Basics of Cancer Biology PDF
Document Details
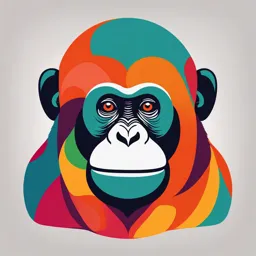
Uploaded by ProperForgetMeNot
Tags
Summary
This document provides an overview of cancer biology, covering topics such as oncogenes, tumor suppressor genes, DNA repair mechanisms, transformation, and the genetics of cancer. It also discusses viral oncogenes and the role of various viruses in cancer development.
Full Transcript
Basics of Cancer Biology Cancer Uncontrolled growth of cells Three characteristics of cancer cells; a. Immortalization, b. transformation and c. Metastasis Types: Benign Malignant Leukemia: Overproduction of leucocytes Sarcoma: cancer of muscle an...
Basics of Cancer Biology Cancer Uncontrolled growth of cells Three characteristics of cancer cells; a. Immortalization, b. transformation and c. Metastasis Types: Benign Malignant Leukemia: Overproduction of leucocytes Sarcoma: cancer of muscle and cartilage Carcinoma: Cancers of epithelial tissue such as glands, breast, skin etc. Mesothelioma: tumors derived from the mesothelial cells lining the peritoneum Glioma: tumors derived from glial cells Germinoma: tumors derived from germ cells normally found in the testicles and ovaries Choriocarcinoma: cancer of placenta Oncogene: gene promote tumor formation. Ex. v-onc (1st oncogene was discovered from the Avian Sarcoma Virus called src) Proto-oncogene: cellular normal gene that causes cancer under altered condition (s). Tumor suppressor genes are gene that restrain cell proliferation. Ex. Rb, p53 etc.2 Oncogene discovery Shotgun cloning ras from bladder carcinoma met oncogene from hepatocarcinoma hst oncogene from stomach carcinoma Screening of oncogene using probe Cloning in Cut DNA Size vector by RE (genomic fractiona (EcoRI, library tion on BamHI) generation) Gel 3 Changes When a Cell Becomes Cancer Cell Immortalization Oncogenesis is the process of malignant transformation leading to the formation of a cancer or tumor (carcinogenesis). Cancer cells have lost their growth control Types of changes: Immortalization: indefinite growth > Transformation: deviation from normal growth requirements and constrains; independent of anchorage and serum growth factors, not inhibited by density/contact > Metastasis: invasion of normal tissues In vitro culture, normal cells Senescence and cease of growth Crisis Immortalization after surviving crisis; growth characters change and establishment of “cell line” (permanently established cell culture) Immortalized cells are “non-tumorigenic; still depend on anchorage & growth factor; density-dependent inhibition; Monolayer 4 5 Proto-oncogene to Oncogene In appropriately activation of Proto-oncogene generates Oncogene Mutational change or Overexpression Oncogene protein products include Transcription factors trans membrane proteins. 6 Changes When a Cell Becomes Cancer Cell Transformed Cell Lines Derived from tumors More changes in transformed than immortalized cell lines > Grow in much less restricted conditions > Reduced growth factor dependence > Less anchorage dependence: round-up vs. spread out > Forms foci instead of monolayer > Tumorigenic [Fig 28-2; Normal and transformed fibroblast cell lines] 7 8 Changes When a Cell Becomes Cancer Cell Multiple Genetic Changes Changes lead the conversion of normal cells transformed cells: Multiple genetic changes: 6-7 events over ~40 years Factors (carcinogens) that increase the conversion: Initiate/Promote suggest stages in cancer development Genes that cause transformation: Oncogenes (100+) Viral oncogenes and cellular counterparts (proto-oncogenes) Gain-of-function or Activated Tumor suppressor genes (~10) Loss-of-function or Inactivated How are oncogenes activated and tumor suppressor gene inactivated? 9 Transforming Viruses Carry Oncogenes Transformation may result from tumor virus infection thus “oncogenes” Tumor viruses > Polyomavirus/dsDNA/6Kb/viral gene/Inactivate tumor suppressor gene > Human papillomavirus/dsDNA/8Kb/E6 & E7 genes/Early viral genes/Inactivate tumor suppressor gene > Adenovirus/dsDNA/37Kb/E1A & E1B genes/Early viral genes/Inactivate tumor suppressor gene > Retrovirus/ssRNA/6-9Kb/Individual genes/Cellular origin/Activate oncogenic pathway 10 Tumor viruses From cellular genes and therefore may mimic the behavior of gain of function mutations in animal proto-oncogenes. Transformation occurs in non-permissive infection (vs. productive infection in permissive hosts) [Fig 28-4] 11 12 Transforming Viruses Carry Oncogenes Common mechanism of DNA tumor virus transformation Early genes with oncogenic potential Integration of viral oncogenes into host genomes Oncogene proteins always interact with host cellular proteins [Cell transformation by polyomavirus/adenovirus; Fig 28-5] Polyoma & SV40 produce T-antigens early in infection T-antigen has transforming activity Papillomaviruses produce E6 & E7 oncoproteins EBV (causes lymphoproliferative disorders) immortalized human B lymphocytes EBV oncogene unknown causes African Burkitt lymphoma Retroviruses transfer vertically & horizontally 13 14 Transforming Viruses Carry Oncogenes Common mechanism of DNA tumor virus transformation Retroviruses transfer vertically & horizontally [Fig 28-6] Integration of viral genome in germ line vertical transfer Reverse transcription needed for virus with RNA genome Types of retroviruses: Non-defective tumor retroviruses: ability to transform depends upon its effects on expression of host genes at its site of insertion into the cellular genome. Ex. Leukemia virus (FLV) No viral oncogene; viral activation of cellular proto-oncogene Acute transforming tumor retroviruses Captured new genes in the form of oncogene (cellular origin) [Cellular gene in transforming retroviruses; Fig 28-7] Rare event, cannot replicate by itself and need helper virus 15 16 17 Retroviral Oncogenes’ Cellular Counterpart Oncogenes of some retroviruses [Fig 28-8] The normal cellular sequence itself is not oncogenic Difference between oncogenes & proto-oncogenes: Changes in v-oncogenes Retrovirus capture of the proto-oncogene (c) oncogene (v) 30+ c-onc genes identified; Most existing oncogene been identified? Rare event, and can be complex; Non-random Direct evidence that v-oncogene accomplishes transformation: Rous Sarcoma Virus carries v-src gene Oncogenes arise by activation of cellular or proto-oncogene is important to animal cancer Human cancer too? 18 19 V-onc is responsible for transformation 20 Basics of Cancer Cancer is a genetic disease because it can be traced to alterations within specific genes, but in most cases, it is not an inherited disease. In an inherited disease, the genetic defect is present in the chromosomes of a parent and is transmitted to the zygote. In contrast, the genetic alterations that lead to most cancers arise in the DNA of a somatic cell during the lifetime of the affected individual. Because of these genetic changes, cancer cells proliferate uncontrollably, producing malignant tumors that invade