Basic Biochemistry PDF
Document Details
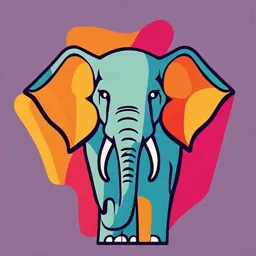
Uploaded by TopNotchHydrogen
Medical School
Dr. Kifaya Azmi
Tags
Summary
These lecture notes cover basic biochemistry, focusing on water's properties and role in biological systems. Topics include the structure of water, hydrogen bonding, and the effects of water on biological molecules. A general overview of important biological principles is also included.
Full Transcript
BASIC Biochemistry Biochemistry and molecular Biology Department Medical school CHAPTER 2 WATER DR. KIFAYA AZMI Water The most abundant substance in living systems, (70% of the weight of most organisms). The water molecule and its ionizati...
BASIC Biochemistry Biochemistry and molecular Biology Department Medical school CHAPTER 2 WATER DR. KIFAYA AZMI Water The most abundant substance in living systems, (70% of the weight of most organisms). The water molecule and its ionization products H+ and OH-, influence the structure, self assembly and properties of all cellular components (proteins, nucleic acids and lipids). The noncovalent interactions among biomolecules are influenced by the solvent properties of water including its ability to form hydrogen bonds with itself and with solutes. Water is a versatile solvent due to its polarity, which allows it to form hydrogen bonds easily. When an ionic compound is dissolved in water, each ion is surrounded by a sphere of water molecules called a hydration shell. 2.1 Weak Interactions in Aqueous Systems ◼ Hydrogen Bonding Gives Water Its Unusual Properties ◼ Water Forms Hydrogen Bonds with Polar Solutes ◼ Water Interacts Electrostatically with Charged Solutes ◼ Entropy Increases as Crystalline Substances Dissolve ◼ Nonpolar Gases Are Poorly Soluble in Water ◼ Nonpolar Compounds Force Energetically Unfavorable Changes in the Structure of Water ◼ van der Waals Interactions Are Weak Interatomic Attractions ◼ Weak Interactions Are Crucial to Macromolecular Structure and Function ◼ Solutes Affect the Colligative Properties of Aqueous Solutions 1. water and its unusual properties ◼ Water has a higher melting point, boiling point, and heat of vaporization than most other common solvents. attractions between adjacent water molecules that give liquid water great internal cohesion (hydrogen bond = H bond). 2. Structure of the water molecule 1. Polar 2. Tetrahedral 3. bond angle is 104.5o and not 109.5o of a perfect tetrahedron **due to electron crowding of the nonbonding orbital of the oxygen atom. 4. Sharing of electrons is unequal.The oxygen nucleus attracts electron more strongly than does the hydrogen nucleus (PROTON) the electrons are more often in the vicinity of the oxygen atom (2d-) than the hydrogen (d+), which generate an electrostatic attraction between the oxygen atom of one water molecule and the hydrogen of another--- 3. Ability to form Hydrogen bond 1. Length of hydrogen bond vs covalent 2. Hydrogen bonds are relatively weak. bond dissociation energy (the energy required to break a bond) of about 23 kJ/mol, compared with 470 kJ/mol for the covalent O—H bond in water. Bond dissociation energy 23 kJ/mol Weak bond 470 kJ/mol Strong bond ◼ Life span hydrogen bond is just 1 to 20 picoseconds (where 1 ps= 10-12 s) ◼ On breaking of one hydrogen bond, another hydrogen bond is formed in 0.1 ps. ◼ Phrase for this phenomena is known “flickering clusters” applied to the short-lived groups of water molecules interlinked by hydrogen bonds in liquid water. ◼ Water molecules are disorganized and in continuous motion. At any moment one (1) molecule of water forms 3.4 H-bonds in liquid state. H2O in regular lattice structure 1. Density of ice is less than water in liquid. 2. Ice form Hexagonal lattice by forming H-bond. thus ice floats on liquid water. This crystal lattice of ice makes it less dense than liquid and regular. 3. Bond length of hydrogen bond in ice is 0.274nm (whereas in liquid state the H-bond length is 0.177nm). 4. The maximum number of hydrogen bonds that one water molecule can have with neighboring water molecules: 4 Hydrogen bond not unique to water. Common hydrogen bonds in biological systems. The hydrogen acceptor is usually oxygen or nitrogen; the hydrogen donor is another hydrogen that bonded with electronegative atom. Water forms Hydrogen Bonds with Polar solutes 1. Hydrogen bonds are not unique to water. 