AUBIO 111 Midterm 2 Review PDF
Document Details
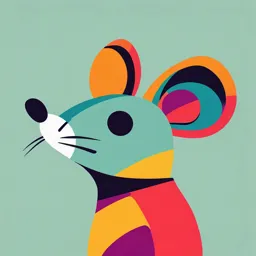
Uploaded by SustainablePhiladelphia
University of Alberta
2024
Kevin Yoon, PhD
Tags
Summary
This is a review of functional biology concepts, suitable for a midterm 2 preparation at the University of Alberta Fall 2024. The document contains different topics that are relevant to the course, like metabolism, enzymes, and cellular respiration.
Full Transcript
AUBIO 111: Functional Biology Dr. Kevin Yoon, PhD [email protected] Fall 2024 - A01 Midterm 2 review Format Monday, Nov 25 – 11 am-12 pm (normal lecture session) In-person Physical copies (not electronic) Bring your OneCard Or know your student ID number Format 60-minute sessio...
AUBIO 111: Functional Biology Dr. Kevin Yoon, PhD [email protected] Fall 2024 - A01 Midterm 2 review Format Monday, Nov 25 – 11 am-12 pm (normal lecture session) In-person Physical copies (not electronic) Bring your OneCard Or know your student ID number Format 60-minute session 40 total marks (rate of 1 min 30 sec per mark) 26 marks associated with multiple choice questions 14 marks associated with short answer questions Blue or black pens recommended; if you use pencil, you won't be able to appeal Calculators are not necessary Content Disclaimer: What follows is not meant to be a definitive review of concepts – It is meant to be a brief reminder of material that we have covered Chapter 8 - An introduction to metabolism An organism’s metabolism transforms matter and energy, subject to the laws of thermodynamics The free-energy change of a reaction tells us whether or not the reaction occurs spontaneously ATP powers cellular work by coupling exergonic reactions to endergonic reactions Enzymes speed up metabolic reactions by lowering energy barriers Regulation of enzyme activity helps control metabolism Metabolic pathways Metabolic pathways begin with a specific molecule (substrate or reactant) and end with a product Each step is catalyzed by a specific enzyme Regulation of key steps in pathway is key – why? Metabolic pathways Cells must be able to harvest energy and store it in forms relevant for cellular activities Cells must also be able to synthesize new components for various functional roles Generally, metabolic pathways can be divided into two types: 1. Catabolism – releases energy 2. Anabolism – consumes energy BMG Labtech Often interconnected Energy Energy is the capacity to cause change Energy exists in various forms, some of which can perform work 1. Kinetic energy - associated with motion 2. Heat (thermal energy) – kinetic energy associated with random movement of atoms or molecules 3. Potential energy - energy that matter possesses because of its location or structure 4. Chemical energy - potential energy available for release in a chemical reaction Energy can be converted (transformed) from one form to another Laws of Thermodynamics Thermodynamics is the study of energy transformations Isolated system - unable to exchange energy or matter with its surroundings Closed system – energy can be transferred, but not matter Open system - energy and matter can be transferred between the system and its surroundings Organisms are open systems Traditionally, there are 3 laws of thermodynamics (now there’s a zeroth) We will focus on the First and Second laws The First Law of Thermodynamics According to the first law of thermodynamics, the amount of energy in the universe is constant Energy can be transferred and transformed, but it cannot be created or destroyed The first law is also called principle of conservation of energy The Second Law of Thermodynamics During every energy transfer or transformation, some energy is unusable, and often lost as heat Second law of thermodynamics Every energy transfer or transformation increases entropy (disorder) of universe Think about direction of spontaneous processes (i.e., heat transfer, diffusion) Sum of entropies within a thermodynamic system never decreases ∆Stotal = ∆Ssystem + ∆Ssurroundings Free-energy change (∆G) Which reactions occur spontaneously, and which require input of energy? Need to determine energy changes that occur in chemical reactions Living system’s free energy is energy that can do work when temperature and pressure are uniform, as in a living cell Determines if a given reaction is spontaneous or not Change in free energy (∆G) is related to change in total energy (also called enthalpy) (∆H), change in entropy (∆S), and temperature in Kelvin (T): ∆G = ∆H – T∆S Free-energy change (∆G) ∆G = ∆H – T∆S Processes in which ∆G < 0 (negative value) are spontaneous (exergonic; favourable) ∆H < T∆S Could be that energy is lost due to heat loss (∆H < 0) or that there is an increase in entropy (∆S > 0) Processes in which ∆G > 0 (positive value) are non-spontaneous (endergonic; unfavourable) ∆H > T∆S Could be that energy is gained due to heat absorption (∆H > 0) or that there is a decrease in entropy (∆S < 0) ∆G = 0 represents a system in equilibrium Free-energy, stability, and work Free energy - measure of a system’s instability, its tendency to change to a more stable state Equilibrium - a state of maximum stability A process is spontaneous and can perform work only when it is moving toward equilibrium During a spontaneous change, free energy decreases and stability of a system increases Spontaneous processes can be harnessed to perform work Free-energy, stability, and work Exergonic and endergonic reactions Based on their free energy changes, all chemical reactions can be classified as exergonic or endergonic Exergonic reaction - net release of free energy; spontaneous (∆G < 0) Endergonic reaction - absorbs free energy from its surroundings; nonspontaneous (∆G > 0) AT P powers cellular work Three kinds of cellular work Chemical Transport Mechanical To do work, cells manage energy resources by energy coupling, Use of an exergonic process to drive an endergonic one Most cellular energy coupling is mediated by ATP (adenosine triphosphate) Think of it as the cell’s energy shuttle Highly stable, covalent bond ATP hydrolysis Bonds between the phosphate groups of ATP can be broken by hydrolysis Breakage of terminal phosphate bond of ATP releases energy Note: Release of energy comes from the chemical change to a state of lower energy, not from the phosphate bonds themselves Loss of the covalent bond means products have less free energy (∆G < 0) How ATP hydrolysis powers cellular work Hydrolysis of ATP results in cellular work (see two slides back) In the cell, the energy from the exergonic reaction of ATP hydrolysis can be used to drive an endergonic reaction Overall, the coupled reactions are exergonic (∆GNet < 0) Activation energy barrier Every chemical reaction between molecules involves bond breaking and bond forming Initial energy needed to start a chemical reaction is called free energy of activation, or activation energy (EA) Often supplied in form of thermal energy that reactant molecules absorb from their surroundings Enzymes Catalyst – chemical agent that speeds up a reaction without being consumed in the reaction Enzyme – catalyst protein Helps the reaction overcome the activation energy barrier (Enzyme) How Enzymes Speed up Reactions Enzymes catalyze reactions by lowering EA barrier Enzymes do not affect change in free energy (∆G) Rather, enzymes speed up reactions that would occur eventually Catalytic Cycle of an Enzyme Enzyme inhibitors Competitive inhibitors Bind to the active site of an enzyme Examples of Compete with substrate inhibitors: Noncompetitive inhibitors - Toxins - Poisons Bind to an enzyme at a separate - Pesticides site from the active site - Antibiotics Causing a conformational change in the enzyme that makes the active site less effective Allosteric regulation Most allosterically regulated enzymes contain multiple polypeptide subunits Each enzyme has active and inactive forms Binding of an activator stabilizes the active form of an enzyme Binding of an inhibitor stabilizes the inactive form of an enzyme Non-covalent binding Reversible process Cooperativity is a form of allosteric regulation that can amplify enzyme activity Change in one subunit results in change to another subunit that affects its binding affinity If increase affinity, positive cooperativity If decrease affinity, negative cooperativity Feedback loops Feedback loops End product of reaction affects the reaction Can be negative or positive Feedback inhibition (negative) Creation of the end product results in stoppage of reaction Consider why this is needed Chapter 9 – Cellular respiration and fermentation Catabolic pathways yield energy by oxidizing organic fuels Glycolysis harvests chemical energy by oxidizing glucose to pyruvate After pyruvate is oxidized, the citric acid cycle completes the energy-yielding oxidation of organic molecules During oxidative phosphorylation, chemiosmosis couples electron transport to ATP synthesis Fermentation and anaerobic respiration enable cells to produce ATP without the use of oxygen Glycolysis and the citric acid cycle connect to many other metabolic pathways Catabolic pathways and ATP production Breakdown of organic molecules is exergonic (free energy is released) Aerobic respiration