surrounding healthy tissue (Figure 16.1). As long as the growth of the tumor remains localized, the disease can usually be treated and cured by surgical removal of the tumor. But malignant tumors tend to metastasize, that is, to spawn cells that break away from the parent mass, enter the lymphatic or vascular circulation, and spread to distant sites in the body where they establish lethal secondary tumors (metastases). Basics of cancer continued… Because of its impact on human health and the hope that a cure might be developed, cancer has been the focus of a massive research effort for decades. Though these studies have led to a remarkable breakthrough in our understanding of the cellular and molecular basis of cancer, they have very much impact on preventing cancers. The incidence of various types of cancer in the United States and the corresponding mortality rates are shown in Figure 16.2. Current treatments, such as chemotherapy and radiation, lack the specificity needed to kill cancer cells without simultaneously damaging normal cells, as evidenced by the serious side effects that accompany these treatments. As a result, patients cannot usually be subjected to high enough doses of chemicals or radiation to kill all of the tumor cells in their body. Researchers have been working for many years to develop more effective and less debilitating (impairing strength and vitality) treatments. Basic properties of cancerous cell The behaviour of cancer cells is most easily studied when the cells are growing in culture. Cancer cells can be obtained by removing a malignant tumor, dissociating the tissue into its separate cells, and culturing the cells in vitro. Over the years, many different lines of cultured cells that were originally derived from human tumors have been collected in cell banks and are available for study. Alternatively, normal cells can be converted to cancer cells by treatment with carcinogenic chemicals, radiation, or tumor viruses. Cells that have been transformed in vitro by chemicals or viruses can generally cause tumors when introduced into a host animal. There are many basic differences exist between normal cells and cancer cell. Basic properties of cancerous cell continued… At the cellular level, the most important characteristic of a cancer cell whether residing in the body or on a culture dish is its loss of growth control. When normal cells are grown in tissue culture under conditions that promote cell proliferation, they grow and divide at a rate similar to that of their malignant counterparts. However, when the normal cells proliferate to the point where they cover the bottom of the culture dish, their growth rate decreases markedly, and they tend to remain as a single layer (monolayer) of cells (Figure 16.3a,b). Growth rates drop as normal cells respond to inhibitory influences from their environment. Growth-inhibiting influences may arise as the result of depletion of growth factors in the culture medium or from contact with surrounding cells on the dish. In contrast, when malignant cells are cultured under the same conditions, they continue to grow, piling on top of one another to form clumps (Figure 16.3c,d ). It is evident that malignant cells are not responsive to the types of signals that cause their normal counterparts to cease growth and division. Not only do cancer cells ignore inhibitory growth signals, they continue to grow in the absence of stimulatory growth signals that are required by normal cells. Normal cells growing in culture depend on growth factors, such as epidermal growth factor and insulin, that are present in serum (the fluid fraction of blood), which is usually added to the growth medium (Fig-ure 16.4). Cancer cells can proliferate in the absence of serum because their cell cycle does not depend on the interaction between growth factors and their receptors, which are located at the cell surface. In the below figure, transformation is a result of basic changes in the intracellular pathways that govern cell proliferation and survival. Normal cells growing in culture exhibit a limited capacity for cell division; after a finite number of mitotic divisions, they undergo an aging process that renders them unfit to continue to grow and divide. Cancer cells, on the other hand, are seemingly immortal because they continue to divide indefinitely. This difference in growth potential is often attributed to the presence of telomerase in cancer cells and its absence in normal cells. Telomerase is the enzyme that maintains the telomeres at the ends of the chromosomes, thus allowing cells to continue to divide. The absence of telomerase from most types of normal cells is thought to be one of the body’s major defences that protects against tumor growth. Basic properties of cancerous cell continued… Normal cells maintain their diploid chromosomal complement as they grow and divide, both in vivo and in vitro. In contrast, cancer cells are genetically unstable and often have highly aberrant chromosome complements, a condition termed aneuploidy (Figure 16.5), which may occur primarily as a result of defects in the mitotic checkpoints. It is evident from Figure 16.5 that the growth of cancer cells is much less dependent on a standard diploid chromosome content than the growth of normal cells. In fact, when the chromo some content of a normal cell becomes disturbed, a signalling pathway is usually activated that leads to the self-destruction (apoptosis) of the cell. In contrast, cancer cells typically fail to elicit the apoptotic response even when their chromosome content becomes highly deranged. Protection from apoptosis is another important hallmark that distinguishes many cancer cells from normal cells. Basic properties of cancerous cell continued… Finally, it can be noted that cancer cells often depend on glycolysis, which is an anaerobic metabolic pathway. This property may reflect the high metabolic requirements of cancer cells and an inadequate blood supply within the tumor. Under conditions of hypoxia (reduced O2 ), cancer cells activate a transcription factor called HIF that induces the formation of new blood vessels and promotes the migratory properties of the cells, which may contribute to the spread of the tumor. However, even when oxygen is plentiful, tumor cells continue to generate much of their ATP by glycolysis. The end product of glycolysis is lactic acid, which is secreted into the tumor’s microenvironment, where it may promote tumor growth. The causes of cancer In 1775, Percivall Pott, a British surgeon, made the first known correlation between an environmental agent and the development of cancer. Pott concluded that the high incidence of cancer of the nasal cavity in ink- industry was due to their chronic exposure to soot. Within the past several decades, the carcinogenic chemicals in soot have been isolated, along with hundreds of other compounds shown to cause cancer in laboratory animals. In addition to a diverse array of chemicals, a