B - A - 3.12 - DC Motor Generator Theory PDF
Document Details
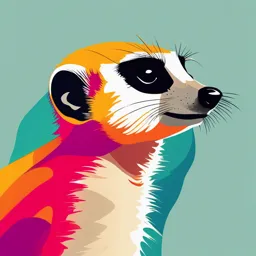
Uploaded by GoodMilkyWay
Emirates Aviation University
Tags
Summary
This document is a module on DC motor and generator theory from Emirates Aviation University. It covers basic concepts, components, and operation of DC generators and motors. It also introduces starter generators.
Full Transcript
Module 3: Electrical Fundamentals Topic 3.12: DC Motor/Generator Theory INTRODUCTION On completion of this topic you should be able to: 3.12.1 Describe basic motor and generator theory. 3.12.2 Identify components of a DC generator and de...
Module 3: Electrical Fundamentals Topic 3.12: DC Motor/Generator Theory INTRODUCTION On completion of this topic you should be able to: 3.12.1 Describe basic motor and generator theory. 3.12.2 Identify components of a DC generator and describe their construction and purpose. 3.12.3 Describe the operation of DC generators and factors affecting: Output Direction of current flow 3.12.4 Describe the operation of DC motors and factors affecting: Output power Torque Speed Direction of rotation 3.12.5 Describe the operation and features of the following DC motors: Series wound Shunt wound Compound 3.12.6 Describe the construction and operation of starter generators. 30-03-2024 Slide No. 2 LEFT HAND RULE MAGNETIC FIELDS ARE PERPENDICULAR TO CONDUCTOR Direction of magnetic field dependent on direction of current flow in conductor. Simple rule for remembering direction of magnetic field around a conductor. Left-Hand Rule Grasp conductor in left hand with thumb pointing in direction of current. Fingers will circle conductor in direction of magnetic field. This rule for Electron Flow ONLY. 30-03-2024 Slide No. 3 MAGNETIC FIELD PATTERNS MAGNETIC FIELD AROUND CONDUCTORS CARRYING CURRENT Imagine you are looking at conductors end on. Cross indicates tail of arrow / feather / conductor (heading away from you). Point indicates front of arrow / conductor (coming towards you). Using Left-Hand Rule, magnetic field direction is worked out. Magnetic field around a current-carrying conductor is perpendicular to conductor. 30-03-2024 Slide No. 4 MAGNETIC FIELD AROUND PARALLEL CONDUCTORS 2 parallel conductors – current in same direction – magnetic lines of force combine. Increases strength of field around conductors – aid each other. 2 parallel conductors – currents in opposite directions – lines of force oppose. Deforms field around each conductor – repel each other. 30-03-2024 Slide No. 5 BASIC AC GENERATION 1 way of producing an emf – magnetism and movement. A changing magnetic field produces an emf in a conductor. An emf is induced in a conductor if placed in a magnetic field, and either: The field is changing around the conductor The conductor moves through magnetic field This effect is called electromagnetic induction. 30-03-2024 Slide No. 6 VOLTAGE PRODUCED BY MAGNETISM AND MOTION 3 conditions must exist before emf produced by magnetism: Must be a conductor in which voltage will be produced Must be a magnetic field in vicinity of conductor Must be relative motion between field and conductor Conductor must be moved to cut magnetic lines of force. Magnetic field must be moved so flux cuts across conductor. When conductor cuts lines of force, electrons are propelled. This creates the electric motive force (emf) or voltage. 30-03-2024 Slide No. 7 LEFT HAND RULE FOR GENERATORS First, position your LEFT HAND with the fingers as shown. Your THUMB will point in direction of rotation (relative movement of wire to field). Your INDEX FINGER will point in direction of magnetic flux (north to south). Your MIDDLE FINGER will point in direction of electron current flow. Direction of current flow can be determined for any conductor in a magnetic field. 30-03-2024 Slide No. 8 BASIC ALTERNATING-CURRENT GENERATOR Position A @ Zero degrees: Conductor rotated clockwise through magnetic field between poles of permanent Magnet. Orange half is moving parallel to lines of force – cutting NO lines of force. Same is true of purple half moving in opposite direction. Since conductors are cutting NO lines of force – NO emf is induced. 30-03-2024 Slide No. 9 BASIC ALTERNATING-CURRENT GENERATOR Position B: As loop rotates toward position (B) – it cuts more and more lines of force. Induces an ever-increasing voltage due to cutting more directly across field. At (B), conductor has completed one-quarter of a complete revolution (90° of 360°). Conductor is now cutting directly across field – voltage induced is maximum. 