Basic Electrical Concepts PDF
Document Details
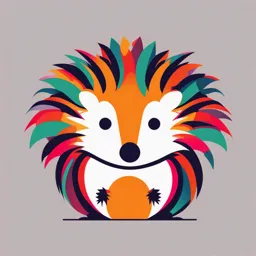
Uploaded by HeavenlySard99
Université Mustapha Stambouli
Mme K. Djelid
Tags
Summary
This document provides an overview of basic electrical concepts, including voltage, current, and resistance. It is geared toward students in fundamental electronics and electrical engineering introductory courses.
Full Transcript
People’s Democratic Republic of Algeria Ministry of Higher Education And Scientific Research Mustapha Stambouli University, Mascara-Algeria Faculty of Exact Sciences 1st year engineer BASIC ELECTRICAL CONCEPTS...
People’s Democratic Republic of Algeria Ministry of Higher Education And Scientific Research Mustapha Stambouli University, Mascara-Algeria Faculty of Exact Sciences 1st year engineer BASIC ELECTRICAL CONCEPTS Mme K. Djelid An electric current is a flow of electric charge in a circuit - the flow of free electrons between two points in a conductor. These free electrons in motion is what constitutes electrical energy. Electricity production consists of forcing electrons to move together in a conducting material by creating an electron deficit on one side of the conductor, and a surplus on the other. The device that produces this imbalance is called a generator. The terminal on the surplus side is marked +, that on the deficit side –. 2 When a charge is connected to the generator’s terminals, the generator pushes electrons: it absorbs the positive charged particles and sends back the negatively charged particles. In a circuit, the electrons circulate from the – terminal to the + terminal. To be able to use electrical equipment voltage properly and safely it is important to understand how electricity works. It is vital to understand the three basic building blocks current resistance required to manipulate and utilise electricity. 3 Electricity is the movement of electrons. Electrons create charge, which is used to generate energy. Every electrical device -a phone, a refrigerator - uses the motion of electrons to operate. voltage, current and resistance are described as Voltage Current Resistance The difference in charge The rate at which any given A material’s tendency to resist between two points. charge is flowing. the flow of charge (current). These values describe the movement of charge, and thus, the behaviour of electrons. A circuit is a closed loop that allows charge to move from one place to another. Components in the circuit 4 allows to control this charge and use it to do work. Voltage (U) is defined as the amount of potential energy between two points on a circuit. This difference in charge between the + and – poles in a generator is measured in volts and is represented with the letter “V". Sometimes voltage can be called “electric pressure,” an appropriate analogy because the force provided by electric potential difference to electrons passing through a conductive material can be compared to water pressure as water moves through a pipe; the higher the volts, the greater the “water pressure”. The available energy of the free electrons in motion is what constitutes electrical energy. Electricity production consists of forcing the electrons to move together through a conducting material by creating an electron deficit on one side of the conductor, and a surplus on the other. The terminal on the surplus side is marked (+), that on the deficit side (–). 5 An Electrical Current (I) is the flow of free electrons between two points in a conductor. As electrons move, an amount of charge moves with them; this is called current. The number of electrons that are able to move through a given substance is governed by the physical properties of the substance itself conducting the electricity - some materials allow current to move better than others. Electrical current (I) is expressed and measured in Amperes (A) as a base unit of electrical current. If volts (V) can be compared to the water pressure of water passing through a pipe, amperes (A) can be compared to the overall volume of water capable of flowing through the pipe at any given moment. 6 The motion of the free electrons is normally random, resulting no overall movement of charge. If a force acts on the electrons to move them in a particular direction, then they will all drift in the same direction. No Potential Difference Applied Potential Difference Diagram: Free electrons in a conductive material with and without current applied 7 The resistance of an object is the tendency of this object to oppose to the flow of electric current. In terms of electricity, the resistance of a conductive material is a measure how the device or material reduces the electric current flowing through it. Every material has some degree of resistance; it can be very low – such as copper (1-2 ohm per 1 meter) – or very high – such as wood (10000000 ohm per 1 meter). As an analogy to water flowing through a pipe, resistance is bigger when the pipe is narrower, decreasing the flow of water. In two circuits with equal voltages and different resistances, the circuit with the higher resistance will allow less charge to flow, meaning the circuit with higher resistance has less current flowing through