity sulfur, magnesium, and fluorine peaks were also 10% of the TREE despite being a strong mineral acid, likely due to present in the Utah fertilizer, but were not observed in the other two coprecipitation with the phosphogypsum formed during the leaching samples. XRD analysis shows fluorapatite (>60% mineral composition) process. REEs can become structurally integrated into the phospho and SiO2 (between 7 to 30%) were present in all three fertilizers. gypsum by substituting three Ca2+ ions with two REE3+ ions (Dutrizac Additionally, the Montana sample is 31% calcium sulfate dihydrate and 2017). For organic acids, 6 M formic acid leached less than 5% of the the Utah sample is 9% calcite (Fig. 2). REEs in all three fertilizers and 6 M acetic acid and propionic acid leached less than 1% of the TREE present. Individual REEs extracted 3.2. REE leaching evaluation with aqua regia, mineral acids, and organic with the mineral and organic acids in the Utah fertilizer are shown in acids Fig. 4b. Although total REE contents of the three fertilizers vary widely, the Microwave digestion with aqua regia and concentrated hydrochloric relative enrichment values above elemental crustal abundance demon acid (12 M) were used to determine the maximum possible extractable strates different enrichments among the top 5 elements. Yttrium is the REE concentration from three fertilizer samples and two reference ma highest concentration REE in all three samples (>350 mg/kg in Utah terials (3 rock phosphate fertilizers, stamp sand, and gypsum fertilizer). sample). In the Montana and Utah samples, La, Nd, and Ce are the 2nd, Utah fertilizer had the highest TREE concentration with 1,027 ± 12.8 3rd, and 4th most abundant REEs with almost three times more La than mg kg− 1, followed by Montana fertilizer with 522.3 ± 47.2 mg kg− 1, Ce. However, in the Florida sample, Ce, La, and Nd are the 2nd, 3rd, and Florida fertilizer with 310.0 ± 1.2 mg kg− 1, stamp sand with 103.5 mg 4th most abundant REEs with a very similar concentration of all three kg− 1, and gypsum fertilizer with only 2.2 mg kg− 1 (Figure S3). The REE elements. The 5th most abundant element in all samples is Pr, followed concentration variation of the feedstocks can be attributed to the min by Gd, Dy, and Sm. eral composition of the source material. High TREE concentrations 3.3. Total leachable metals with 6 M HCl (46 elements – Major cations, TREEs and CMs) Aqua regia and HCl leaching experiments were used to screen sam ples for maximum leachable metal fractions and HCl leach solutions were further investigated for two reasons: (1) HCl leaching results from screening tests were similar to aqua regia; (2) HCl is more economical and compatible with downstream metal recovery from the leachate solutions. The measured concentrations of 46 elements for 6 M HCl leaching of the three phosphate fertilizers are shown in Fig. 5. Although copper, lead, cadmium and radioelements thorium and uranium are not listed in the critical elements category by USGS, their applications in critical sectors such as electric vehicles, EV batteries, and alloy production make them valuable and potential critical elements in the future (Bauer et al., 2023; U.S. Geological Survey 2023). Concentrations of cations can be divided into 3 groups based on concentration: (1) > 1,000 mg kg− 1, (2) 100–1000 mg kg− 1, and (3) < 100 mg kg− 1. Ca, Al, Fe, and Mg are each > 1,000 mg kg− 1 in the Utah fertilizer. Calcium alone is 63.4% of the total cation concentration and Ca, Al, Fe, and Mg together represent 92.1% of the total leached Fig. 2. Mineral composition of the rock fertilizers obtained from XRD analysis. elemental cation concentration. REEs represent 2.9% of the remaining 5 C.M. Tummala et al. Separation and Puri cation Technology 357 (2025) 130139 Fig. 3. Comparison of REE extraction with ten mineral and organic acids. Note that the first two samples (aqua regia and concentrated HCl) are for microwave digestion and the other eight are for room temperature leaching. 7.9% of the total measured cations (or 37% of the non– Ca, Al, Fe, and exceeded the CDFA arsenic limits (50 mg kg− 1 versus 14 mg kg− 1 limit, Mg cations). Elements in the 100–1,000 mg kg− 1 group include Sr, Zn, or 3.8 × higher) and was on the borderline for non-nutritive heavy metal Cr, Nd, La, and Y. Elements in the < 100 mg kg− 1 group include Ba, Mn, standard Cd limits proposed by the CDFA in the Montana fertilizer. V, U, Cu, Ni, Cd, Ga, As, Pb, Th, Ag, Co, Lu, Tm, Tb, Sc, Ho, Eu, Yb, Er, Even with these exceedances, the concentration of Cd and As present Dy, Sm, Gd, Pr, and Ce. in these phosphate fertilizers is not expected to significantly elevate the heavy metal concentrations in the receiving agricultural soils. For 3.4. Mixed oxide equivalent value (MOEV) – Critical and valuable example, a phosphate fertilizer containing 20 mg kg− 1 of Cd when elements applied at 500 kg ha− 1 can increase soil Cd concentrations by only 0.0033 mg kg− 1 per year. However, these heavy metals can bio The critical mineral metal oxide equivalent value (MOEV) for the accumulate in plants, affect soil productivity, and damage human health three phosphate samples were $63.6 ton-1 (Montana), $61.4 ton-1. Application of these fertilizers in agriculture fields without (Utah), and $40 ton-1 (Florida), where ton refers to metric ton (Fig. 6). recovering the high REE concentrations could potentially lead to the The REE value was the following: Utah ($45) > Montana ($30) > Florida enrichment of REEs in agricultural soils and crop tissue. It is ($24). The UT fertilizer sample had the largest value with > 90% of the important to note that these concentrations are the maximum leachable value attributed to eleven elements − 9 REEs, Ga, and V (Fig. 6 and REEs, and that other methods described below can help determine Figure S4). 