30-03-2024 Slide No. 10 BASIC ALTERNATING-CURRENT GENERATOR Position C: As conductor continues rotation toward (C) – it cuts fewer and fewer lines of force. Induced voltage decreases from its peak value – at (C) there is NO induced emf. 30-03-2024 Slide No. 11 BASIC ALTERNATING-CURRENT GENERATOR Position D: As loop rotates toward position (D) – it cuts more and more lines of force. At Position (D) - Conductor is now cutting directly across field – voltage induced is maximum. – however polarity has changed. 30-03-2024 Slide No. 12 BASIC ALTERNATING-CURRENT GENERATOR Value of induced voltage at various points during rotation is shown – sine wave. 30-03-2024 Slide No. 13 DC GENERATOR THEORY Sine curve shows value of induced voltage at each instant of time during rotation. Notice that this curve contains 360°, or two alternations. 2 alternations represent ONE complete CYCLE of rotation. In DC generators – AC is always induced in output windings. Generated current is rectified to DC mechanically by a commutator and brushes. 30-03-2024 Slide No. 14 ELEMENTARY DC GENERATOR A single-loop generator. Each conductor end connected to a segment of a 2-segment metal ring. 2 segments are insulated from each other – forms a simple COMMUTATOR. Commutator in a DC generator replaces slip rings of AC generator. This is the main difference in their construction. 30-03-2024 Slide No. 15 ELEMENTARY DC GENERATOR Commutator – mechanically reverses armature loop connections to external circuit. This occurs at same instant that the polarity of voltage in armature loop reverses. Commutator changes generated AC voltage to a pulsating DC voltage. This action is known as COMMUTATION. 30-03-2024 Slide No. 16 ELEMENTARY DC GENERATOR Voltage developed across brushes is pulsating and unidirectional. It varies twice during each revolution between zero and maximum. This variation is called RIPPLE. A pulsating voltage is unsuitable for most applications. Practical generators - more armature loops (coils) and more commutator segments. This will produce an output voltage waveform with less ripple. 30-03-2024 Slide No. 17 TYPICAL GENERATOR CONSTRUCTION Major parts of DC Generator: Field Frame or Yoke Armature Commutators Brushes Note laminated field poles to reduce eddy currents. 30-03-2024 Slide No. 18 TYPICAL 24-VOLT DC GENERATOR 30-03-2024 Slide No. 19 GENERATOR FIELD FRAME The field frame, or yoke, has 2 primary functions: Completes magnetic circuit between poles Acts as mechanical support for other generator parts In small generators – made of 1 piece of iron. In larger generators – usually made up of 2 parts bolted together. Highly permeable and, together with pole pieces, forms majority of magnetic circuit. 30-03-2024 Slide No. 20 GENERATOR FIELD FRAME FIELD COIL AND POLE SHOE Magnetising force is produced by: Electromagnet consisting of a wire coil called a field coil. Core called a field pole, or pole shoe – bolted to inside of the frame. Usually laminated to reduce eddy current losses Concentrates lines of force produced by field coils Always one north pole for each south pole – always an even number of poles. 30-03-2024 Slide No. 21 EFFECTS OF ADDITIONAL COILS Effects of additional coils illustrated by addition of a 2nd coil to armature. Commutator must now be divided into 4 parts since there are 4 coil ends (2 coils). Coil is rotated in a clockwise direction from the position shown. emf induced in white coil decreases for next 90° of rotation (from maximum to zero. emf induced in red coil increases from zero to maximum at same time. 30-03-2024 Slide No. 22 EFFECTS OF ADDITIONAL COILS With 4 commutator segments – a new segment passes each brush every 90°. Allows brush to switch from white to red coil at instant voltages in 2 coils are equal. Brush remains in contact with red coil as its induced voltage increases to maximum. As it decreases to level A (90° later), the brush will contact the white coil again. Average voltage level Average voltage output level is thus higher with more coils/commutator segments. 30-03-2024 Slide No. 23 EFFECTS OF ADDITIONAL COILS Variation in DC voltage is called ripple – reduced by adding more coils. The more conductors – more voltage peaks per revolution – less ripple. More coils used – the closer the output resembles pure DC. Diagram corresponds to the graph at the 0 degrees position. 