number of other types of agents are also carcinogenic, including ionizing radiation and a variety of DNA and RNA containing viruses. All of these agents have one property in common: they alter the genome. Carcinogenic chemicals, such as those present in soot or cigarette smoke, can almost always be shown either to be directly mutagenic or to be converted to mutagenic compounds by cellular enzymes. Similarly, ultraviolet radiation, which is the leading cause of skin cancer, is also strongly mutagenic. The causes of cancer continued… A number of viruses can infect mammalian cells growing in cell culture, transforming them into cancer cells. These viruses are broadly divided into two large groups: DNA tumor viruses and RNA tumor viruses. Among the DNA viruses capable of transforming cells are polyoma virus, simian virus 40 (SV40), Human papilloma virus (E6 and E7) and adenovirus (E1A and E1B). Tumor viruses can transform cells because they carry genes whose products interfere with the cell’s normal growth regulating activities. Although tumor viruses were an invaluable tool for researchers in identifying numerous genes involved in cell transformation, they are associated with human cancers. The causes of cancer continued… This relationship between viral infection and cancer is illustrated by human papilloma virus (HPV), which can be transmitted through sexual activity and is increasing in frequency in the population. Although the virus is present in about 90 percent of cervical cancers, indicating its importance in development of the disease, the vast majority of women who have been infected with the virus will never develop this malignancy. An effective vaccine against this virus is now available. Other viruses linked to human cancers include hepatitis B virus, which is associated with liver cancer; Epstein Barr virus, which is associated with Burkitt’s lymphoma in areas where malaria is common. The causes of cancer continued… Certain gastric lymphomas are associated with chronic infection by the stomach-dwelling bacterium Helicobacter pylori, which is also responsible for ulcers. Recent evidence suggests that many of these cancers linked to infections are actually caused by the chronic inflammation that is triggered by the presence of the pathogen. Inflammatory bowel disease (IBD), which is also characterized by chronic inflammation, has been associated with an increased risk of colon cancer. These findings have caused researchers to look more closely at the general process of inflammation as a previously unexplored factor in the development of many types of cancers. Genetics of Cancer Cancer is one of the leading causes of death in Western countries, affecting approximately one in every three individuals. Whenever the cells of a cancerous tumor are genetically scrutinized, they are invariably found to have arisen from a single cell. Thus, unlike other diseases that require modification of a large number of cells, cancer results from the uncontrolled proliferation of a single wayward (resistant to guidance and discipline) cell (cancer is said to be monoclonal). Consider for a moment that the human body contains trillions of cells, billions of which undergo cell division on any given day. Though almost any one of these dividing cells may have the potential to change in genetic composition and grow into a malignant tumor, this only occurs in about one-third of the human population during an entire lifetime. Genetics of cancer continued… One of the primary reasons why a greater number of cells do not give rise to cancerous tumors is that malignant transformation requires more than a single genetic alteration. We can distinguish between two types of genetic alterations that might make us more likely to develop a particular type of cancer—those that we inherit from our parents (germ-line mutations) and those that occur during our own lifetime (somatic mutations). Tumor suppressor genes loss-of-function mutation cause cancer Tumor-suppressor genes act as a cell’s brakes; they encode proteins that restrain cell growth and prevent cells from becoming malignant (See figure). The existence of such genes originally came to light from studies in the late 1960s in which normal and malignant rodent cells were fused to one another. Some of the cell hybrids formed from this type of fusion lost their malignant characteristics, suggesting that a normal cell possesses factors that can suppress the uncontrolled growth of a cancer cell. Further evidence for the existence of tumor- suppressor genes was gathered from observations that specific regions of particular chromosomes are consistently deleted in cells of certain types of cancer. If the absence of such genes is correlated with the development of a tumor, then it follows that the presence of these genes normally suppresses the formation of the tumor. Proto-oncogenes gain-of- function arise oncogene that causes cancer Oncogenes, on the other hand, encode proteins that promote the loss of growth control and the conversion of a cell to a malignant state (see figure). Oncogenes may lead to genetic instability, prevent a cell from becoming a victim of apoptosis, or promote metastasis. Tumor viruses transform a normal cell into a malignant cell because they carry an oncogene that encodes a protein that interferes with the cell’s normal activities. The turning point in these studies came in 1976, when it was discovered that an oncogene called src, carried by an RNA tumor virus called avian sarcoma virus, was actually present in the genome of uninfected cells. The oncogene, in fact, was not a viral gene, but a cellular gene that had become incorporated into the viral genome during a previous infection. It soon became evident that cells possess a variety of genes, now referred to as protooncogenes, that have the potential to subvert (destroy) the cell’s own activities and push the cell toward the malignant state. Protooncogene: cellular normal gene that causes cancer under altered condition(s) Tumor suppressor genes The transformation of a normal cell to a cancer cell is accompanied by the loss of function of one or more tumor-suppressor genes. At the present time, more than two dozen genes have been implicated as tumor suppressors in humans, some of which are listed in Table Included among the genes on the list are those that encode transcription factors (e.g., TP53), cell cycle regulators (e.g., RB and p16), and a protein that regulates protein degradation (VHL). In one way or another, most of the proteins encoded by tumor-suppressor genes act as negative regulators of cell proliferation, which is why their elimination promotes uncontrolled cell growth. Gene Tumor Function TP53 Lymphomas, sarcoma Transcription factor (cell cycle and Ref. Adapted from Karp apoptosis) cell and Molecular Biology 7th edition RB Retinal Binds E2F (cell cycle regulation) VHL Renal Protein degradation p16 Pancreatic Cdk inhibitor Tumor suppressor gene: RB It is known