36 of the 46 measured elements were included in MOEV whether the metals present would leach over time, or are not expected to calculations (with 29 of the 36 elements classified as critical elements or leach for 100s or 1000s of years. having potential supply chain risks). The plant available concentrations of heavy metals in phosphate Critical elements vanadium, chromium, zinc, barium and manganese with Mehlich-3 extractions varied between 0 to 35 mg kg− 1 (Fig. 7), with were between a concentration range of 100–600 mg kg− 1 in all three most plant-available metal concentrations less than 5 mg kg− 1. In the fertilizers and the USGS reports that the US imports more than 50% of Montana fertilizer, 34% of the Cd and 10% of the As are plant available, these elements for domestic use (USGS, 2024). which exceeds the CDFA standards. 70% of the total Cd concentrations in the Utah fertilizer are also plant available, while only 1% TREE in the 3.5. Heavy, toxic, and trace metal removal from phosphate fertilizers Utah fertilizer and 3% of the TREE in the Florida fertilizer are plant available. Although the plant available concentrations are low, this is an Heavy, toxic, and trace metal concentrations (considered environ important factor to consider because heavy metals can be absorbed by mental pollutants when present in excess levels) such as V, Fe, As, Co, Ni, the plants and can result in food toxicity. Extraction and recovery of the Cu, Zn, Sr, Cd, Ba, and Pb were measured in all three fertilizers. Heavy metals and TREE could potentially reduce the concentration of the metal concentrations were compared to standard fertilizer limits pro heavy metals and TREE that are soluble and plant available. posed by The Association of American Plant Food Control Officials (AAPFCO) and the California Department of Food and Agriculture 3.6. Leaching process efficiency optimization screening (CDFA) (Table S2 and Table S3). Although all three fertilizers were below acceptable AAPFCO heavy metal levels, the Montana fertilizer Based on the leaching results shown above, HCl was selected as the 6 C.M. Tummala et al. Separation and Puri cation Technology 357 (2025) 130139 lower solid–liquid ratios (or higher liquid–solid ratios) yield high REE leaching efficiencies (Fig. 8a). The leached REE concentrations increased until a solid–liquid ratio of 1:10, and a further decrease in the solid–liquid ratio gave no improvement in REE extraction yield. This suggests that there is a sufficient volume of acid to: (1) release ions into solution and (2) prevent precipitation due to solubility exceedances at a solid–liquid ratio of 1:10. Solid-liquid ratios above 1:10 increases the slurry density, which can slow down the reaction and lead to high concentration elements like Ca, Al, Fe, and P exceeding the solubility limit of the solvent solution and inhibiting further leaching. The optimum solid–liquid ratio for leaching REE from the phosphate fertil izers is between 1:10 and 1:20, where most of the REEs were extracted from the phosphate fertilizers. A conservative solid–liquid ratio of 1:20 was selected for subsequent experiments to eliminate potential solubil ity artifacts; however, lower solid–liquid ratios are effective as described. 3.6.2. Acid concentration (0–6 M HCl) To determine the optimum HCl concentration range, batch leaching experiments were conducted with a liquid–solid ratio of 20 mL g− 1, ambient room temperature (25 ◦ C), and by varying the HCl concentra tion from 0 to 6 M HCl. TREE leaching efficiencies of Montana, Florida, and Utah fertilizers surged from 34%, 31%, and 5% to 65%, 66%, and 84% between 0.5 M and 1.5 M HCl, respectively (Fig. 8b). An increase in the leach solution concentration (above 1.5 M) did not increase REE Fig. 4. REE leaching results from: (a) all three fertilizers in aqua regia and (b) Utah fertilizer using various acids. acid system for the proposed separation system and further experiments were conducted to determine optimal leaching conditions including solid–liquid ratio, HCl concentration, leaching time, and leaching temperature. 3.6.1. Solid-liquid ratio Leaching experiments were first conducted with 3 M HCl to identify a reasonable solid–liquid ratio range to then be used to re-evaluate acid concentration and leaching time and temperature. Results show that Fig. 6. Equivalent metal oxide value in phosphate fertilizer and coal fly ash. Fig. 5. 37 quantifiable elements present in the phosphate fertilizers (individual REEs 1–300 mg kg− 1). 