30-03-2024 Slide No. 24 EFFECTS OF ADDITIONAL POLES Practical generators use many armature coils. They also use more than one pair of magnetic poles. Additional magnetic poles have same effect as do additional armature coils. Increased number of poles provides a stronger magnetic field (more flux lines). Increases output voltage because coils cut more lines of flux per revolution. 30-03-2024 Slide No. 25 ELECTROMAGNETIC POLES Nearly all generators use electromagnetic poles instead of permanent magnets. Consist of coils of insulated copper wire wound on soft iron cores. Main advantages of using electromagnetic poles are: Increased field strength Means of controlling strength of fields By varying input voltage – field strength is varied. By varying field strength – output voltage of generator can be controlled. 30-03-2024 Slide No. 26 BRUSHES Ride on surface of commutator. Act as electrical contact between armature coils and external circuit. Made of high grade carbon – may include molybdenum for lubrication. Held in place by spring-loaded brush holders that are insulated from frame. Free to slide up and down in holders – allows for wear and irregularities. High altitude, self lubricating brushes used in aircraft – drier atmospheric conditions. Flexible braided-copper conductor (pig-tail) connects each brush to external circuit. 30-03-2024 Slide No. 27 COMMUTATOR Located at one end of armature. Consists of wedge shaped segments of hard drawn copper. Each segment is insulated from the other by thin sheet of mica. Segments held in place by steel V-rings or clamping flanges. Raised portion of each segment – Riser. Leads from armature coils are soldered into each riser. Some generators have no risers – leads soldered to slits in segment ends. 30-03-2024 Slide No. 28 COMMUTATION Commutation may be defined as: Successful reversal of current in an armature coil while under short circuit of a brush Mechanical conversion from AC to DC at the brushes of a DC machine. As a commutator segment moves in under brush – armature coil is shorted. As armature coil is under short circuit of brush – current in coil reverses direction. 30-03-2024 Slide No. 29 COMMUTATION Waveform of current in an armature coil with linear commutation Armature coil shorted Current in coils being commutated should reverse linearly with time, as shown. If reversal is not complete by time coil leaves the brush – severe sparking results. Good commutation requires coil being commutated when in neutral flux position. Brush assemblies adjusted to ensure correct (neutral) positioning for commutation. 30-03-2024 Slide No. 30 COMMUTATION Commutation occurs simultaneously in 2 coils briefly short-circuited by brushes. Brushes positioned on commutator so each coil is short-circuited in neutral plane. No voltage generated in coil at that time – therefore, no sparking occurs. Sparking between brushes and commutator is an indication of improper commutation. Improper brush placement is main cause of improper commutation. 30-03-2024 Slide No. 31 ARMATURE Consists of: Armature coils Commutator Other associated parts Mounted on shaft which rotates in bearings located in generator’s end frames. Most commonly used winding is 2-layer winding: Number of coils is equal to number of armature slots. Coil span is made equal to 180 electrical degrees. 30-03-2024 Slide No. 32 ARMATURE TWO-LAYER WINDING Coils placed in slots of armature core. NO electrical connection between coils and core. Coils typically held in slots by wedges. Coil ends brought out to individual segments of commutator. Wound with either lap winding or wave winding. 30-03-2024 Slide No. 33 ARMATURE REACTION Magnetic fields are perpendicular to the conductor From previous – known that all current-carrying conductors produce magnetic fields. Magnetic field produced by current in armature of a DC generator affects flux pattern. This distorts main field – causes a shift in neutral plane – affects commutation. Change in neutral plane and reaction of magnetic field is called armature reaction. For proper commutation – coil short-circuited by brushes must be in neutral plane. 