consumes organic molecules and O2 and yields YES Oxygen AT P Anaerobic respiration is similar to aerobic respiration but consumes compounds other than O2 NO Oxygen Fermentation is partial degradation of sugars that occurs without O2 Catabolic pathways and ATP production Cellular respiration Refers to both aerobic and anaerobic respiration, but is often used to specifically refer to aerobic respiration Carbohydrates, fats, and proteins from our diet are all consumed as fuel; but cellular respiration is usually demonstrated with glucose: C6H12O6 + 6 O2 → 6 CO2 + 6 H2O + Energy (ATP + heat) Redox reactions Electron Reducing agent = electron donor Oxidizing agent = electron acceptor A B Some redox reactions do not completely transfer electrons but change the relative share of electrons Reducing agent Oxidizing agent in covalent bonds Essentially, not all redox reactions result in A loses electrons, while B gains electrons +ve and -ve charge on respective agents - A is oxidized by B; A becomes more positive - B is reduced by A; B becomes more negative Principles of redox reactions O2 is highly electronegative → potent oxidizing agent Generally, abundance of H atoms correlates with higher energy harvest As glucose is oxidized, energy state of e - lowers as H atoms are transferred to O2 Liberated energy is used to synthesize ATP Substrate-Level Phosphorylation In addition to oxidative respiration, a small amount of AT P is formed during glycolysis and the citric acid cycle by substrate- level phosphorylation Overview of cellular respiration Overview of cellular respiration Glycolysis Breaks down glucose into two molecules of pyruvate Glycolysis summary Input Output Glycolysis Glucose 2 Pyruvate + 2 ATP + 2 NADH So… now what? What happens to the pyruvate? Overview of cellular respiration Pyruvate oxidation and the citric acid cycle Completes breakdown of glucose Keep track of ATP, NADH, FADH2 produced! Overview of cellular respiration Oxidative phosphorylation Accounts for most of ATP synthesis Chemiosmosis Chemiosmosis - the use of energy in a H+ gradient to drive cellular work The energy released via ETC is used to pump H+ from the mitochondrial matrix to the intermembrane space Generates a concentration gradient H+ then flow down their concentration gradient back across the inner membrane, through ATP synthase ATP synthase uses the exergonic flow of H+ to drive phosphorylation of ADP to form ATP Proton-motive force So… how much energy is produced? 1 NADH = 2.5 ATP 1 FADH2 = 1.5 ATP But there’s an exception! Producing energy without O2 Anaerobic respiration uses an electron transport chain with a final electron acceptor other than O2 i.e., sulphate (SO42-) – highly electronegative, can function similarly to O2 to facilitate electron movement Fermentation enables glycolysis to continue to make AT P by substrate-level phosphorylation in the absence of oxidative phosphorylation driven by the E T C Not as efficient as ETC-mediated ATP production Alcohol fermentation Alcohol fermentation by yeast is used in brewing, winemaking, and baking Pyruvate is converted to ethanol in two steps Releases CO2, and regenerates NAD+ to be used in glycolysis Lactic acid fermentation Lactic acid fermentation by some fungi and bacteria is used to make cheese and yogurt Pyruvate is reduced by NADH to yield NAD+ Forms lactate as an end product without release of CO2 Lactic acid build-up in muscles due to this process When O2 is depleted, glucose is broken down via anaerobic pathways to meet energy demands Chapter 10 - Photosynthesis Photosynthesis converts light energy to the chemical energy of food The light reactions convert solar energy to the chemical energy of ATP and NAD PH The Calvin cycle uses the chemical energy of ATP and NAD PH to reduce CO2 to sugar Alternative mechanisms of carbon fixation have evolved in hot, arid climates Location of photosynthesis For plants, leaves are the major locations of photosynthesis Large, flat areas for maximal sunlight exposure Full of mesophyll cells Located between two epidermal (“skin”) cell layers Chlorophyll found here Internal thylakoid membranes contain chlorophyll (green colour) and form membranous sacs Thylakoids are stacked to form grana Internal soluble compartment called thylakoid lumen Remember that we are only seeing the end result There were a lot of steps here Aerobic respiration: Photosynthesis: So… what are the steps here? Two stages of photosynthesis – a preview Conversion of energy followed by fixation of carbon The light reactions (in the thylakoids) Split H2O Release O2 Reduce NADP+ to NADPH Generate