7 C.M. Tummala et al. Separation and Puri cation Technology 357 (2025) 130139 reaction. At the initial stage of the dissolution process, acid reacts with the Ca, P, and Al ions, followed by the REE which are trapped in the inner surfaces. The optimum HCl concentration range to leach REEs from the phosphate fertilizers was found to be between 1.5 and 3 M. Another important consideration is whether any preferential extraction with different acid types and concentrations occurs that could provide additional separation benefits with a sequential extraction approach. Individual REE concentrations leached with 0.25 to 6 M HCl (Figure S6 and S7) show no significant difference in the leachability of individual REEs, suggesting that the mineral phases dissolve homoge neously and no preferential extraction is observed. Based on these ex periments, 1.5 M HCl concentration was selected for further investigation with the REE recovery system. 3.6.3. Temperature (25–65 ◦ C) Leaching experiments were performed at 25, 35, 50 and 65 ◦ C with 1.5 M HCl and a liquid–solid ratio of 20 mL g− 1 to evaluate the role of temperature in leaching efficiency. Although 3 M HCl was used to evaluate liquid–solid ratios, the same leaching efficiency was demon Fig. 7. Plant-available toxic, heavy, and trace metals (and rare-earth element strated with 1.5 M and 3 M HCl (Fig. 8b); therefore, we used 1.5 M HCl and critical element) concentrations in 6 M HCl leachate from three phosphate for the temperature experiments to reduce acid consumption. Nie et al. fertilizers. showed that higher temperature can have a positive effect on REE leaching from phosphorite by reducing the solid film diffusion control leaching, suggesting the REE leaching reached a maximum value and process. In contrast, we observed no significant change in REE leaching this correlates with the total metal leaching values (Figure S5). Leaching from 25 to 65 ◦ C (Fig. 8c). Higher temperatures can also lead to an in with HCl concentrations below 1.5 M led to significantly lower REE crease in undesirable element leaching such as Al, Si, and Fe from Al2O3, leaching efficiencies. This is likely because low HCl concentrations may SiO2, Fe2O3, along with other impurities. In addition, leaching at not be able to completely dissolve the fluorapatite minerals due to the lower temperatures can help save energy. Based on these results, room neutralization of acidity, where consumption of free protons limits the temperature conditions were used for the REE separation system leach Fig. 8. REE leaching optimization from three phosphate fertilizers for: (a) liquid–solid ratio, (b) HCl concentration, (c) temperature, and (d) leaching time. 8 C.M. Tummala et al. Separation and Puri cation Technology 357 (2025) 130139 solutions. 3.8. Proof-of-concept REEs recovery with TODGA-associated organosilica sorbent media 3.6.4. Leaching kinetics (24 h) To study the REE release time, leaching experiments were conducted 3.8.1. TODGA media characterization with 1.5 M HCl at a liquid–solid ratio of 20 mL g− 1 at room temperature Media surface morphology was characterized by SEM-EDS. The mass (25 ◦ C) with results shown in Fig. 8d. The REE percent leached after 10 of nitrogen species increased from near zero for the unmodified solid min are as follows: Montana fertilizer (96%) > Utah fertilizer (82%) > support to around 3.4 wt% of the mass of the sorbent after TODGA Florida fertilizer (76%). This suggests that most of the REE from the impregnation (SEM-EDS results shown in Figure S9). Praneeth, Sakr phosphate fertilizers were leached in the first ten minutes; however, and et al. provides additional characterization. XPS analysis confirms additional 5% leaching of Y, La, Ce, and Nd continued for up to 240 min that the TODGA was responsible for the nitrogen peak with a measured in the Florida and Utah fertilizers (Figure S8). The optimal time to leach nitrogen atomic percentage of 2.38%. Moreover, the C atomic percent the REEs is around 15 min with continuous stirring. age was increased from 62.47% to 66.90% with TODGA on organosilica. Only Si, O, and C peaks were identified on the unmodified organosilica 3.7. Solid residue characterization after leaching (mostly quartz). In addition, the surface area of organosilica decreased from 557 to 74 m2/g, pore volume decreased from 0.68 to 0.13 cm3 g− 1, and the pore A significant amount of solid residue remains after 24 h leaching, size decreased from 4.3 to 3.87 nm, confirming incorporation of TODGA indicating that some of the mineral phases of the fertilizers are not into organosilica media. completely dissolved during the acid leaching process. The amount of TODGA-organosilica has previously been shown to be reusable in fertilizer solid residue for Montana fertilizer, Florida fertilizer, and Utah packed-bed column experiments with 5 M nitric acid for at least 3 fertilizer was 25.8, 38.1, and 20.1%, respectively. The filtered fertilizer loading and strip cycles without any loss of efficiency. Similar residue was dried at 50 ◦ C before conducting mineral phase analysis ligand-associated organosilica sorbents impregnated with bisethylhex (XRD and XRF). Fluorapatite peaks were no longer present in Montana yamido DTPA have shown less than 10% loss of efficiency after six