30-03-2024 Slide No. 34 ARMATURE REACTION View A shows field poles and main magnetic field. Simple 2-pole DC generator 30-03-2024 Slide No. 35 ARMATURE REACTION Field generated around each side of coil is shown in view B. This field increases in strength for each wire in armature coil. Sets up a magnetic field almost perpendicular to main field. Simple 2-pole DC generator 30-03-2024 Slide No. 36 ARMATURE REACTION View C shows how armature field distorts the main field. Neutral plane is shifted in the direction of rotation. If brushes remain in old neutral plane – will be shorting coils with voltage in them. Consequently, there will be arcing between brushes and commutator. To prevent arcing, the brushes must be shifted to the new neutral plane. Simple 2-pole DC generator 30-03-2024 Slide No. 37 ARMATURE REACTION IN GENERATOR WITH FIELD WINDINGS Only field windings excited – Armature NOT connected – Neutral plane unaffected. 30-03-2024 Slide No. 38 ARMATURE REACTION IN GENERATOR WITH FIELD WINDINGS NO field excitation – ‘armature current’ effect shown – Neutral plane unaffected. 30-03-2024 Slide No. 39 ARMATURE REACTION IN GENERATOR WITH FIELD WINDINGS Field excited and armature connected: Neutral plane shifts in ‘direction of rotation’ 2 opposing fields produce distortion in main field Shift of ‘neutral plane’ also shifts the ideal plane of commutation Amount of distortion will vary with load placed on generator 30-03-2024 Slide No. 40 ARMATURE REACTION Shifting brushes to advanced position (new neutral plane) does not completely solve problems of armature reaction. Effect of armature reaction varies with the load current. Therefore, each time load current varies – neutral plane shifts. This means brush position needs to be changed each time load current varies. Shifting brush position for variations is not practiced (except in small generators). In larger generators, other means are taken to eliminate armature reaction. 30-03-2024 Slide No. 41 ARMATURE REACTION 30-03-2024 Slide No. 42 COMPENSATING WINDINGS AND INTERPOLES Compensating windings and/or interpoles are used to eliminate armature reaction. Compensating windings – series of coils embedded in slots in pole faces Connected in series with armature Produce a magnetic field, which varies directly with armature current Wound to produce a field that opposes magnetic field of armature Tend to cancel the effects of the armature magnetic field Neutral plane will remain stationary and in original position for all load values. Once 30-03-2024 brushes have been set correctly, they do not have to be moved again. Slide No. 43 DC GENERATOR INTERPOLES Direction of Rotation (dor) Another way to reduce effects of armature reaction is by using interpoles. Interpoles – small auxiliary poles placed between main field poles. Have a few turns of large wire and are connected in series with armature Wound and placed to have same polarity as main pole ahead of it (dor) Field generated produces same effect as compensating winding This field, in effect, cancels armature reaction for all values of load current. Causes a shift in neutral plane opposite to shift caused by armature reaction. Amount of shift will equal shift caused by armature reaction. 30-03-2024 Slide No. 44 MOTOR REACTION IN A GENERATOR When generator delivers current to load, armature current creates a magnetic force that opposes the rotation of the armature – this is called motor reaction. When current flows through conductor – sets up lines of flux around conductor. Interaction between conductor field and main field weakens field above conductor. Strengthens field below conductor – thus, an upward reaction force is produced. If current in conductor increases (more load) – motor reaction force increases. Therefore, more mechanical force must be applied to keep conductor moving. 30-03-2024 Slide No. 45 MOTOR REACTION IN A GENERATOR With NO armature current, there is NO magnetic (motor) reaction. As armature current increases – motor reaction increases. Actual force in a generator is multiplied by number of conductors in armature. Driving force required to maintain speed must increase to overcome motor reaction. Device that provides turning force to armature is called the prime mover: May be electric motor, gas turbine engine, piston engine, etc. 30-03-2024 Slide No. 46 ARMATURE LOSSES In DC generators, as in most electrical devices, forces act to decrease efficiency. These forces, as they affect the armature, are considered as losses: Copper loss in the winding Eddy current loss in the core Hysteresis loss (a sort of magnetic friction) 30-03-2024 Slide No. 47 COPPER LOSSES Power lost in form of heat in generator armature winding is known as copper loss. Heat is generated any time current flows in a conductor. Copper