it. 8 The Resistance (R) is expressed in ohms. Ohm defines the unit of resistance of “1 ohm” as the resistance between two points in a conductor where the application of 1 volt will push 1 ampere. This value is usually represented in schematics with the Greek letter “Ω”, which is called omega, and pronounced “ohm”. For a given voltage, the current is proportional to the resistance. This proportionality, expressed as a mathematical relationship, is known as Ohm’s Law: U=I×R Voltage = Current × Resistance Ohm’s Law is valid only for pure resistance, i.e., for devices that convert electrical energy into purely thermal energy. With motors, for example, this isn’t the case. 9 Resistance depends on charge. For example, wires with a larger cross-section offer less resistance to current flow, resulting a lower voltage loss. Conversely, resistance is directly proportional to wire length. To minimize voltage loss, a current needs the shortest possible wire with a large cross-section. the type of wire (copper, iron, etc.) also affects wire resistance. When the resistance in an electrical circuit is near zero, the current may become extremely large, sometimes resulting in what is called a “short-circuit.” A short- circuit will cause an overcurrent within the electrical circuit, and can cause damage to the circuit or device. 10 Electric power (P) is the amount of work done by an electric current in a unit of time. It represents the amount of energy consumed by a device connected to the circuit. It is calculated by multiplying the voltage by the current, and is expressed in Watts (W). P=U×I The more powerful the charge, the more current it consumes. This calculation is useful for analyzing power needs. Energy consumption is the amount of electricity produced or consumed during a given period of time. This is calculated by multiplying the power of a device by the duration of its use, expressed in hours, expressed in kilowatt-hours (kWh). E=P×t 11 Power Vs. Energy Electric energy is often confused with electric power, but they are two different things: Power measures capacity to deliver electricity Energy measures total electricity delivered POWER Watts "like the flow rate of water" Kilowatts ENERGY Watt-hours "like the water that ends up in the Kilowatt- hours bucket" 12 Electric current flows in two ways as an alternating current (AC) or direct current (DC). The main difference between AC and DC lies in the direction in which the electrons flow. In alternating current, the electric charge flow changes its direction periodically. AC is the most commonly used and most-preferred electric power for household equipment, office, buildings, etc. It was first tested based on the principles of Michael Faraday in 1832 using a Dynamo Electric Generator. Alternating current can be identified in a waveform called a sine wave. In other words, it can be referred to as a curved line. These curved lines represent electric cycles and are measured per second. The measurement is read as Hertz (Hz). AC is used in powerhouses and buildings because generating and transporting AC across long distances is relatively easy. AC is capable of powering electric motors which are used in refrigerators, washing machines, etc. 13 Unlike alternating current, the flow of direct current does not change periodically. The current electricity flows in a single direction in a steady voltage. The major use of DC is to supply power to electrical devices and also to charge batteries. Example: mobile phone batteries, flashlights, flat-screen television and electric vehicles. DC has the combination of a plus and a minus sign, a dotted line or a straight line. 14 The major differences between Alternating Current and Direct Current are given in the table below: 15 We are most often concerned with average power rather than its fluctuations—that 60-W light bulb in your desk lamp has an average power consumption of 60 W, for example. As illustrated, the average power Pave is 16 Similarly, we define an average or rms current Irms and average or rms voltage Vrms to be, respectively, where rms stands for root mean square, a particular kind of average. This is useful for AC, since the average value is zero. Now, above. It is standard practice to quote Irms, Vrms, and Pave rather than the peak values. For example, most household electricity is 120 V AC, which means that Vrms is 120 V. The common 10-A circuit breaker will interrupt a sustained Irms greater than 10 A. Your 1.0-kW microwave oven consumes Pave=1.0 kW, and so on. You can think of these rms and average values as the equivalent DC values for a simple resistive circuit. 17 To summarize, when dealing with AC, Ohm’s law and the equations for power are completely analogous to those for DC, but rms and average values are used for AC. Thus, for AC, Ohm’s law is written The various expressions for AC power Pave are 18 Most large power-distribution systems are AC. Moreover, the power is transmitted at much higher voltages than the 120-V AC (240 V in most parts of the world) we use in homes and on the job. Economies of scale make it cheaper to build a few very large electric power-generation plants than to build numerous small ones. This necessitates sending power long distances, and it is obviously important that energy losses en route be minimized. High voltages can be transmitted with much smaller power losses than low voltages, as we shall see. For safety reasons, the voltage at the user is reduced to familiar values. The crucial factor is that it is much easier to increase and decrease AC voltages than DC, so AC is used in most large power distribution systems. 