fertilizer, Florida fertilizer, and Utah fertilizer after leaching, indicating loading and strip cycles (Hovey, Dardona et al., 2021), demonstrating most of the fluorapatite minerals were dissolved or altered during the the resilience of the organosilica solid support. leaching (Fig. 9). Notably, significant quartz mineral peaks were observed in the left-over solid residue, which confirms quartz minerals 3.8.2. Two stage recovery: (1) precipitation and (2) TODGA-organosilica did not dissolve during the leaching process. Based on this result, the solid phase extraction reaction proposed in equation 2 is plausible during the leaching process. It also confirms that most REEs are present in fluorapatite min 3.8.2.1. REE precipitation (pH increase to 2.5). A sodium hydroxide erals, which can be easily extracted, and with only a minor amount in precipitation was conducted to separate REEs from the filtered phos the undissolved residue (quartz minerals). phate fertilizer leachates produced with 1.5 M HCl at room temperature XRF analysis (Table 1) shows a reduction in percent CaO and P2O5 in for 24 h. Initial metal concentrations for the precipitation and adsorp the residue after leaching, suggesting that these oxides are effectively tion experiments using this solution are shown in Figure S10). The REE dissolved during the leaching process. Conversely, there is an increase in precipitate was then dissolved in HCl and tested for TODGA-organosilica the SiO2, Fe2O3, and K2O percentages, suggesting these oxides remain recovery. More than 95% of the REEs in all three fertilizer leachates undissolved or only undergo partial dissolution, and the increase is a precipitated when the pH was raised to 2.5 (Fig. 10a). Below pH 1.5, the result of the total mass of residue decreasing by >70% due to dissolution overall REE precipitation percentage was less than 15% for all three of other mineral phases. In addition, chlorine was detected in the res fertilizers. The REE precipitation percentage was found to be high be idue, which is likely from the HCl used for leaching. tween pH of 1.5 and 2.25. This sudden spike in the precipitation of REEs between pH 1.5 and 2.25 could be due to co-precipitation along with Fe, Ca10 (PO)6.F2 + 20HCL→10CaCl2 + 6H3 PO4 + 2HF (2) which precipitates Fe(OH)3 above pH 1.5, as significant iron pre cipitation was measured above pH 2. Zhang et al., found that the REE removal from leachate solutions can also be due to the adsorption of the REE on the precipitated surfaces of iron and aluminum. Hydrolysis is another potential precipitation mechanism through the formation of REE(OH)2+, REE(OH)+ 2 , and REE(OH)3; however, REEs have pKa values above 3, making this reaction pathway unlikely in this system. In addition to REEs, concentrations of other metals such as Pb, Zn, Fe, Cu, Ni, Co, Cr, V, Mg, As, and Cd, were also measured. A small percentage of Pb, As, Mg, and Cu precipitation was observed while other metals remained mostly in solution. The precipitate formed at pH 2.5 had high percentages of Ca, Cl, Al, P, and Fe along with the REE (Table S4). From the mass balance before and after precipitation ex periments, an estimated 96.7 ± 0.19% of REEs in Montana, 94.0 ± 0.27% in Florida and 97.8 ± 0.21% in Utah were transferred to the precipitate. The separated REEs were further purified using solid–liquid extraction, while the REE-free leachate solution after REE precipitation is highly enriched in phosphate and depleted of most metals and could be readily utilized as either a liquid phosphoric acid fertilizer or pro cessed into solid phosphate fertilizer. Research studies have shown that application of phosphoric acid in agriculture fields with alkaline soil conditions enhanced crop growth due to an increase in phosphate bioavailability [18,36]. The phosphate in this solution could also Fig. 9. XRD analysis of rock phosphate fertilizers (before leaching – black line) potentially be loaded to a biodegradable solid support (such as iron- and solid residue (after HCl leaching – green line). Note: Q represents quartz, F coated waste nutshells) and used as a slow-release fertilizer. represents fluorapatite, and G represents gypsum. 9 C.M. Tummala et al. Separation and Puri cation Technology 357 (2025) 130139 Table 1 Comparison of the elemental oxide percentages in the phosphate fertilizers before and after leaching with 1.5 M HCl. Oxides Montana fertilizer Florida fertilizer Utah fertilizer Before leaching (%) After leaching (%) Before leaching (%) After leaching (%) Before leaching (%) After leaching (%) CaO 18.4 18 19.5 6.4 27.8 8.2 P2O5 42.4 27.6 31.3 11 29.3 7.1 Al2O3 20 ND 26.6 ND 16.9 ND SiO2 11.3 39.1 17.1 75 20.3 70 Fe2O3 1.8 4 2.9 3.6 1 2.7 K2O 4 7.2 2.2 2 3.9 9.5 SO3 1.4 1.2 − ND 0.16 0.103 Y2O3 0.016 0.014 0.005 0.004 0.02 0.01 Cl 1.4 1.3 1.04 Others 0.7 1.5 0.4 0.7 0.6 1.3 Fig. 10. REE separation and redissolution from phosphate fertilizer leachate via (a) precipitation (black squares for Montana, blue triangles for Utah, and red circles for Florida) and (b) dissolving precipitate in 4 M HCl. 3.8.2.2. REE content in dissolved precipitate. The REE precipitate formed mixture of REEs and very small concentrations of Al and Fe, with all in