loss is an I2R loss – increases as current increases. Amount of heat generated is also proportional to resistance of coil conductor. Resistance of conductor varies directly with length and inversely with cross- section. Minimised 30-03-2024 in armature windings by using large diameter wire. Slide No. 48 EDDY CURRENT LOSSES Core of a generator armature is made from soft iron. Soft iron – conducting material with desirable magnetic characteristics. Any conductor will have currents induced in it when rotated in a magnetic field. These currents induced in generator armature core are called eddy currents. Power dissipated in form of heat, as a result of eddy currents, is considered a loss. Eddy currents affected by resistance of material in which currents flow. Resistance of any material is inversely proportional to its cross-sectional area. 30-03-2024 Slide No. 49 EDDY CURRENT LOSSES Solid core – larger cross-sectional area – lower resistance – greater eddy currents. Laminated core – smaller pieces joined and insulated from each other – laminations. Sum of eddy currents in laminated core – considerably less than in solid core. Laminations are insulated from each other by a thin coat of lacquer or oxidation. Insulation value need not be high because voltages induced are very small. Most generators use armatures with laminated cores to reduce eddy current losses. 30-03-2024 Slide No. 50 HYSTERESIS LOSSES Hysteresis loss – heat loss caused by magnetic properties of armature. Armature core magnetic particles tend to line up with magnetic field. When armature core is rotating, its magnetic field keeps changing direction. Continuous movement of magnetic particles produces molecular friction. This produces heat – transmitted to armature windings – armature resistances rises. To compensate for hysteresis losses, heat-treated silicon steel laminations are used. After steel has been formed to shape, laminations are heated and allowed to cool. This annealing process reduces hysteresis loss to a low value. 30-03-2024 Slide No. 51 TERMINAL VOLTAGE DC generator output voltage is dependent on 3 factors: Number of conductor loops in series in the armature Armature speed Magnetic field strength In order to change generator output, one of these factors must be varied. Number of conductors in armature cannot be changed. Usually impractical to change speed at which armature rotates. Strength of magnetic field can be changed quite easily by varying field current. 30-03-2024 Slide No. 52 FIELD EXCITATION When a DC voltage is applied to the field windings, current flows through the windings and sets up a steady magnetic field - called Field Excitation: Separately excited generators - supplied by an independent current source. 30-03-2024 Slide No. 53 FIELD EXCITATION When a DC voltage is applied to the field windings, current flows through the windings and sets up a steady magnetic field - called Field Excitation: Separately excited generators - supplied by an independent current source. Self-excited generator - supplied directly from the output of the generator. 30-03-2024 Slide No. 54 FIELD EXCITATION When a DC voltage is applied to the field windings, current flows through the windings and sets up a steady magnetic field - called Field Excitation: Separately excited generators - supplied by an independent current source. Self-excited generator - supplied directly from the output of the generator. Possible only if field pole pieces have retained some residual magnetism 30-03-2024 Slide No. 55 DC MOTORS 30-03-2024 Slide No. 56 INDUCING A FORCE ON A CONDUCTOR There are 2 conditions which are necessary to produce a force on a conductor: Conductor must be carrying current Conductor must be within a magnetic field When these 2 conditions exist, a force will be applied to conductor. Force will attempt to move conductor in direction perpendicular to magnetic field. This is the basic theory by which all DC motors operate. 30-03-2024 Slide No. 57 PRINCIPLES OF OPERATION Current-Carrying Conductor in a Magnetic Field Every current-carrying conductor has a magnetic field around it. Direction of magnetic field found using left-hand rule for current-carrying conductors. Where conductor field opposes main field – weak point. Where conductor field supports main field – strong point. Strong point overcomes weak point – conductor moves in direction of weak point. 