19 Conductors are the materials or substances which allow electricity to flow through them. They conduct electricity because they allow electrons to flow easily inside them from atom to atom. Also, conductors allow the transmission of heat or light from one source to another. Conductors have free electrons on its surface which allow current to pass through easily. This is the reason why conductors are able to conduct electricity. When a charge is transferred to such an element, it gets distributed across the entire surface of the object, which results in the movement of electrons in the object. The charges transferred to an electrical conductor distribute until the force of repulsion between electrons in areas of excess electrons is decreased to the minimum value. When such an object is brought in contact with another conductor, the charge gets transferred from the first conductor to the other until the overall repulsion due to charge is minimized. 20 Insulators are the materials or substances which resist or don’t allow the current to flow through them. In general, they are solid in nature. Also, insulators are finding use in a variety of systems. As they do not allow the flow of heat. They give protection against heat, sound and of course passage of electricity. Furthermore, insulators don’t have any free electrons. It is the main reason why they don’t conduct electricity. 21 Conductors have a property called conductivity which defines their ability to conduct electricity. For insulators, their effectiveness is determined based on how much resistance they offer to the flow of electricity. This property, in contrast to conductivity, is called resistivity. Conductors are essential in applications like wiring, where electric current needs to flow freely. Insulators are important for protecting us from unwanted electric shocks and preventing energy loss in systems like electrical circuits and buildings. Conductors: Used in electrical circuits, power cables, and heating elements. Insulators: Used in the coating of wires, circuit boards, and thermal insulation. 22 1. Power Source (Voltage Source): Provides the necessary energy to drive current through the circuit. Batteries: Provide direct current (DC) by converting chemical energy into electrical energy. AC Power Supply: Provides alternating current (AC), commonly used in homes. 2. Conductors (Wires): Connect various components in the circuit, allowing the flow of current. Typically made from conductive materials like copper or aluminum. 3. Resistor: A device that consumes electric power. It can limit or control the amount of current flowing through the circuit. 4. Switch: Controls the flow of current by opening (turning off) or closing (turning on) the circuit. Manual switches: Like light switches in homes. Automatic switches: Like relays and sensors. 23 5. Capacitor: Stores electrical energy in an electric field and can release it when needed. 6. Inductor: Stores energy in a magnetic field when current flows through it and resists changes in current. 7. Diode: Allows current to flow in only one direction, acting as a one-way valve for electricity. 8. Transistor: Acts as a switch or amplifier for electrical signals. It can control the flow of a large current with a small current. 24 In 1845, a German physicist, Gustav Kirchhoff, developed a pair of laws that deal with the conservation of current and energy within electrical circuits. These two laws are commonly known as Kirchhoff’s Voltage and Current Law. These laws help calculate the electrical resistance of a complex network or impedance in the case of AC and the current flow in different network streams. 25 According to Kirchhoff’s Current Law, The total current entering a junction or a node is equal to the charge leaving the node as no charge is lost. A node refers to a junction connecting two or more current-carrying routes like cables and other components. 26 According to Kirchhoff’s Voltage Law, The voltage around a loop equals the sum of every voltage drop in the same loop for any closed network and equals zero. 27 Two or more resistors are said to be connected in series when the same amount of current flows through all the resistors. In such circuits, the voltage across each resistor is different. In a series connection, if any resistor is broken or a fault occurs, then the entire circuit is turned off. for the above circuit, the total resistance is given as: The total resistance of the system is just the total sum of individual resistances. 