that members of some families are at high risk of developing certain types of cancers. Although these inherited cancer syndromes are rare, they provide an unprecedented opportunity to identify tumor suppressor genes that, when missing, contribute to the development of both inherited and sporadic (i.e., noninherited) forms of cancer. The first tumor-suppressor gene to be studied and eventually cloned and one of the most important is associated with a rare childhood cancer of the retina of the eye, called retinoblastoma. The gene responsible for this disorder is named RB. The incidence of retinoblastoma follows two distinct patterns: (1) it occurs at high frequency and at young age in members of certain families, and (2) it occurs sporadically at an older age among members of the population at large (freely). The fact that retinoblastoma runs in certain families suggested that the cancer can be inherited. Examination of cells from children suffering from retinoblastoma revealed that one member of the thirteenth pair of homologous chromosomes was missing a small piece from the interior portion of the chromosome. The deletion was present in all of the children’s cells both the cells of the retinal cancer and cells elsewhere in the body—indicating that the chromosomal aberration had been inherited from one of the parents. Retinoblastoma: Retina tumor Loss of RB: Osteosarcoma and lung cancer. 40 RB protein continued… Retinoblastoma is inherited as a dominant genetic trait because members of high-risk families that develop the disease inherit one normal allele and one abnormal allele. In fact, approximately 10 percent of individuals who inherit a chromosome with an RB deletion never develop the retinal cancer. How is it that a small percentage of these predisposed individuals escape the disease? RB protein continued… Although deficiencies in the RB gene are first manifested in the development of retinal cancers, this is not the end of the story. People who suffer from the inherited form of retinoblastoma are also at high risk of developing other types of tumors later in life, particularly soft-tissue sarcomas (tumors of mesenchymal rather than epithelial origin). Mutations in RB alleles are a common occurrence in retinobalstoma, sporadic prostate, osteo and lung cancers. When cells from these tumors are cultured in vitro, the reintroduction of a wild-type RB gene back into the cells is sufficient to suppress their cancerous phenotype, indicating that the loss of this gene function contributes significantly to tumorigenesis. The Role of pRB in Regulating the Cell Cycle It is well established fact that control the cell cycle can play a pivotal role in the development of cancer. In its best studied role, the protein encoded by the RB gene, pRB, helps regulate the passage of cells from the G1 stage of the cell cycle into S phase, during which DNA synthesis occurs. The transition from G 1 to S is a time of commitment for the cell; once a cell enters S phase, it invariably proceeds through the remainder of the cell cycle and into mitosis. The Role of pRB in Regulating the Cell Cycle continued… RB protein continued… The importance of pRB as a negative regulator of the cell cycle is demonstrated by the fact that DNA tumor viruses (including adenoviruses, human papilloma virus, and SV40) encode a protein that binds to pRB, blocking its ability to bind to E2F. The ability of these viruses to induce cancer in infected cells depends on their ability to block the negative influence that pRB has on progression of a cell through the cell cycle. By using these pRB-blocking proteins, these viruses accomplish the same result as when the RB gene is deleted, leading to the development of human tumors. p53 suppresses growth or triggers apoptosis Tumor suppressor gene >50% of cancers lost p53 or have mutations in p53 gene p53 protein level in many tumor cells; Oncogene? Mutant protein acted as dominant negative mutants tetramer Loss of p53: Cell growth advantage; not tissue-specific (many cancers) [Wild type p53 restrains cell growth; Fig 8-24] Implication: p53 inhibits normal cells’ capacity of unrestrained growth? Evidence that p53 is indeed a tumor suppressor gene: p53-/- mice develop a variety of tumors early in life p53 inhibits transformation by oncogenes in cultured cells 46 47 P53 Suppresses Growth or Triggers Apoptosis p53 protects cells from consequences of DNA damages p53 (repair it or destroy if it is unable to repair!) Activation of p53 growth arrest or apoptosis [Fig 28-25] Depends on cell cycle Other molecular activities of p53 [Fig 28-26] p53 can also activate various pathways [Fig 28-27] as a transcription factor How p53 trigger apoptosis? Separable from growth arrest Is p53 function essential for survival? 48 p53 presents in low concentration in normal cells. DNA damage activates the p53. Amount of p53 increases. Two types of events triggered: 1. Growth arrest (G1) and 2. apoptosis (S-G2). 49 Protein structure of p53 p53 is a DNA binding protein recognizes 10 bp palindromic sequences N-teriminal is for activate transcription Has ability to bind damaged DNA region C-terminal region recognizes the ssDNA region p53 is a tetramer (oligomerzation is needed before become functional) TBP:The TATA-binding protein (TBP) is a general transcription factor that binds specifically to a DNA sequence called the TATA box. Mdm2:Mdm2 is an important negative regulator of the p53 tumor suppressor. E1B55: adenovirus oncoprotein, E1B lysis produces 55 and 18 kd products. The E1B 55kDa protein inhibits p53 acetylation, and therefore activation. P300/CBP: Coactivator, p300 (also called EP300 or E1A binding protein p300) CBP (also known as CREB-binding protein or CREBBP) 50 p21: is a CKI (cell cycle inhibitor, involved in arresting cell in G1 phase) GADD45:(growth arrest and DNA- damage inducible) DNA repair gene, helps cell to maintain genome stability. 51 P53 continued… The TP53 gene codes for p53 protein. 