30-03-2024 Slide No. 58 PRINCIPLES OF OPERATION Right-hand Rule for Motors Principle of operation of a DC motor: A current-carrying conductor placed in a magnetic field, perpendicular to lines of flux, tends to move in a direction perpendicular to magnetic lines of flux. Resultant conductor movement – depends on directions of magnetic fields. Best explained by using RIGHT-HAND RULE FOR MOTORS. 30-03-2024 Slide No. 59 BASIC SINGLE COIL DC MOTOR Conventional current flow A current-carrying conductor placed in a magnetic field, perpendicular to lines of flux, tends to move in a direction perpendicular to magnetic lines of flux. 30-03-2024 Slide No. 60 BASIC SINGLE COIL DC MOTOR A current-carrying conductor placed in a magnetic field, perpendicular to lines of flux, tends to move in a direction perpendicular to magnetic lines of flux. 30-03-2024 Slide No. 61 BASIC SINGLE COIL DC MOTOR A current-carrying conductor placed in a magnetic field, perpendicular to lines of flux, tends to move in a direction perpendicular to magnetic lines of flux. 30-03-2024 Slide No. 62 BASIC SINGLE COIL DC MOTOR 30-03-2024 Slide No. 63 EXERCISE Electron flow What is the direction of rotation? 30-03-2024 Slide No. 64 TORQUE Torque – defined as that force which tends to produce and maintain rotation. Function of torque in a DC motor – to provide mechanical output. Sum of the forces (lbs) multiplied by radius of armature (ft) is equal to torque (lb-ft). Combined action of magnetic fields develops force on conductor of armature. Force – directly proportional to strength of main field and field around conductor. Field strength around each armature conductor depends on amount of current flow. 30-03-2024 Slide No. 65 TORQUE IN A DC MOTOR Conductors parallel to magnetic field (Neutral Position) – NO torque is produced. Conductors at right angles to magnetic field – maximum torque is produced. Positions in-between – varying levels of torque. ‘Direction of force’ is always 90 º to magnetic field. 30-03-2024 Slide No. 66 TORQUE VARIATION IN DC MOTOR Commutator – stops torque from reversing each time coil moves through neutral plane. Neutral plane – perpendicular to magnetic field. Commutator (and brushes) – used to reverse current at point where torque is zero. 30-03-2024 Slide No. 67 COUNTER emf Any time a conductor is moved in a magnetic field, an emf is produced. When this occurs in DC motor – emf is called counter emf. This is because emf produced in motor opposes emf supplied to armature. Counter emf – directly proportional to speed of armature and field strength. That is, counter emf increases if armature speed or field strength increases. Greater the cemf – greater the resistance to current flow delivered to armature. 30-03-2024 Slide No. 68 DC MOTOR TYPES In all important aspects – DC motors are identical to DC generators. Main difference between DC motor and DC generator is what must be controlled. Generator: must control what comes out – Motor: must control what goes in. As with generators, major classes of DC motors are: Series wound Shunt wound Compound wound Separately excited / Permanent magnet 30-03-2024 Slide No. 69 DC MOTOR TYPES These types of motors differ only in the connection of field circuits. Armatures, commutators, etc. – nearly identical with each other and with generators. All 4 major classes of motors are widely used. Contrast to generators – compound wound type is nearly always used. 30-03-2024 Slide No. 70 SERIES MOTOR Field is connected in series with armature. Field – few turns of large wire – must carry full armature current. Develops a very large amount of torque (turning force) from a standstill. Used for small electric appliances, portable electric tools, cranes, winches, etc. Speed varies widely between no-load and full-load. Cannot be used for constant speed under conditions of varying load. 30-03-2024 Slide No. 71 SERIES MOTOR Some load must always be connected to a series motor before turning it on. A major disadvantage of series motor: Speed with no load connected increases to point where damage may occur Either bearings are damaged or windings fly out of armature slots Danger to both equipment and personnel No load precaution is primarily for large motors. Small motors (portable power tools) have enough internal friction to load themselves. Series motors – can be operated by either AC or DC. 30-03-2024 Slide No. 72 SERIES MOTOR SPEED CONTROL A – For below normal speed control – rheostat connected in parallel with armature. Motor speed is increased by