2. They readily form between an electronegative atom (the hydrogen acceptor, usually O or N with a lone pair of electrons) and a hydrogen atom covalently bonded to another electronegative atom (the hydrogen donor) in the same or another molecule. 3. Hydrogen atoms covalently bonded to carbon atoms do not participate in hydrogen bonding, because carbon is only slightly more electronegative than hydrogen and thus the C-H bonds is only very weakly polar. 4. Alcohols, aldehydes, ketones and compounds containing N-H bonds all form hydrogen bonds with water molecules and tend to be soluble in water. Some biologically important hydrogen bonds Directionality of the hydrogen bond Acceptor atom O ◼ Hydrogen bonds are strongest when the bonded molecules oriented to maximize electrostatic interaction, which occurs when the hydrogen atom and the two atoms that share it are in a straight line - that is, when the acceptor atom is in line with the covalent bond between the donor atom and H - holding two hydrogen bonded molecules or groups in a specific geometric arrangement. ◼ Charged or polar molecules are dissolved easily in water (Hydrophilic). ◼ Nonpolar solvents are poor solvents for polar molecule but easily dissolved hydrophobic compound (lipid or waxes). ◼ Amphipathic compounds contain regions that are polar or (charged) and nonpolar ◼ Ex:Fatty acids Bioeneregetics Gibbs free energy, G The amount of energy capable of doing work during a reaction (at constant temperature and pressure). Exergonic reaction: When a reaction proceeds with the release of free energy the free-energy change, ΔG, has a negative value. Endergonic reaction: the system gains free energy and ΔG is positive. Enthalpy, H The heat content of the reacting system. It reflects the number and kinds of chemical bonds in the reactants and products. Exothermic reaction: When a chemical reaction releases heat the heat content of the products is less than that of the reactants H has a negative value. Endothermic reaction: Reacting systems that take up heat from their surroundings have positive values of H. Entropy, S A quantitative expression for the randomness or disorder in a system. When the products of a reaction are less complex and more disordered than the reactants, the reaction is said to proceed with a gain in entropy. Enthalpy is related with the first law of thermodynamics where it says, “Energy can be neither created nor destroyed.” But the entropy is directly related with the second law of thermodynamics. Entropy Increases as Crystalline Substances Dissolve Water as solvent. 1. Water dissolves many crystalline salts by hydrating their component ions. 2. The NaCl crystal lattice is disrupted as water molecules cluster about the Cl and Na ions. 3. The ionic charges are partially neutralized, and the electrostatic attractions necessary for lattice formation are weakened. Nonpolar Gases Are Poorly Soluble in Water Why CO2 Nonpolar (in blood): O2 → Hemoglobin CO2 → Hemoglobin + H2CO3- system Polar molecules dissolves better at low T than do nonpolar molecules at high T Nonpolar compounds force energetically unfavorable changes in the structure of water Amphipathic compounds in aqueous solution. Long-chain fatty acids have very hydrophobic alkyl chains, each of which is surrounded by a layer of highly ordered water molecules. No compensation like NaCl The stable structure of Amphipathic compound in water=micelles. By clustering fatty acid molecules together in micelles: they expose the smallest possible hydrophobic surface area to the water. fewer water molecules are required in the shell of ordered water. the energy gained by freeing immobilized water molecules stabilizes the micelle. What happen with enzyme substarte complex?? Release of ordered water ! Part of the driving force for binding of a polar substrate to the complementary polar surface of an enzyme is… the entropy increase as the enzyme displaces, ordered water from the substrate. the resulting increase in entropy provides a thermodynamic push toward formation of the enzyme- substrate complex. Weak Interactions are Crucial to Macromolecular Structure and Function Four types of noncovalent (“weak”) interactions among biomolecules in aqueous solvent: 1. Hydrogen bonds (between polar groups) 2. Ionic interactions (between charged groups) 3. Hydrophobic interactions (between nonpolar) 4. Van der Waals interactions (attraction and repulsion) two uncharged atoms are brought very close together… their surrounding electron clouds influence each other… random variations in the position of the electrons around one nucleus may create a transient, opposite electric dipole in the nearby atom... The two dipoles weakly attract each other, bringing the two nuclei closer. The cumulative effect of many such interactions can be very significant Weak Interactions are Crucial to Macromolecular Structure and Function Although these four types of interactions are individually weak relative to covalent bonds, the cumulative effect of many such interactions can be very significant. The folding of a single polypeptide or polynucleotide chain into its three-dimensional shape is determined by this principle. The binding of an antigen to a specific antibody depends on the cumulative effects of many weak interactions. The energy released when an enzyme binds non covalently to its substrate is the main source of the enzyme's catalytic power. The binding of a hormone or a neurotransmitter to its cellular receptor protein is the result of weak interactions. Solutes Affect the Colligative Properties of Aqueous Solutions Colligative (“tied together”) properties: vapor pressure, boiling point, melting point (freezing point), and osmotic pressure. Solutes of all kinds alter colligative properties of water by lowering the effective concentration of water The effect of solute concentration on the colligative properties of water is: independent of the chemical properties of the solute. depends only on the number of solute particles (molecules, ions) in a given amount of water. has the same basis: the concentration of water is lower in solutions than in pure water. Osmosis When two different aqueous solutions are separated by a semipermeable membrane… Water molecules diffuse from the region of higher water concentration to that of lower water concentration The water movement produces osmotic pressure Osmosis: water movement across a semipermeable membrane driven by differences in osmotic pressure. important factor in the life of most cells: ✓ Plasma membranes are more permeable to water than to most other small molecules, ions, and macromolecules. ✓ due (partly) to simple diffusion of water through the lipid bilayer and (partly) to protein channels in the membrane that selectively permit the passage of water. Osmosis and the measurement of osmotic pressure Water flows from the beaker Osmotic pressure is measured as into the tube to equalize its the force that must be applied to concentration across the return the solution in the tube to membrane At equilibrium, the force of gravity operating on the the level of that in the beaker. solution in the tube balances the tendency of water to move into the tube. Effect of extracellular osmolarity on water movement across a plasma membrane: Isotonic: ✓ solutions of equal osmolarity. ✓ surrounded by an isotonic solution, a cell neither gains nor loses water. Hypertonic: ✓ solution with higher osmolarity than the cytosol. ✓ the cell shrinks as water flows out. Hypotonic: ✓ solution with lower osmolarity than the cytosol. ✓ the cell swells as water enters. Let’s think about this ! Cells generally contain higher concentrations of biomolecules and ions than their surroundings … so osmotic pressure tends to drive water into cells…. If not counterbalanced, this would cause bursting of the cell (osmotic lysis) !!! To prevent this catastrophe… blood plasma and interstitial fluid are maintained at an osmolarity close to that of the cytosol: 1. High concentration of albumin and other proteins in blood plasma. 2. Cells actively pump out ions (such as Na+) into the interstitial fluid to stay in osmotic balance with their surroundings. Effect of macromolecules and monomers on Osmosis The effect of solutes on osmolarity depends on the number of dissolved particles (not their mass) Therefore, macromolecules have far less effect on the osmolarity of a solution than would an equal mass of their monomeric components. e.g. 1g of polysaccharide (composed of 1,000 glucose units) has the same effect on osmolarity as a mg of glucose. o That’s why polysaccharides are stored rather than simple sugars in order to prevent the enormous increase in osmotic pressure within the