53 Kda protein. The tumor-suppressor gene that, when absent, is responsible for a rare inherited disorder called Li-Fraumeni syndrome. Victims of this disease are affected with a very high incidence of various cancers, including breast and brain cancer and leukemia. Like individuals with the inherited form of retinoblastoma, persons with Li-Fraumeni syndrome inherit one normal and one abnormal (or deleted) allele of the TP53 tumor- suppressor gene and are thus highly susceptible to cancers that result from random mutations in the normal allele. p53 continued… Point mutations or deletions in both alleles of the TP53 gene leads to cancer progression. Furthermore, tumors composed of cells bearing TP53 mutations are correlated with a poorer survival rate than those containing a wild-type TP53 gene. Clearly, the elimination of TP53 function is an important step in the progression of many cancer cells toward the fully malignant state. p53 continued… Why is the presence of p53 so important in preventing a cell from becoming malignant? p53 is a transcription factor that activates the expression of a large number of genes involved in cell cycle regulation and apoptosis. The importance of the transcription-regulating role of p53 is evident in Figure 16.12, which shows the location of the six mutations most commonly found to disable p53 in human cancers; all of them map in the region of the protein that interacts with DNA. p53 protein function p53 continued… One of the best studied genes activated by p53 is p21 that inhibits the cyclin-dependent kinase that normally drives a cell through the G1 checkpoint. As the level of p53 rises in the damaged G1 cell, expression of the p21 gene is activated, and progression through the cell cycle is arrested. This gives the cell time to repair the genetic damage before it initiates DNA replication. When both copies of the TP53 gene in a cell have been mutated so that their product is no longer functional, the cell can no longer produce the p21 inhibitor or exercise the feedback control that prevents it from entering S phase when it is not prepared to do so. Failure to repair DNA damage leads to the production of abnormal cells that have the potential to become malignant. p53 and apoptosis Cell cycle arrest is not the only way that p53 protects an organism from developing cancer. Alternatively, p53 can direct a genetically damaged cell along a pathway that leads to death by apoptosis, thereby ridding the body of cells with a malignant potential. Whether p53 moves a cell toward cell cycle arrest or apoptosis apparently depends on the type of posttranslational modifications to which it is subjected. The p53 protein is thought to direct a cell into apoptosis as the result of several events, including the activation of expression of the BAX gene, whose encoded product (Bax) initiates apoptosis. Bcl2 associated X protein (BAX) belongs to BCL2 family. BCL2 family members form homo or heterodimers and act as anti- or pro- apoptotic regulators that are involved in a wide variety of cellular activities. This protein forms a heterodimer with BCL2, and functions as an apoptotic activator. p53 and apoptosis continued… This protein is reported to interact with, and increase the opening of, the mitochondrial voltage-dependent anion channel (VDAC), which leads to the loss in membrane potential and the release of cytochrome c. The expression of this gene is regulated by the tumor suppressor p53 and has been shown to be involved in p53- mediated apoptosis. If both alleles of TP53 should become inactivated, a cell that is carrying damaged DNA fails to be destroyed, even though it lacks the genetic integrity required for controlled growth. Several studies have shown that established tumors in mice will undergo regression when the activity of their p53 genes is restored. p53 and apoptosis continued… The level of p53 in a healthy G1 cell is very low, which keeps its potentially lethal action under control. However, if a G1 cell sustains genetic damage, as occurs if the cell is subjected to ultraviolet light or chemical carcinogens, the concentration of p53 rises rapidly. A similar response can be elicited simply by injecting a cell with DNA containing broken strands. The increase in p53 levels is not due to increased expression of the gene but to an increase in the stability of the protein. In unstressed cells, p53 has a half-life of a few minutes. p53 degradation is facilitated by a protein called MDM2, which binds to p53 and escorts it out of the nucleus and into the cytosol. Once in the cytosol, MDM2 adds ubiquitin molecules to the p53 molecule, leading to its destruction by a proteasome. p53 and cell cycle How does DNA damage lead to stabilization of p53? Persons suffering from ataxia telangiectasia lack a protein kinase called ATM and are unable to respond properly to DNA-damaging radiation. ATM is normally activated following DNA damage, and Chk2 (Check point kinase 2) is one of the proteins ATM phosphorylates then chk2 phosphorylates p53 protein. The phosphorylated version of the p53 molecule is no longer able to interact with MDM2, which stabilizes existing p53 molecules in the nucleus and allows them to activate the expression of genes such as p21 and BAX. Some tumor cells have been found that contain a wildtype TP53 gene but extra copies of MDM2. Such cells are thought to produce excessive amounts of MDM2, which prevents p53 from building to required levels to stop the cell cycle or induce apoptosis following DNA damage (or other oncogenic stimuli). p53 and cell cycle continued… A major effort is underway to develop drugs that block the interaction between MDM2 and p53 in an attempt to restore p53 activity in cancer cells that retain this key tumor suppressor. The relationship between MDM2 and p53 has also been demonstrated using gene knockouts mice that lack a gene encoding MDM2 die at an early stage of development, presumably because their cells undergo p53-dependent apoptosis. This interpretation is supported by the finding that mice lacking genes that encode both MDM2 and p53 (double knockouts) survive to adulthood but are highly prone to cancer. Because these embryos cannot produce p53, they don’t require a protein such as MDM2 that facilitates p53 destruction. This observation illustrates an important principle in cancer genetics: even if a “crucial” gene such as RB or TP53 is not mutated or deleted, the function of that gene can be affected as the result of alterations in other genes whose products are part of the same pathway as the “crucial” gene. In this case, overexpression of MDM2 can have the same effect as the absence of p53. As long as the tumor suppressor pathway is blocked, the tumor-suppressor gene it self need not be mutated. Numerous studies indicate that both the p53 and pRB pathways have to be inactivated, one way or another, to allow the progression of most tumor cells. p53 and cell cycle continued… Because of its ability to trigger apoptosis, p53 plays a pivotal role in treatment of cancer by radiation and chemotherapy. It was assumed for many years that cancer cells are more susceptible than normal cells to drugs and radiation because cancer cells divide more rapidly. Theory suggests that normal cells are more resistant to drugs or radiation because, once they sustain genetic damage, they either arrest their cell cycle until the damage is repaired or they undergo apoptosis. In contrast, cancer cells that have sustained DNA damage are more likely to become apoptotic—as long as they possess a functioning TP53 gene. If cancer cells lose p53 function, they often cannot be directed into apoptosis and they become highly resistant to further treatment (Figure 16.14). Familial adenomatous polyposis coli (FAP) (FAP) is an inherited disorder in which individuals develop hundreds or even thousands of premalignant polyps (small vascular growth on the surface of a mucous membrane) (adenomas; a benign epithelial tumor of glandular origin) from