the previous step was dissolved in 4 M HCl to use in TODGA sorbent other elements (Ca, Pb, Zn, Cu, Ni, Co, Cr, V, Mg, As, and Cd) below media experiments (Fig. 10b). In all three fertilizers, Y had the highest detection limit (confirming high REE selectivity). This process increased REE concentration, followed by lanthanum, cerium, and neodymium. the REE concentration in the eluted solution compared to the initial The total REE concentration was highest in the dissolved Utah precipi digestion solution (Fig. 11). The percentage of individual REE cations in tate followed by the Montana and Florida precipitates and correlated the strip solution increased from 0.38 to 7%, 1 to 5%, and 2% to 12% for with results from the leaching experiments. the FL, MT, and UT samples, respectively, when compared to the leach solution. The overall mass balance of REEs in each step is shown in 3.8.3. REE recovery from dissolved precipitate and filtered leachate with Figure S11. TODGA-organosilica In addition to the dissolved precipitate, a sample of leachate was Batch adsorption experiments showed TODGA media was able to simply filtered of residue solids and combined with TODGA-organosilica sorb REE directly from the REE-laden dissolved precipitate solution media without the precipitation and dissolution steps. Fig. 12 shows a (Fig. 11). The highest percent REE removal was for the Florida fertilizer comparison of the REE sorption from filtered leachate for 6 M HCl, followed by Montana and Utah. Higher selectivity towards HREEs is HNO3, and H2SO4. clearly observed and is desirable since HREEs are more valuable than LREEs. This is likely due to an ion exchange mechanism, where HREEs 3.8.4. Proof-of-concept for resin-in-pulp ternary system with TODGA can substitute for the LREEs sorbed to TODGA in acidic conditions. organosilica media Furthermore, it has been reported that tetrabutyl diglycolamide (a The critical elements along with REEs recovered from the direct derivative of TODGA) can form neutral complex species with REEs in the adsorption resin-in-pulp (RIP) method are shown in Fig. 13. TODGA presence of high chloride concentrations as shown in equation 3. media was able to extract REEs in high molarity acids (>1.5 M) for all three mineral acids tested. TODGA media adsorbed HREEs in H2SO4 but REE3+ + TODGA + 3Cl− →REE(TODGA)Cl3 (3) the efficiencies were lower when compared to the performance in the Elution proof-of-concept experiments showed that as high as 70% of HCl and HNO3 acidic leachates (6 M HNO3 > 6 M HCl > 6 M H2SO4), sorbed REEs can be desorbed from the TODGA media when high-purity likely due to the formation of phosphogypsum and the coprecipitation of water is used for elution. Higher than 90% recovery of sorbed REEs has REEs. been reported with packed bed column experiments using coal fly ash In the resin-in-pulp method, TODGA recovered 10–40% higher leachate solutions with high-purity water. Batch experiments often concentrations of Pr, Nd, Sm, Eu and Y in 6 M HNO3, 6 M HCl, and 6 M underpredict stripping potential, since no additional stripping will occur H2SO4 (Fig. 13) compared to recovery from the filtered leachate once the strip solution becomes saturated. The eluted solution is a (Fig. 12). In addition, RIP recovered slightly higher concentrations of V, 10 C.M. Tummala et al. Separation and Puri cation Technology 357 (2025) 130139 Fig. 13. Resin-in pulp recovery of REEs from unfiltered acid leach slurry using the Utah fertilizer sample with three acids: 6 M HCl (black), 6 M HNO3 (red), and 6 M H2SO4 (blue). Cr, Mn, Ni, Cu and Ga in the 6 M HNO3, 6 M HCl, and 6 M H2SO4 sys tems. The large error bars in the H2SO4 system (especially for V, Cr, Mn, Ni, Cu, Ga, Ag, and Ba) are due to the instability of the sulfate system, which results in significant precipitation and error between replicates. This increase in efficiency in the RIP over filtered leachate systems might be related to the simultaneous leaching and adsorption process reducing the effective ion concentrations competing for adsorption sites. For example, the final ionic strength of the leach solution is higher than the ionic strength of the early time behavior (before complete leaching). The Fig. 11. REE extraction efficiency for TODGA media from dissolved pre remaining filtrate after TODGA-organosilica adsorption is a mostly REE- cipitates.(a) REE sorption from three dissolved precipitates; (b) REE desorption free phosphate-rich solution that may be utilized as a feedstock for with high-purity water. traditional phosphate fertilizer production. Most REE recovery processes involve four steps: (1) acid extraction, (2) filtration, (3) pH increase to remove Fe and Al and to enable ligand attachment (pKa values are usually above 2 for most ligands used), and (4) either solvent extraction or solid–liquid extraction to recover REEs from the aqueous system. In this work, we have proposed a method to recover the REEs directly from an acid system (as high as 6 M acid) without the need for a pH increase to enable ligand complexation. The resin-in-pulp system (direct addition of sorbent media to acid leach slurry) also eliminates the need for filtering the fly ash fines (which is challenging and