28 Two or more resistors are said to be connected in parallel when the voltage is the same across all the resistors. In such circuits, the current is branched out and recombined when branches meet at a common point. A resistor or any other component can be connected or disconnected easily without affecting other elements in a parallel circuit. The sum of reciprocals of resistance of an individual resistor is the total reciprocal 29 resistance of the system. A capacitor is made of two conducting sheets (called plates) separated by an insulating material (called the dielectric). The plates will hold equal and opposite charges when there is a potential difference between them. When a potential difference V exists between the two plates, one holds a charge of +Q and the other holds an equal and opposite charge of −Q. The total charge is zero, Q refers to the charge which has been moved from one plate to the other. The voltage between the plates and the charge held by the plates are related by a term known as the capacitance of the capacitor. Capacitance is defined as: 𝑄 𝐶= 𝑉 The unit of capacitance is the farad (F), equivalent to one coulomb stored for each volt of potential difference. The capacitance C of a parallel plate capacitor with plates each having cross sectional area A, separated by a distance d is given by 𝜀0 𝐴 𝐶= 𝑑 30 where 𝜀0 is the permittivity of free space with value 8.85×10^(−12)F/m. The energy stored on a capacitor of capacitance C, charged to a voltage V and carrying a charge Q=CV is: 1 2 𝐸= 𝐶𝑉 2 If two capacitors of capacitance C1 and C2 are connected in parallel to an input voltage V, then the potential difference across the two capacitors will be the same and equal to V. If Q is the total amount of charge flow (see above) then Q=Q1+Q2 where Q1 is stored in the first capacitor and Q2 is stored in the second capacitor. Using the definition of capacitance, we thus have 𝑄 𝐶𝑒𝑞= = (𝑄1+ 𝑄2)/𝑉 = (𝐶1𝑉 + 𝐶2𝑉)/𝑉 = 𝐶1+ 𝐶2 𝑉 The formula also generalises to n capacitors in parallel: 𝐶𝑒𝑞= 𝐶1+ ⋯ + 𝐶𝑛 31 If two initially uncharged capacitors of capacitance C1 and C2 are connected in series to an input voltage V, then each capacitor must carry the same opposite charges on its plates, regardless of the values of C1 and C2. Consider charge flowing through the circuit. By the conservation of charge, as positive charge accumulates on one outer plate, negative charge must equally accumulate on the other outer plate. Hence the charge stored on each inner plate must be of equal magnitude and opposite sign to its associated outer plate. So, as shown in the diagram, both capacitors must separate equal charge Q. However, the voltage drops across the capacitors may be different; denote them by V1 and V2. The total charge which will flow in the rest of the circuit will be equal to Q (and not to Q+Q=2Q). Since V=V1+V2 by Kirchhoff's Voltage Law, we obtain 𝑄 𝑄 1 1 𝐶𝑒𝑞= 𝑄/𝑉 = 𝑄/(𝑉1+ 𝑉2) = 𝑄/( + ) = 1/( + ). 𝐶1 𝐶2 𝐶1 𝐶2 That is, 1 1 1 = + 𝐶𝑒𝑞 𝐶1 𝐶2 1 1 1 For n of capacitors in series, = + ⋯+ 32 𝐶𝑒𝑞 𝐶1 𝐶𝑛 Initially there is no charge on the capacitor. The battery will cause a current to flow and charge to build up on the capacitor. By using Kirchhoff's Voltage Law we can write down a relationship between the voltages around the circuit: V=VR+VC, then by using Ohm's Law, and the definition of capacitance, we have Rearranging to solve the equation gives: Here VC is the largest charge that will be held on the capacitor and Q(t) is the charge on the capacitor after a time t. Differentiating this expression gives an equation for current. RC is known as the time constant, τ and represents the time taken for the capacitor 33 to reach approximately 63% of its maximum charge. Here the switch is open and the capacitor is charged. The switch is closed at time t=0s and the capacitor starts to discharge. The rate of discharge can be determined by looking at the voltage over the two components. Given that the components are connected together directly, VR=VC when the switch is 'on' (closed). Separating the variables gives: where Q0 is the charge on the capacitor at t=0s and Q(t) is the charge on the capacitor at time t. This leads to the final expression: RC is known as the time constant, τ and is the time taken for the capacitor's charge to drop to approximately 37% of its original value. 34 Ceramic Capacitors: Electrolytic Capacitors: Common for high-frequency and Have higher capacitance values (μF to general-purpose applications. mF) but are polarized (must be connected Available in small values (pF to μF). with correct polarity). Used in power supply filtering and energy storage. Tantalum Capacitors: Known for stability and reliability, Supercapacitors (or ultracapacitors): often used in precision circuits. Store much more energy than typical Smaller in size for the same capacitors but less than batteries. capacitance compared to Used for quick bursts of energy in electrolytics but more expensive. applications like regenerative braking in electric vehicles. Film Capacitors: Typically used in audio circuits and high-precision applications. Offer excellent stability and low distortion. 35 Energy Storage: Capacitors store energy that can be discharged quickly when needed (e.g., in camera flashes or audio amplifiers). Filtering: In power supplies, capacitors smooth out voltage fluctuations. Coupling and Decoupling: Capacitors block DC while allowing AC signals to pass, making them useful in signal processing. Tuning Circuits: In radios and other communication devices, capacitors are used in resonance circuits for tuning. 