epithelial cells that line the colon wall (Figure 16.15). If not removed, cells within some of these polyps are very likely to progress to a fully malignant stage. The cells of patients with this condition were found to contain a deletion of a small portion of chromosome 5, which was subsequently identified as the site of a tumor-suppressor gene called APC (Adenomatous polyposis coli). A person inheriting an APC deletion is in a similar position to one who inherits an RB deletion. FAP continued… The loss of the second allele of APC causes the cell to lose growth control and proliferate to form a polyp rather than differentiating into normal epithelial cells of the intestinal wall. The conversion of cells in a polyp to the more malignant state, characterized by the ability to metastasize and invade other tissues, is presumably gained by the accumulation of additional mutations, including those in TP53. Mutated APC genes are found not only in inherited forms of colon cancers, but also in up to 80 percent of sporadic colon tumors, suggesting that the gene plays a major role in the development of this disease. The protein encoded by the APC gene binds a number of different proteins, and its mechanism of action is complex. In its best studied role, APC suppresses the transcription of genes CCND1 that promote cell proliferation. APC may also play a role in the attachment of microtubules to the kinetochores of mitotic chromosomes. Loss of APC function could therefore lead directly to abnormal chromosome segregation and aneuploidy. The presence of mutated APC DNA has been found in the blood of persons with early-stage colon cancer, which raises the possibility of a diagnostic test for the disease. BRCA1 & 2 It is estimated that breast cancer strikes approximately one in eight women living in the United States, Canada, and Europe. Of these cases, 5–10 percent are due to the inheritance of a gene that predisposes the individual to development of the disease. After an intensive effort by several laboratories, two genes named BRCA1 and BRCA2 were identified in the mid-1990s as being responsible for the majority of the inherited cases of breast cancer. BRCA mutations also predispose a woman to the development of ovarian cancer, which has an especially high mortality rate. BRCA1 & 2 continued… BRCA1 and BRCA2 proteins have been the focus of a major research effort, but their precise functions remain unclear. It was known that cells possess check points that halt progression of the cell cycle following DNA damage. The BRCA proteins are part of one or more large protein complexes that respond to DNA damage and activate DNA repair by means of homologous recombination. Cells with mutant BRCA proteins contain unrepaired DNA and exhibit a highly aneuploid karyotype. BRCA1 & 2 continued… In cells with a functional TP53 gene, failure to repair DNA damage leads to the activation of p53, which causes the cell to either arrest cell cycle progress or undergo apoptosis, as illustrated in Figure 16.16. Unlike most tumor-suppressor genes, neither of the BRCA genes is commonly mutated in sporadic forms of the cancer, although BRCA1 is often epigenetically silenced. Oncogenes Oncogenes encode proteins that promote the loss of growth control and the conversion of a cell to a malignant state. Oncogenes are derived from proto-oncogenes, which are genes that encode proteins having a function in the normal cell. Most known proto-oncogenes play a role in the control of cell growth and division. The oncogene mutated most frequently in human tumors is RAS, which encodes a GTP- binding protein (Ras) that functions as an on–off switch for a number of key signaling pathways controlling cell proliferation. Oncogenic RAS mutants typically encode a protein whose GTPase activity cannot be stimulated, which leaves the molecule in an active GTP-bound form, sending continuous proliferation signals along the pathway. The functions of a number of oncogenes are summarized in next slide Figure 16.17. Oncogenes continued…. Oncogenes that Encode Growth Factors or their receptors. The first connection between oncogenes and growth factors was made in 1983, when it was discovered that the cancer-causing simian sarcoma virus contained an oncogene (sis) derived from the cellular gene for platelet-derived growth factor (PDGF), a protein present in human blood. Cultured cells that are transformed with this virus secrete large amounts of PDGF into the medium, which causes the cells to proliferate in an uncontrolled fashion. Overexpression of PDGF has been implicated in the development of brain tumors (gliomas). Oncogenes continued…. Another oncogenic virus, avian erythroblastosis virus, was found to carry an oncogene (erbB) that encodes an EGF receptor that is missing part of the extracellular domain of the protein that binds the growth factor. One might expect that the altered receptor would be unable to signal a cell to divide, but just the reverse is true. This altered version of the receptor stimulates the cell constitutively, that is, regardless of whether or not the growth factor is present in the medium. This is the reason why cultured cells that carry the altered gene proliferate in an uncontrolled manner. A oncogenic receptor constitutively activates the pathway v-erb is truncated version of c-erbB (codes EGF receptor) retains kinase and transmembrane domain. Mutant receptor lacks N- terminal ligand binding site and also C-terminus. Both ends deletion are needed for Oncogenecity. Oncogenes continued…. A number of spontaneous human cancers have been found to contain cells with genetic alterations that affect growth factor receptors, including EGFR. Most commonly, the malignant cells contain a much larger number of the receptors in their plasma membranes than do normal cells. The presence of excess receptors makes the cells sensitive to much lower concentrations of the growth factor, and thus, they are stimulated to divide under conditions that would not affect normal cells. SRC and Myc oncogene The first oncogene to be discovered, SRC, is also a protein kinase, but one that phosphorylates tyrosine residues on protein substrates rather than serine and threonine residues. Transformation of a cell by a src-containing tumor virus is accompanied by the phosphorylation of a wide variety of proteins. Included among the apparent substrates of Src are proteins involved in signal transduction, control of the cytoskeleton, and cell adhesion. Myc oncogene Oncogenes that encode nuclear transcription factors a number of oncogenes encode proteins that act as transcription factors. The progression of cells through the cell cycle requires the timely activation (and repression) of a large variety of genes whose products contribute in various ways to cell growth and division. It is not surprising, therefore, that alterations in the proteins that control the expression of these genes could seriously disturb a cell’s normal growth patterns. Probably the