decreasing current through field. B – For normal speed control – rheostat connected in series with motor field. Motor speed is increased by increasing voltage across entire motor. C – For above normal speed control – rheostat connected in parallel with series field. Part of voltage bypasses series field causing motor to speed up. 30-03-2024 Slide No. 73 SHUNT MOTOR Used where uniform speed (regardless of load) is wanted. Has reasonably good starting torque but is not suited for starting very heavy loads. Used where: Starting load is not too heavy (as in blowers) Mechanical load is not applied until motor has come up to speed Essentially a constant speed machine. Speed does not ordinarily change more than 10 to 15 percent within load limits. 30-03-2024 Slide No. 74 SHUNT MOTOR Field windings connected in parallel (shunt) with armature windings. Speed remains relatively constant even under changing load conditions. Reason for this – field flux remains constant – connected directly across input. Constant voltage across field makes it independent of variations in armature circuit. If load on motor increases – motor tends to slow down. Upon slowing – cemf in armature decreases – decreases opposition to current flow. Armature current increases – causes motor to speed up – Original speed maintained. Instantaneous 30-03-2024 tendency to change rather than a large fluctuation in speed. Slide No. 75 SHUNT MOTOR SPEED CONTROL Magnetic field is necessary to maintain an adequate Cemf in armature. As long as Cemf is maintained – motor operates at its rated speed. DC shunt motors can control speed above a certain operating (or base) point. Above Normal Speed Control Rheostat in series with shunt field determines amount of current through field. If resistance increases– less current to field – magnetic field strength reduces. With reduction in magnetic field – reduction in armature Cemf. When Cemf is reduced – armature receives more current. More current in armature – greater torque developed – motor speed increases. 30-03-2024 Slide No. 76 SHUNT MOTOR SPEED CONTROL Below Normal Speed Control To reduce speed of shunt or any DC motor – reduce current to armature. A rheostat in series with armature will vary current to armature. As armature resistance is increased – current to armature is decreased. Decrease in armature current decreases torque and armature speed. Control of armature circuit in this manner does not substantially affect cemf. 30-03-2024 Slide No. 77 COMPOUND MOTOR 2 field windings – shunt field (parallel) and series field (series with armature): Shunt field gives constant speed advantage of a regular shunt motor Series field gives advantage of being able to develop large torque Compound motor has both shunt and series motor characteristics. Long shunt – shunt field connected in parallel with both series field and armature. Short shunt – shunt field connected in parallel only with armature. 30-03-2024 Slide No. 78 CUMULATIVE COMPOUND MOTOR In most cases, series winding is connected so its magnetic field aids shunt winding. Current flow through both series field and shunt field is in same direction. Both fields produce same magnetic field and aid each other. Motors of this type are called cumulative compound motors. When a load is applied: Speed decreases more rapidly than shunt motor, but less rapidly than series motor Used when reasonable uniform speed and good starting torque is required. 30-03-2024 Slide No. 79 DIFFERENTIAL COMPOUND MOTOR Differential compound motor is used only for low power work. Series winding's field is connected to oppose shunt winding's magnetic field. Maintains even better constant speed, within its load limit, than the shunt motor. Very poor starting torque and is unable to handle serious overloads. Speed control in compound motors is typically as per shunt motors (field control). 30-03-2024 Slide No. 80 SEPARATELY EXCITED MOTOR Individual armature circuit and an individual field circuit. DC power source (that is not armature-connected) supplies power to field poles. Armature rheostat is for below normal speed control (as per all DC motors). Field rheostat is for above normal speed control (as per shunt motor). Used to control speed and torque of motor from "no load" to "full load“. Used for its high torque capability at low speed. Used in some servo systems for control of speed and/or position. 