energy intensive) and replaces this step with simple sieving, where the slurry can be poured through a sieve and the larger sorbent grains with attached REEs are collected in the sieve and the smaller fly ash particles and non-REE metals are passed through the sieve. The proposed process represents a step forward in REE recovery from phosphate minerals from current industry standards that rely on using sulfuric acid for rock phosphate dissolution and results in significant co- precipitation with phosphogypsum. The use of a resin-in-pulp recovery system with TODGA media (especially with nitric or hydrochloric acid instead of sulfuric acid) could enable economic recovery of REEs during phosphoric acid production. Fig. 12. REE recovery from filtered acid leachate using the Utah fertilizer 4. Conclusions sample with three acids: 6 M HCl (black), 6 M HNO3 (red), and 6 M H2SO4 (blue). A framework was developed to characterize, extract, and recover REEs in acidic conditions from rock phosphate fertilizers. The total and extractable cations (46 elements) from three phosphate rock types were evaluated with REE concentrations as high as 1,200 mg kg− 1 for a Utah 11 C.M. Tummala et al. Separation and Puri cation Technology 357 (2025) 130139 sample. Yttrium, lanthanum, cerium, neodymium and praseodymium interests or personal relationships that could have appeared to influence comprise more than 80% of the TREE concentration. REEs can be effi the work reported in this paper. ciently extracted in less than 10 min with 1.5 M HCl. Fluorapatite dissolution is necessary to achieve high leaching efficiencies, as most Acknowledgements REEs are incorporated in these minerals. More than 95% of the REEs in the leachate were precipitated when the solution pH was raised to 2.5. A We thank the U.S. Department of Energy (DE-SC0021702) and U.S. newly developed TODGA-organosilica sorbent was used to recover REEs Army Engineer Research Development Center (W912HZ-21-2-0048) for from fertilizer leachates via (1) precipitate dissolution, (2) TODGA- partially supporting this research work. Thanks to Dr. Shawn McElmurry organosilica sorption from filtered leachate, and (3) a resin-in-pulp for microwave digestor equipment and lab use and Dr. Stephanie Brock system with sorbent, acid, and fertilizer in a ternary system. Overall, for BET surface area analysis. REE recovery from phosphate fertilizers could potentially reduce REEs and other metal cation enrichment in agriculture fields while providing Appendix A. Supplementary material an additional potential REE feedstock. There are several important implications from this study. First, the Supplementary data to this article can be found online at https://doi. high REE concentration in rock phosphate fertilizers justified further org/10.1016/j.seppur.2024.130139. study to develop sustainable recovery strategies. REE leaching from these materials requires less acid consumption than more researched Data availability options such as coal fly ash. At the same time, REE recovery could also offer an opportunity to reduce the heavy and toxic metal load to agri Data will be made available on request. cultural fields. The use of new sorbents that can recover metal ions from highly acidic waste streams without the need for pH increases can References provide a more simplified recovery system. The use of a ternary resin-in- pulp system also eliminates the initial filtration step, providing an Artiushenko, O., Zaitsev, V., Rojano, W. S., Freitas, G. A., Nazarkovsky, M., Saint’Pierre, T. D., & Kai, J. (2021). Rationally designed dipicolinate- additional simplification to complex recovery treatment systems. functionalized silica for highly efficient recovery of rare-earth elements from e- Although promising, several limitations from this study require waste. Journal of Hazardous Materials, 408, 124976. further investigation. The TODGA-organosilica sorbent media has been C.R. Cánovas, S. Chapron, G. Arrachart, S. Pellet-Rostaing, Leaching of rare earth elements (REEs) and impurities from phosphogypsum: A preliminary insight for cycled two times with a coal fly ash leachatewithout loss of effi further recovery of critical raw materials, J. Clean. Prod. 219 (2019) 225–235. ciency; however, large numbers of cycles will be needed to make this S. Cao, C. Zhou, J. Pan, C. Liu, M. Tang, W. Ji, N. Zhang, Study on influence factors technology cost-effective and potential long-term degradation of the of leaching of rare earth elements from coal fly ash, Energy Fuel 32 (7) (2018) 8000–8005. media must be further investigated. The TODGA ligand is also not M.E. Case, R.V. Fox, D.L. Baek, B.J. Mincher, C.M. Wai, Extraction behavior of currently produced in large commercial quantities and efficiencies in selected rare earth metals from acidic chloride media using tetrabutyl TODGA synthesis would need to be improved to reduce the cost of the diglycolamide, Solvent Extr. Ion Exch. 35 (7) (2017) 496–506. Dardona, M., & Dittrich, T. M. (2019, May). Investigating the potential for final media. The TODGA media also has fairly slow desorption rates recovering REEs from coal fly ash and power plant wastewater with an engineered when using high-purity water as the elution agent. Although water is sorbent. In World Environmental and Water Resources Congress 2019. Wayne State low-cost, the slow desorption kinetics will affect the efficiency of scaled Univ., Detroit, MI (United States). up systems. M. Dardona, S.K. Mohanty, M.J. Allen, T.M. Dittrich, From ash to oxides: Recovery of rare-earth elements as a step towards valorization of coal fly ash waste, Sep. Future research can address these limitations by investigating: (1) Purif. Technol. 