Batteries Handout PDF
Document Details
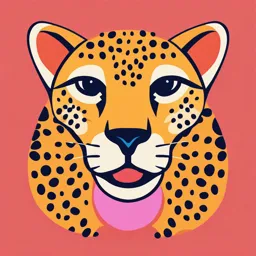
Uploaded by HandierBoltzmann
University of Nottingham
Darren Walsh
Tags
Summary
This document is a chemistry lecture handout on the topic of batteries. It covers the basic operating principles of batteries, including historical developments and the role of lithium-ion batteries. The handout uses diagrams and figures to illustrate key concepts.
Full Transcript
Frontiers in Chemistry CHEM1008 Electrochemistry and Devices 2 Batteries Darren Walsh Room A09, GSK Carbon Neutral Laboratory for Sustainable Chemistry Email: [email protected] Lecture 2 Learning Outcomes o To...
Frontiers in Chemistry CHEM1008 Electrochemistry and Devices 2 Batteries Darren Walsh Room A09, GSK Carbon Neutral Laboratory for Sustainable Chemistry Email: [email protected] Lecture 2 Learning Outcomes o To understand the basic operating principles of batteries o To know that secondary batteries can be recharged – primary batteries cannot o To appreciate why lithium is used in most of today’s batteries o To understand the developments that led to the invention of the Li-ion battery o To be aware of some of the challenges facing today’s Li-ion batteries o To know that “beyond-Li-ion technologies are coming along that use more sustainable and lighter materials, and may boost the energy stored in batteries by a large amount Batteries are an enabling energy-storage technology Batteries o Like fuel cells, batteries are galvanic devices –devices that generate current due to the release of energy from a spontaneous reaction o The Baghdad battery – discovered in 1936 – is a 2000-year old artefact containing an iron rod wrapped in copper, which may have been a battery Officially, the first battery was the voltaic pile (1799) On the Zn anodes (oxidation) Zn(s) → Zn2+(aq) + 2e– On the Cu cathodes (reduction) 2H+(aq) + 2e– → H2(g) Net: Zn(s) + 2H+(aq) → Zn2+(aq) + H2(g) The electrolyte was salt water and a stack produced about 6 V – each element has a thermodynamic cell potential of 0.76 V (determined by ∆rG for the net reaction) The Daniell Cell On the Zn anodes (oxidation) Zn(s) → Zn2+(aq) + 2e– On the Cu cathodes (reduction) Cu2+(aq) + 2e– → Cu(s) Net: Zn(s) + 2Cu2+(aq) → Zn2+(aq) + Cu(s) Daniell went one better than Volta in 1836 and changed the electrolyte from salt water, resulting in the formation of Cu(s) instead of H2(g), increasing the voltage to 1.1 V History since then has involved changing the chemistry – the principles basically remain the same 1799 Voltaic pile 1946 Neumann: sealed NiCd 1836 Daniell cell 1960s Alkaline, rechargeable NiCd 1859 Planté: rechargeable lead-acid 1970s Lithium, sealed lead acid 1868 Leclanché: carbon zinc wet 1990 Nickel metal hydride (NiMH) cell 1991 Lithium ion 1888 Gassner: carbon zinc dry cell 1992 Rechargeable alkaline 1898 Commercial torch D cell 1999 Lithium ion polymer 1899 Junger: nickel cadmium cell Today What is “beyond Li-ion”? Why do we use lithium? o Lithium is highly electropositive (is readily gives up its electrons) o Reactions involving lithium oxidations are very energetic (exergonic) o The high ∆rG results in high Ecell values (remember ∆rG = –zFEcell) Tesla Model S = 100 kWh = 360 MJ = 600 fireworks The Start: Stanley Whittingham’s Li-TiS2 battery o Whittingham was trying to make a rechargeable battery with a Li negative electrode and TiS2 positive electrode o Recharging pushed Li+ ions back to the negative electrode, reforming Li metal Stanley Whittingham’s Li-TiS2 battery o The problem was that the Li+ ions, when reduced to Li metal, didn’t form a nice even layer o If the metal “dendrites” touch the positive electrode, a short circuit formed, uncontrolled discharge occurred o Revolutionary but unusable The key was to not use dangerous metallic Li o By returning to the old and well- understood chemistry of ion intercalation in graphite, Akira Yoshino developed negative electrodes o Lithiated graphite electrodes were much more stable and safer than those containing pure Li metal The 3rd part of the puzzle – LiCoO2 positive electrodes o In LiCoO2, Li+ ions occupy octahedral sites and can hop from site to site o Reaction between lithiated graphite negative electrodes and LiCoO2 gives cell voltage of 3.6 V o much bigger than achievable using Whittingham’s TiS2 electrodes The operating principles of the modern Li-ion battery Charging Reaction: LiCo3+O2 + C6 → Co4+O2 + LiC6 Discharging Reaction: Co4+O2 + LiC6 → LiCo3+O2 + C6 Half the Li+ ions can be removed from LiCoO2 during each discharge cycle – take out more and it becomes mechanically unstable A fortuitous phenomenon is that solid-electrolyte interphases (SEIs) form and protect the electrodes o Lithiated graphite and LiCoO2 is so reactive that the liquid electrolyte, which is an organic solvent containing a Li salt, reacts with the electrodes o A layer of breakdown products coats and protects the electrodes o These layers remain conductive to Li+ ions Painstaking work by the 3 men led in 2019 to… Of course, things can and do go wrong – and it’s complicated We don’t have time in this module to consider all of these effects What is beyond Li-ion technology? o For more energy per unit weight of battery, we need lighter positive- electrode materials (O2 and/or S?) o The future supply of Li is not guaranteed – we need alternatives (Na, K, Mg and/or Ca?) o LiC6 carries a lot of extra weight not contributing to the energy of the battery – how about metallic Li? o Each of these technologies requires a huge amount of new work to make them viable