30-03-2024 Slide No. 81 D.C. MOTOR CHARACTERISTICS 30-03-2024 Slide No. 82 REVERSING MOTOR DIRECTION + Direction of rotation of a DC motor is reversible. Achieved by reversing direction of current flow in either armature or field windings. This reverses magnetism of either armature or magnetic field. If wires connecting power to motor are reversed – DOR remains unchanged. 1 method employs 2 field windings wound in opposite directions on same pole. This type of motor is called a split field motor. 30-03-2024 Slide No. 83 SPLIT FIELD DC MOTOR Series motor with a split field winding. Single pole, double throw switch directs current through either of the 2 windings. When switch placed in lower position – current flows through lower field winding. This creates North pole at lower pole piece and South pole at upper pole piece. Switch to upper position – magnetism of field reversed – armature rotation opposite. 30-03-2024 Slide No. 84 REVERSING MOTOR DIRECTION Another method of direction reversal is the switch method. Uses a double pole, double throw switch. Changes direction of current flow in either armature or field. In above example: Current direction may be reversed through field but not through armature Switch to "up" position – North pole at right side field winding of motor Switch to "down" position – polarity reversed – armature rotates in 30-03-2024 opposite direction Slide No. 85 ARMATURE REACTION IN DC MOTORS Reasons for armature reaction – same for DC motors as for DC generators. Figure reiterates distorting effect that armature field has on flux between pole pieces. In DC motor – 1 major difference to armature reaction in a DC generator? Effect has shifted neutral plane backward, opposite direction of rotation. Shift of ‘neutral plane’ also shifts ideal plane of commutation – must shift brushes. Shift is opposite DOR – proper location is reached when there is no sparking. Methods of compensating for armature reaction – basically same as generators. 30-03-2024 Slide No. 86 CANCELLING ARMATURE REACTION Shifting brushes reduces sparking, but it also makes field less effective. Compensating windings and interpoles cancel armature reaction in DC motors. Cancelling armature reaction eliminates need to shift brushes in the first place. Compensating windings and interpoles are as important in motors as in generators. Compensating windings are same in motors as in generators. Compensating windings are also relatively expensive. Therefore, most large DC motors depend on interpoles to correct armature reaction. 30-03-2024 Slide No. 87 DC MOTOR INTERPOLES Interpoles are different in DC motors as compared to DC generators. In a generator – interpole has same polarity as the main pole ahead of it in DOR. In a motor – interpole has same polarity as the main pole following it in DOR. As load varies, interpole flux varies, and commutation is automatically corrected. It is not necessary to shift brushes when there is an increase or decrease in load. 30-03-2024 Slide No. 88 STARTER GENERATORS Combined 2 functions: Starter Generator Provides: Electric starter Power generation Weight-savings NO clutch assembly – always spins. Coupled to engine via a quill shaft. 30-03-2024 Slide No. 89 STARTER GENERATORS Many small turbine engines are equipped with starter generators. Provides appreciable weight savings – both starters and generators are very heavy. Typical starter generator – consists of 2 sets of field windings and 1 armature winding. 1 field winding (start only) and armature wound with larger conductors. In starter mode – high current flows through both sets of field windings and armature. In generator mode – current only flows through shunt winding (finer wire). Current through shunt winding produces field that induces voltage into armature. 30-03-2024 Slide No. 90 CONCLUSION Now that you have completed this topic, you should be able to: 3.12.1 Describe basic motor and generator theory. 3.12.2 Identify components of a DC generator and describe their construction and purpose. 3.12.3 Describe the operation of DC generators and factors affecting: Output Direction of current flow 3.12.4 Describe the operation of DC motors and factors affecting: Output power Torque Speed Direction of rotation 3.12.5 Describe the operation and features of the following DC motors: Series wound Shunt wound Compound 3.12.6 Describe the construction and operation of starter generators. 30-03-2024 Slide No. 91 This concludes: Module 3: Electrical Fundamentals Topic 3.12: DC Motor/Generator Theory