314 (2023) 123532. recycling performance of TODGA-organosilica media in a strong acid P. Emsbo, P.I. McLaughlin, G.N. Breit, E.A. du Bray, A.E. Koenig, Rare earth system, (2) the possibility of sequential extraction with combinations of elements in sedimentary phosphate deposits: solution to the global REE crisis? Gondw. Res. 27 (2) (2015) 776–785. different acids and acid strengths, (3) modifications to the TODGA M.S. Gasser, Z.H. Ismail, E.A. Elgoud, F.A. Hai, O.I. Ali, H.F. Aly, Process for structure that adjust the sorption pocket (bite angle) and result in faster lanthanides-Y leaching from phosphogypsum fertilizers using weak acids, desorption kinetics, (4) the use of alternative eluents (e.g., oxalic acid, J. Hazard. Mater. 378 (2019) 120762. M. Gergoric, C. Ekberg, M.R.S.J. Foreman, B.M. Steenari, T. Retegan, citric acid, other organic ligands) that could either selectively strip REEs Characterization and leaching of neodymium magnet waste and solvent extraction from the loaded resin or result in faster desorption, and (5) the synthesis of the rare-earth elements using TODGA, J. Sustainable Metall. 3 (2017) 638–645. of lower-cost ligands that effectively sorb REEs at low pH. M.W. Guidry, F.T. Mackenzie, Experimental study of igneous and sedimentary apatite dissolution: Control of pH, distance from equilibrium, and temperature on dissolution rates, Geochim. Cosmochim. Acta 67 (16) (2003) 2949–2963. CRediT authorship contribution statement J.L. Hovey, T.M. Dittrich, M.J. Allen, Coordination chemistry of surface-associated ligands for solid–liquid adsorption of rare-earth elements, J. Rare Earths 41 (1) (2023) 1–18. Chandra M. Tummala: Conceptualization, Methodology, Investi J.L. Hovey, M. Dardona, M.J. Allen, T.M. Dittrich, Sorption of rare-earth elements gation, Writing – original draft, Writing – review & editing. Mohammed onto a ligand-associated media for pH-dependent extraction and recovery of Dardona: Methodology, Writing – review & editing. Sai Praneeth: critical materials, Sep. Purif. Technol. 258 (2021) 118061. W. Jiao, W. Chen, A.C. Chang, A.L. Page, Environmental risks of trace elements Methodology, Writing – review & editing. Ahmed K. Sakr: Methodol associated with long-term phosphate fertilizers applications: A review, Environ. ogy, Writing – review & editing. Dimitrios Kakaris Porter: Conceptu Pollut. 168 (2012) 44–53. alization, Methodology. Timothy M. Dittrich: Conceptualization, R. Karan, M. Babu, T. Sreenivas, Development of process scheme for recovery of Methodology, Resources, Writing – original draft, Writing – review & rare earths from leachate of coal flyash, Clean. Chem. Eng. 4 (2022) 100078. J.J. Kasper-Zubillaga, B. Acevedo-Vargas, O.M. Bermea, G.O. Zamora, Rare earth editing. elements of the Altar Desert dune and coastal sands, Northwestern Mexico, Geochemistry 68 (1) (2008) 45–59. Declaration of competing interest S.L. Liu, H.R. Fan, X. Liu, J. Meng, A.R. Butcher, L. Yann, X.C. Li, Global rare earth elements projects: New developments and supply chains, Ore Geol. Rev. 157 (2023) 105428. The authors declare the following financial interests/personal re Y. Ma, L. Kuang, X. He, W. Bai, Y. Ding, Z. Zhang, Z. Chai, Effects of rare earth lationships which may be considered as potential competing interests: oxide nanoparticles on root elongation of plants, Chemosphere 78 (3) (2010) 273–279. Timothy Dittrich reports financial support was provided by US Army T.M. McBeath, R.D. Armstrong, E. Lombi, M.J. McLaughlin, R.E. Holloway, Engineer Research and Development Center Coastal and Hydraulics Responsiveness of wheat (Triticum aestivum) to liquid and granular phosphorus Laboratory Field Research Facility. Timothy Dittrich reports financial fertilizers in southern Australian soils, Soil Res. 43 (2) (2005) 203–212. support was provided by US Department of Energy. If there are other authors, they declare that they have no known competing financial 12 C.M. Tummala et al. Separation and Puri cation Technology 357 (2025) 130139 M. Mohammadkhani, M. Noaparast, S.Z. Shafaei, A. Amini, E. Amini, H. Abdollahi, A. Soussi-Baatout, M. Hichri, A. Bechrifa, I. Khattech, Test and calibration Double reverse flotation of a very low grade sedimentary phosphate rock, rich in processes for the differential reaction calorimeter (DRC): Application: Dissolution carbonate and silicate, Int. J. Miner. Process. 100 (3–4) (2011) 157–165. of calcium fluorapatite in the hydrochloric acid, Thermochim. Acta 580 (2014) D. Nie, A. Xue, M. Zhu, Y. Zhang, J. Cao, Separation and recovery of associated rare 85–92. earths from the Zhijin phosphorite using hydrochloric acid, J. Rare Earths 37 (4) C.M. Tummala, M. Dardona, S. Praneeth, S.K. Mohanty, T.M. Dittrich, Iron-coated (2019) 443–450. nutshell waste bioadsorbents: Synthesis, phosphate remediation, and subsequent N. Otero, L. Vitoria, A. Soler, A. Canals, Fertilizer characterization: major, trace fertilizer application, Environ. Res. 240 (2024) 117468, https://doi.org/10.1016/j. and rare earth elements, Appl. Geochem. 