best studied oncogene whose product acts as a transcription factor is MYC. SRC & Myc oncogene continued… Cells that are not actively growing and dividing tend to withdraw from the cell cycle and enter a stage referred to as G0 , from which they can be retrieved. Myc is normally one of the first proteins to appear when a cell in this quiescent stage has been stimulated by growth factors to re-enter the cell cycle and divide. Myc regulates the expression of a huge number of proteins and miRNAs involved in cell growth and proliferation. When MYC expression is selectively blocked, the progression of the cell through G1 is blocked. The MYC gene is one of the proto-oncogenes most commonly altered in human cancers, often being amplified within the genome or rearranged as the result of a chromosome translocation. These chromosomal changes are thought to remove the MYC gene from its normal regulatory influences and increase its level of expression in the cell, producing an excess of the Myc protein. Myc oncogene continued… One of the most common types of cancer among populations in Africa, called Burkitt’s lymphoma, results from the translocation of a MYC gene to a position adjacent to an antibody gene. The disease occurs primarily in persons who have also been infected with Epstein-Barr virus. This same virus causes only minor infections (e.g., mononucleosis) in people living in Western countries and is not associated with tumorigenesis. Oncogenes product affect apoptosis Apoptosis is one of the body’s key mechanisms to rid itself of tumor cells at an early stage in their progression toward malignancy. Consequently, any alteration that diminishes a cell’s ability to self- destruct would be expected to increase the likelihood of that cell giving rise to a tumor. PI3K/PKB pathway is involved in cell survival and tumorigenesis. With this in mind, it is not surprising that both PI3K and PKB are encoded by documented oncogenes. The oncogene most closely linked to apoptosis is BCL-2, which encodes a membrane-bound protein that inhibits apoptosis. The role of BCL-2 in apoptosis is most clearly revealed in the phenotypes of knockout mice that are lacking a Bcl-2 gene. Oncogenes product affect apoptosis continued… Once formed, the lymphoid tissues of these mice undergo dramatic regression as the result of wide spread apoptosis. Like MYC, the product of the BCL-2 gene becomes oncogenic when it is expressed at higher than normal levels, as can occur when the gene is translocated to an abnormal site on the chromosome. Certain human lymphoid cancers (called follicular B-cell lymphomas) are correlated with the translocation of the BCL-2 gene next to a gene that codes for the heavy chain of antibody molecules. It is suggested that overexpression of the BCL-2 gene leads to the suppression of apoptosis in lymphoid tissues, allowing abnormal cells to proliferate to form lymphoid tumors. The BCL-2 gene may also play a role in reducing the effectiveness of chemotherapy by keeping tumor cells alive and proliferating despite damage by the drug treatment. To counteract this property of cancer cells, a number of pharmaceutical companies are developing compounds that make cancer cells more likely to undergo apoptosis. PTEN: (phosphatidylinositol-3,4,5- trisphosphate 3-phosphatase ) DNA Repair and Oncogenes DNA mutation(s) is likely to increase the risk of developing cancer. Chemically altered or nucleotides that are incorporated incorrectly during replication are selectively removed from the DNA strand by DNA repair. DNA repair processes require the cooperative efforts of a substantial number of proteins, including proteins that recognize the lesion, remove a portion of the strand containing the lesion, and replace the missing segment with complementary nucleotides. If any of these proteins are defective, the affected cell can be expected to display an abnormally high mutation rate, which is described as a “mutator phenotype.” Cells with a mutator phenotype are likely to cause mutations in both tumor-suppressor genes and proto-oncogenes, which increases their risk of becoming malignant. DNA Repair and Oncogenes continued.. Defects in nucleotide excision repair (NER) lead to the development of a cancer syndrome called xeroderma pigmentosum. In 1993, evidence was obtained that defects in a different type of DNA repair might also lead to the initiation of cancer. In this case, studies were performed on the cells of patients with the most common hereditary form of colon cancer, called hereditary nonpolyposis colon cancer (HNPCC). A defective gene responsible for HNPCC is carried by approximately 0.5 percent of the population and accounts for about 3 percent of all colon cancer cases. It was known that the genome contains large numbers of very short, repetitive DNA sequences called microsatellites. Analysis of the DNA of persons with HNPCC revealed that microsatellite sequences in the tumor cells were often of different length than the corresponding sequences in the DNA of normal cells from the same patient. One would expect such variations in the DNA from different individuals, but not from the DNA of different cells of the same person. DNA Repair and Oncogenes continued.. The discovery of SNP in microsatellite sequence leads to generation of cancers like colon cancer has been reported. Support for this proposal has come from studies of persons with HNPCC. Whereas extracts from normal cells carry out mismatch repair in vitro, extracts from HNPCC tumor cells display DNA-repair deficiencies. Analysis of the DNA from a large number of people with HNPCC has revealed deletion mutations in any one of a number of genes that code for defective proteins that form the DNA mismatch repair pathway. Cells with mismatch repair deficiencies accumulate secondary mutations throughout the genome. Diseases in which DNA repair is failed Xeroderma pigmentosum (XP): Patients are hypersensitive to UV light; patients often develop malignancies of the skin. Ataxia telangiectasia (AT): Patients are sensitive to gamma irradiation; patients develop neurological and skin lesions. Xeroderma Pigmentosum (XP) and DNA Repair Defects XP is an autosomal recessive disease associated with dry skin, freckling, and skin tumors Many patients die before age 30 from metastasis of malignant skin tumors One form of XP is produced by a defect in the human endonuclease that removes pyrimidine dimers Mutations in genes involved in repairing UV-damaged DNA can cause XP Xeroderma Pigmentosum (XP) Symptoms include: Extreme sensitivity to sunlight Early onset of skin cancer Why are XP patients sensitive to sunlight? UV NER NO REPAIR Healthy Healthy Damaged DNA DNA DNA Micro RNA and cancer microRNAs are tiny regulatory RNAs that negatively regulate the expression of target mRNAs. Given that cancers arise as the result of abnormal gene expression, it would not be surprising to discover that miRNAs are somehow involved in tumorigenesis. In 2002, it was reported that