36 An inductor is a passive electrical component that stores energy in a magnetic field when electric current flows through it. It typically consists of a coil of wire, and the inductance (measured in henries, H) depends on factors like the number of turns in the coil, the material around which the coil is wound (core), and the coil's geometry. a coil has an inductance, ( L ) of one Henry, ( 1H ) when the current flowing through the coil changes at a rate of one ampere/second, ( A/s ). This change induces a voltage of one volt, ( VL ) in it. L is in Henries N is the Number of Turns Φ is the Magnetic Flux 37 Ι is in Amperes Series inductors have a Common Current flowing through them, the sum of the individual voltage drops across each inductor can be found using Kirchoff’s Voltage Law where, VT = V1 + V2 + V3 38 Inductors in Parallel have a Common Voltage across them. The sum of the individual currents flowing through each inductor can be found using Kirchoff’s Current Law (KCL) where, IT = I1 + I2 + I3 39 Air-Core Inductors: Inductors without a Iron-Core Inductors: These have an iron core to boost magnetic core, used in high-frequency inductance and are used in power supplies and transformers applications like radio transmission. for lower frequency applications. Ferrite-Core Inductors: With a ferrite Toroidal Inductors: Shaped like a doughnut, these inductors have core, they are used in high-frequency a toroidal core, which helps confine the magnetic field, making applications such as RF circuits and them efficient and reducing electromagnetic interference (EMI). switching power supplies. 40 Energy Storage: Inductors store energy in magnetic fields and are used in power supplies and DC-DC converters to smooth out voltage and current. Filtering: In combination with capacitors, inductors form LC filters, which block certain frequency ranges in power and signal processing circuits. Transformers: Inductors are key components in transformers, where they transfer energy between circuits via electromagnetic induction. Chokes: Inductors are used to block high-frequency AC while allowing low- frequency or DC signals to pass. 41 A diode is a semiconductor device that allows current to flow in one direction while blocking it in the opposite direction. It has two terminals: the anode (positive side) and the cathode (negative side). Diodes are essential components in many electronic circuits for rectification, protection, and signal processing. 42 Rectifier Diodes: Used in power supplies to convert AC to DC. They are designed to handle high currents. Zener Diodes: Used for voltage regulation. They allow current to flow in reverse if the voltage exceeds a certain threshold (called Zener voltage), thus stabilizing voltage in circuits. Schottky Diodes: Have a lower forward voltage drop and are used in high-speed switching applications. They're ideal for circuits where power efficiency is critical. Light-Emitting Diodes (LEDs): Emit light when forward biased. LEDs are widely used for display, indicator, and lighting applications. Photodiodes: Detect light by generating current when exposed to photons, commonly used in sensors and solar cells. Tunnel Diodes: These exhibit negative resistance due to quantum tunneling and are used in specialized high-frequency circuits. 43 44 Rectification: In power supplies, diodes are used to convert AC into DC. Voltage Regulation: Zener diodes are used to maintain a constant voltage across a circuit. Signal Demodulation: Diodes are used in radio receivers to extract audio signals from modulated carrier waves. Protection: Diodes protect circuits from voltage spikes by allowing current to bypass sensitive components during voltage surges (e.g., clamping diodes). 45 A transistor is a semiconductor device used to amplify or switch electronic signals and electrical power. It is one of the fundamental building blocks of modern electronic devices. Transistors are widely used in various applications such as signal amplification, switching, and digital circuits. Emitter (E): The terminal that emits charge carriers (electrons or holes), usually connected to the negative side in an NPN transistor or the positive side in a PNP transistor. Base (B): The terminal that controls the transistor’s operation by allowing a small current or voltage to control the flow of a larger current. Collector (C): The terminal that collects the charge carriers emitted by the emitter, completing the current flow through the 46 transistor. Bipolar Junction Transistors (BJT): NPN: In this type of BJT, current flows from the collector to the emitter when a small current is applied to the base. PNP: In a PNP transistor, current flows from the emitter to the collector when a small current is applied to the base. Field-Effect Transistors (FET): JFET (Junction FET): A voltage applied to the gate terminal controls the current between the source and the drain. MOSFET (Metal-Oxide-Semiconductor FET): A voltage at the gate controls the flow of current between the source and drain, making it widely used in digital circuits. 47