storage cell. Glycogen 2.2 Ionization of Water, Weak Acids, and Weak Bases ◼ Pure Water Is Slightly Ionized ◼ The Ionization of Water Is Expressed by an Equilibrium Constant ◼ The pH Scale Designates the H+ and OH- Concentrations ◼ Weak Acids and Bases Have Characteristic Dissociation Constants ◼ Titration Curves Reveal the pKa of Weak Acids Hydronium ions and proton hopping ◼ 1. Hydrogen ions formed in water are immediately hydrated to hydronium ions (H3O+). ◼ 2. No individual proton moves very far through the bulk solution, but a series of proton hops between hydrogen bonded water molecules causes the net movement of a proton over a long distance in a remarkably short time. The ionization of water is expressed by an equilibrium constant (Keq) ion-product of water=Kw Kw is found by multiplying Keq by the concentration of water. Application exercises The ion product of water, Kw, is the basis for the pH scale pH = -log [H+] if concentration is 1.0×10-7M pH = log (1.0×10-7) = 7 The pH of an aqueous solution reflects, on a logarithmic scale, the concentration of hydrogen ions The pH Scale & some aqueous fluids The pH scale is logarithmic, not arithmetic. Ex: two solutions differ in pH by 1 pH unit means that one solution has ten times the H concentration of the other. A cola drink (pH 3.0) or red wine (pH 3.7) has an H concentration approximately 10,000 times that of blood (pH 7.4). Weak acids and bases have characteristic dissociation constants HA H+ + A- K eq = H A + − =K HA a 1 pKa = log = − log K a Ka pKa = _ log Ka (analogous to pH) Equilibrium constants for ionization reactions are usually called ionization or dissociation constants, often designated Ka. Henderson-Hasselbalch equation Ka = H A + − 1. solve for H conc H = Ka + HA HA A − − logH = − log Ka − log + HA 2. take -log A − 3. Substitute pH for –log H conc) pH = pKa + log A − HA 4. Inver pH = pKa + log proton _ acceptor Conjugated base proton _ donor Conjugated acid The Henderson-Hasselbalch equation can be used to calculate 1) the pH of a solution of an organic acid. 2) the amount of salt and acid to add to form a specific buffer. 3) the pKa of a weak acid. Acids: proton donors Bases: proton acceptors. A proton donor and its corresponding proton acceptor make up a conjugate acid-base pair Strong acids/bases (Completely ionized) ◼ Hydrochloric ◼ NaOH ◼ Sulfuric ◼ KOH ◼ Nitric acids Weak acids/bases (not completely ionized) ◼ Important in biological systems. ◼ Metabolism regulation The stronger the acid, the greater its tendency to lose its proton. The tendency of any acid (HA) to lose a proton and form its conjugate base (A-) is defined by the equilibrium constant (Keq) for the reversible reaction Equilibrium constants for ionization reactions are usually called ionization or dissociation constants, often designated Ka. pKa = _ log Ka (analogous to pH) The stronger the tendency to dissociate a proton, the stronger is the acid and the lower its pKa Conjugate acid-base pairs consist of a proton donor and a proton acceptor Titration curves reveal the pKa of weak acids (acetic acid)..acetate anion ◼ The conc. of the acid in a givin solution can be calculated from the volume and concentration of NaOH added--- titration curve. ◼ See the axis: PH and NaOH amount added ◼ At the midpoint of the titration, at which exactly 0.5 equivalent of NaOH has been added, [HA]=[A-] and pH=pKa ◼ At this midpoint a very important relationship holds: the pH of the equimolar solution of acetic acid and acetate is exactly equal to the pKa of acetic acid (pKa = 4.76) K eq = H A + − =K HA a Flat zone:is the buffering region of the acetic acid acetate buffer pair Titration Curves Titration curves are used to determine the amount of an acids in a given solution. A measured volume of the acids is titrated with a solution of a strong base, usually NaOH of known concentration. A plot of pH against the amount of NaOH added (a titration curve) reveals the pKa of the weak acid. Consider the titration of 0.1M solution of acetic acid (HAc) with 0.1M NaOH at 25oC As Na OH is gradually introduced, the added OH- combines with the free H+ in the solution to form H2O. As free H+ is removed, HAc dissociates further to satisfy its own equilibrium constant, the net result as the titration proceeds is that more and more HAc ionizes, forming Ac-, as the NaOH is added. At the midpoint