two microRNAs, miR-15a and miR-16 were either deleted or under expressed in most cases of chronic lymphocytic leukemia. It was subsequently shown that these two miRNAs act to inhibit expression of the mRNA that encodes the antiapoptotic protein Bcl-2, a known proto- oncogene. In the absence of the miRNAs, the oncogenic Bcl-2 protein is overexpressed, which promotes development of leukemia. Because these miRNAs act to inhibit tumorigenesis, they can be thought of as tumor suppressors. When the leukemic cells lacking miR- 15a and miR-16 were genetically engineered to re-express these RNAs, they underwent apoptosis. The locus that encodes miR-15a and miR-16 is also deleted in other types of cancers, suggesting it has widespread importance in tumor suppression. Micro RNA and cancer continued… The expression of two of the most important human oncogenes, RAS and MYC, have also been shown to be inhibited by an miRNA, namely, let-7, which was the first miRNA to be discovered. Some miRNAs act more like oncogenes than tumor suppressors. One specific cluster of miRNA genes, for example, is overexpressed during the formation of certain lymphomas. Overexpression of these miRNAs can occur because the gene cluster encoding them is present in increased number (amplified) in the tumor cells, or it can occur because the gene cluster is excessively transcribed as the result of an overly active transcription factor. The abnormal expression of miRNAs has also been implicated as a causal factor in tumor cell invasiveness and metastasis, which elevates the interest in these RNAs to an even higher level. Micro RNA and cancer continued… It is not yet clear how important miRNAs are in the overall occurrence of human cancer, but a number of microarray studies that survey large numbers of these tiny regulatory RNAs suggest that most human cancers have a characteristic miRNA expression profile, just as they have a characteristic mRNA expression profile. Recent studies suggest that miRNA expression profiles may serve as sensitive and accurate biomarkers to identify the exact type of tumor a person is suffering from and the best avenue of treatment. These studies also suggest that miRNAs such as let-7 may ultimately serve as another weapon in the arsenal of anticancer therapeutics. Insulin-like growth factor 1 receptor The insulin-like growth factor 1 (IGF-1) receptor is a protein found on the surface of human cells. It is a transmembrane receptor that is activated by a hormone called insulin-like growth factor 1 (IGF-1) and by a related hormone called IGF-2. It belongs to the large class of tyrosine kinase receptors. This receptor mediates the effects of IGF-1, which is a polypeptide protein hormone similar in molecular structure to insulin. IGF-1 plays an important role in cell growth. Mice lacking the IGF-1 receptor die during development. Structure of IGF-1R Two alpha subunits and two beta subunits make up the IGF-1 receptor. Both the α and β subunits are synthesized from a single mRNA precursor. The α chains are located extracellularly, while the β subunit spans the membrane and is responsible for intracellular signal transduction upon ligand stimulation. The mature IGF-1R has a molecular weight of approximately 320 kDa. The receptor is a member of a family which consists of the insulin receptor and the IGF-2R (and their respective https://en.wikipedia.org/wiki/Insulin-like_growth_factor_1_receptor ligands IGF-1 and IGF-2), along with several IGF-binding proteins. Role in cancer The IGF-1R is implicated in several cancers, including breast, prostate, and lung cancers. In some instances its anti-apoptotic properties allow cancerous cells to resist the cytotoxic properties of chemotherapeutic drugs or radiotherapy. Increased levels of the IGF-IR are expressed in the majority of primary and metastatic prostate cancer patient tumors. Evidence suggests that IGF-IR signalling is required for survival and growth when prostate cancer cells progression. Members of the IGF receptor family and their ligands also seem to be involved in the carcinogenesis of Breast cancer. IGF1R is amplified in several cancer types based on analysis of TCGA data, and gene amplification could be one mechanism for overexpression of IGF1R in cancer. ATM and ATR Ataxia –Telangiectasia (AT) is an inherited recessive disorder. Characterized by a host of diverse symptom including greatly prone to the different types of cancer. Other symptom includes degeneration of nerve cells, unsteady posture (ataxia), permanently dilated blood vessels (telangiectasia), prone to infection and abnormal no. of chromosome. ATM and ATR continued… ATM gene codes for kinase, which involve in DNA repair. AT patients are extremely sensitive to ionizing radiations and DNA damaging agents. Damaged DNA of normal Cell stop the progress through the cell cycle till the damaged DNA repaired. For example, if a normal cell is irradiated during G1 phase of the cell cycle, it delays progression into S phase. ATM and ATR continued… Check points of cell cycle was discovered by Leland Hartwell and Ted Weinert in 1988 in yeast system. Check points are surveillance mechanism that halt the progress of the CC if 1. any chr. Damaged, or 2. certain critical processes, such as DNA replication during S-phase or chromosome alignment during M-phase, have not been properly completed. ATM and ATR continued… Check points are activated via sensors when cell’s DNA damaged. If a sensor detects defects in DNA, it triggers a response that halt CC progress further. Meanwhile cell use delay to repair Damaged DNA rather than continuing to next stage. This is important because mammalian cells that undergo with genetic defect run the risk of becoming transformed into cancerous cell. If, the DNA damage is beyond repair then check points transmit a signal that leads to cell death (apoptosis). ATM and ATR continued… ATM gene encodes for kinase, is responsible for AT disease , when this gene mutated. ATM kinase activated when DNA gets damaged especially double/single stranded DNA breaks (sensor). Activation of ATM after DNA damage causes CC arrest. A related kinase called ATR is activated, when of DNA is damaged by UV radiation. Both ATM and ATR are part of multiprotein complexes capable of binding to chromatin that contains damaged DNA. Once bound, ATM and ATR can phosphorylate variety of proteins that participate in CC arrest and DNA repairs. Mechanism of action of ATM-ATR The MRN complex is a protein complex consisting of Mre11, Rad50 and Nbs1 (also known as Nibrin). Double-strand break repair protein MRE11A is a protein that in humans is encoded by the MRE11A gene DNA repair protein RAD50 is a protein that in humans is encoded by the RAD50 gene involved in double strand DNA break repair. Nibrin, also known as Nbs1, is a protein which in humans is encoded by Reference: Karp Cell biology the NBN gene, associated with the repair of double strand breaks (DSBs) which pose serious damage to a genome.