20 (8) (2005) 1473–1488. envres.2023.117468. S. Park, M. Kim, Y. Lim, J. Yu, S. Chen, S.W. Woo, H.S. Kim, Characterization of J. Wang, G. Chu, Phosphate fertilizer form and application strategy affect rare earth elements present in coal ash by sequential extraction, J. Hazard. Mater. phosphorus mobility and transformation in a drip-irrigated calcareous soil, J. Plant 402 (2021) 123760. Nutr. Soil Sci. 178 (6) (2015) 914–922. L. Pietrelli, B. Bellomo, D. Fontana, M.R. Montereali, Rare earths recovery from W.C. Wilfong, T. Ji, Y. Duan, F. Shi, Q. Wang, M.L. Gray, Critical review of NiMH spent batteries, Hydrometall. 66 (1–3) (2002) 135–139. functionalized silica sorbent strategies for selective extraction of rare earth G. Prameswara, I. Trisnawati, P. Mulyono, A. Prasetya, H.T.B.M. Petrus, Leaching elements from acid mine drainage, J. Hazard. Mater. 424 (2022) 127625. behaviour and kinetic of light and heavy rare earth elements (REE) from zircon S. Wu, L. Wang, L. Zhao, P. Zhang, H. El-Shall, B. Moudgil, L. Zhang, Recovery of tailings in Indonesia, JOM 73 (2021) 988–998. rare earth elements from phosphate rock by hydrometallurgical processes–A S. Praneeth, A.K. Sakr, M. Dardona, C.M. Tummala, P.K. Roy, T.M. Dittrich, (in critical review, Chem. Eng. J. 335 (2018) 774–800. press). Selective separation and recovery of rare-earth elements (REEs) from acidic S. Wu, L. Zhao, L. Wang, X. Huang, Y. Zhang, Z. Feng, D. Cui, Simultaneous solutions and coal fly ash leachate by novel TODGA-impregnated organosilica recovery of rare earth elements and phosphorus from phosphate rock by media, Chemical Engineering Journal. phosphoric acid leaching and selective precipitation: Towards green process, Praneeth, S., Sakr, A., Dardona, M., Tummala, C., Allen, M.J., Dittrich, T.M. J. Rare Earths 37 (6) (2019) 652–658. (2023). Selective separation and extraction of rare earth elements (REEs) from C. Xie, Y. Xiao, C. He, W.S. Liu, Y.T. Tang, S. Wang, R.L. Qiu, Selective recovery of acidic solutions by using novel N, N, N′, N′-tetraoctyl diglycolamide (TODGA) rare earth elements and value-added chemicals from the Dicranopteris linearis bio- grafted organosilica media. REMPLEX 2023 Global Summit, November 13-17, 2023. ore produced by agromining using green fractionation, J. Hazard. Mater. 443 E. Quijada-Maldonado, J. Romero, Solvent extraction of rare-earth elements with (2023) 130253. ionic liquids: Toward a selective and sustainable extraction of these valuable F. Yang, F. Kubota, Y. Baba, N. Kamiya, M. Goto, Selective extraction and recovery elements, Curr. Opin. Green Sustainable Chem. 27 (2021) 100428. of rare earth metals from phosphor powders in waste fluorescent lamps using an S.J. Ramos, G.S. Dinali, T.S. de Carvalho, L.C. Chaves, J.O. Siqueira, L. ionic liquid system, J. Hazard. Mater. 254 (2013) 79–88. R. Guilherme, Rare earth elements in raw materials and products of the phosphate Q. Yang, Z. Li, X. Lu, Q. Duan, L. Huang, J. Bi, A review of soil heavy metal fertilizer industry in South America: Content, signature, and crystalline phases, pollution from industrial and agricultural regions in China: Pollution and risk J. Geochem. Explor. 168 (2016) 177–186. assessment, Sci. Total Environ. 642 (2018) 690–700. C. Ramprasad, W. Gwenzi, N. Chaukura, N.I.W. Azelee, A.U. Rajapaksha, D. Yu, R. Du, J.-C. Xiao, S. Xu, C. Rong, S. Liu, Theoretical study of pKa values for M. Naushad, S. Rangabhashiyam, Strategies and options for the sustainable trivalent rare-earth metal cations in aqueous solution, Chem. A Eur. J. 122 (2) recovery of rare earth elements from electrical and electronic waste, Chem. Eng. J. (2017) 700–707. 442 (2022) 135992. W. Zhang, R.Q. Honaker, Rare earth elements recovery using staged precipitation S. Ravi, S.Y. Kim, Y.S. Bae, Novel benzylphosphate-based covalent porous organic from a leachate generated from coarse coal refuse, Int. J. Coal Geol. 195 (2018) polymers for the effective capture of rare earth elements from aqueous solutions, 189–199. J. Hazard. Mater. 424 (2022) 127356. Z. Zhang, Y. Jiang, H. Niu, J. Xing, S. Yan, A. Li, X. Zhao, Enrichment of rare earth U. Ryszko, P. Rusek, D. Kołodyńska, Quality of phosphate rocks from various elements in the early Cambrian Zhijin phosphorite deposit, SW China: Evidence deposits used in wet phosphoric acid and P-fertilizer production, Materials 16 (2) from francolite micro-petrography and geochemistry, Ore Geol. Rev. 138 (2021) (2023) 793. 104342. B. Saha, K. Eliason, D. Golui, J. Masud, A.N. Bezbaruah, S.M. Iskander, Rare earth X. Zheng, C. Wang, J. Dai, W. Shi, Y. Yan, Design of mesoporous silica hybrid elements in sands collected from Southern California sea beaches, Chemosphere materials as sorbents for the selective recovery of rare earth metals, J. Mater. (2023) 140254. Chem. A 3 (19) (2015) 10327–10335. SHEET, F. (2022). Securing a Made in America Supply Chain for Critical Minerals. The White House. 13