of the titration at which exactly 0.5 equivalent of NaOH has been added, one half of the original acetic acid has undergone dissociation, so that the concentration of the proton donor (HAc) now equals that of the proton acceptor, [Ac-]. At this midpoint a very important relationship holds: the pH of the equimolar solution of acetic acid and acetate is exactly equal to the pKa of acetic acid (pKa = 4.76) The acetic acid – acetate pair as a buffer system When H+ or OH is added to buffer: a small change in the ratio of the relative concentration of the weak acid and its anion → a small change in pH. ◼ Buffers tend to resist changes in pH when small amounts of acids or base added. ◼ At 0.5 NAOH added, proton acceptor eq.proton donor Buffers Are Mixtures of Weak Acids and Their Conjugate Bases Comparison of the titration curves of three weak acids ◼ The titration curves of these acids have the same shape, they are displaced along the pH axis because the three acids have different strengths. 1. Acetic acid, with the highest Ka (lowest pKa, lowest pH) of the three, is the strongest (loses its proton most readily); it is already half dissociated at pH 4.76. ◼ 2. Dihydrogen phosphate loses a proton less readily, being half dissociated at pH 6.86. ◼ 3. Ammonium ion is the weakest acid of the three and does not become half dissociated until pH 9.25. 2.3 Buffering against pH Changes in Biological Systems ◼ Buffers Are Mixtures of Weak Acids and Their Conjugate Bases ◼ A Simple Expression Relates pH, pKa, and Buffer Concentration (Henderson- Hasselbalch equation) ◼ Weak Acids or Bases Buffer Cells and Tissues against pH Changes Buffering against pH Changes in Biological Systems Weak acids or bases buffer cells and tissues against pH changes Work example ◼ cytoplasm cells contain ionizable groups: Protein (histidine pKa=6.0, buffer near neutral PH), nucleotides such ATP, low MW metabolites. The organism’s first line of defense against changes in internal pH is provided by buffer systems Buffering System in Cells Phosphate & Bicarbonate Phosphate buffer (cytoplasm, ECF) Maximally effective at a pH close to its pKa of 6.86 and thus tends to resist pH changes in the range between about 5.9 and 7.9. It is therefore an effective buffer in biological fluids; in mammals, extracellular fluids and most cytoplasmic compartments have a pH in the range of 6.9 to 7.4. Buffering System in Cells Phosphate & Bicarbonate Bicarbonate buffer (plasma) The pH of a bicarbonate buffer is determined by the concentration of HCO3- in the aqueous phase and the partial pressure of CO2 in the gas phase. Effective physiological buffer near pH 7.4 The CO2 in the air space of the lungs is in equilibrium with the bicarbonate buffer in the blood plasma passing through the lung capillaries Carbonic acid bicarbonate ◼ Reaction 1: When H is added to blood as it passes through the tissues,- proceeds toward a new equilibrium, in which the concentration of H2CO3 is increased. ◼ Reaction 2 :This increases the concentration of CO2(d) in the blood plasma ◼ Reaction 3: thus increases the pressure of CO2(g) in the air space of the lungs.The extra CO2 is exhaled. ◼ Conversely, when the pH of blood plasma is raised (i.e. by NH3 production during protein catabolism), Lactic acid formation NH3 produced during protein catabolism ◼ The H concentration of blood plasma is lowered, causing more H2CO3 to dissociate into H and HCO3. This in turn causes more CO2(g) from the lungs to dissolve Human blood plasma pH 7.4. Uncontrolled diabetes: over production of metabolic acids → acidosis [pH of the blood ≤ 6.8] → irreparable cell damage and death. H2CO3 [blood plasma] is in equilibrium with CO2(g) [air space of the lungs]. involves three reversible equilibria. Abnormalities 1. Respiratory Acidosis – CO2 in blood; pH 2. Respiratory alkalosis – CO2; pH 3. Metabolic acidosis – HCO3- ; pH 4. Metabolic alkalosis – HCO3- ; pH The pH optima of some enzymes pH optimum: The pH at which Enzymes show maximal catalytic activity. Biological control of pH of cells and body fluids is important because: A small change in pH can make a large difference in the rate of some crucial enzyme-catalyzed reactions. 2.4 Water as a reactant ◼ Condensation reaction: in which the element of water are eliminated. ◼ Hydrolysis reaction: cleavage accompanied by the addition of the water (reverse)