ATP from ADP by photophosphorylation The Calvin cycle fixes carbon, incorporating CO2 into organic molecules such as sugar Energy from the ATP and NADPH made during the light reactions is used to drive the Calvin cycle Photosystem System of chlorophyll + protein subunits Consists of a reaction-centre complex surrounded by light- harvesting complexes The light-harvesting complexes (pigments bound to proteins) transfer the energy of photons to the reaction centre Linear electron flow Cyclic electron flow PSII is not involved in cyclic electron flow; electrons cycle back from Fd to PSI No oxygen is released Uses only PSI and produces ATP, but not NADPH This satisfies the Calvin cycle’s need for more ATP than NADPH The Calvin cycle Carbon enters as CO2 and leaves as glyceraldehyde 3- phosphate (G3P) For the net synthesis of 1 G3P (a 3-carbon molecule), the cycle must “turn” three times, thus fixing three CO2 The Calvin cycle Uses the chemical energy of ATP and NADPH to reduce CO2 to sugar The Calvin cycle has three phases: 1. Carbon fixation (catalyzed by Rubisco) 2. Reduction 3. Regeneration of RuBP (the molecule that accepts CO2) Photorespiration vs. Calvin cycle In most plants, fixation of CO2 by Rubisco generates a 3-carbon compound (therefore called C3 plants) During photorespiration, Rubisco uses O2 instead of CO2, producing a two-carbon compound Consumes O2 and organic molecules, Wiki and ultimately results in the release of CO2, but without producing ATP or sugar In one sense, the plant cell is trying to create its own CO2 to facilitate Calvin cycle Chapter 11 – Cell communication External signals are converted to responses with the cell Reception: A signaling molecule binds to a receptor protein, causing it to change shape Transduction: Cascades of molecular interactions relay signals from receptors to target molecules in the cell Response: Cell signaling leads to regulation of transcription or cytoplasmic activities Apoptosis is programmed cell death and integrates multiple cell-signaling pathways Ability of a cell to respond depends on the presence of specific receptors to the signal, regardless of type of signaling To be effective, a signal must be recognized as a signal “Local” “Long-distance” (Cell-cell recognition) (Local regulators) (Hormones) Reception – signal input Binding between signal molecule (ligand) and receptor is highly specific A conformational change in a receptor is often the initial transduction of signal Most signal receptors are plasma membrane proteins (cell-surface receptors) Types of cell-surface receptors Ligand-gated ion channel Receptor tyrosine kinase (RTK) G protein-coupled receptors (aka ion channel-coupled receptors) (aka enzyme-coupled receptors) Intracellular receptors Intracellular receptor proteins are found in the cytosol or nucleus of target cells Small or hydrophobic chemical messengers (signaling molecules) can readily cross the membrane and activate these receptors Examples: Steroid and thyroid hormones in animals Aldosterone (steroid hormone) can bind to a receptor, form an activated hormone-receptor complex, which can act as transcription factor to affect gene expression Transduction – relay of signals Usually involves multiple steps → allows amplification of signals (signal cascade) Also provides more opportunities for coordination and regulation of the cellular response Comparison of molecular switches What are the similarities and differences? Signal cascade Note that each kinase can phosphorylate multiple target molecules i.e., One active protein kinase 1 can phosphorylate multiple inactive protein kinase 2 molecules Results in signal amplification Second messengers The ligand that binds the initial receptor is a pathway’s “first messenger” Second messengers are small, nonprotein, water-soluble molecules or ions, that spread throughout a cell by diffusion Common second messengers include cyclic AMP (cAMP) and calcium ions (Ca2+) Second messengers participate in pathways initiated by GPCRs and RTKs We will examine them in the context of GPCRs cAMP Most adenyl cyclases are activated downstream of GPCRs cAMP usually activates protein kinase A, which phosphorylates various other proteins Further regulation provided by G- protein systems that inhibit adenylyl cyclase Calcium ions and inositol triphosphate (IP3) PIP2 Activated G proteins activate phospholipase C Triggers production of two signaling molecules from PIP2 Diacylglycerol (DAG) Inositol triphosphate (IP3) Leads to Ca2+ release into cytosol and activation of protein kinase C (PKC) Ligand-gated ion channel Response – signal output Ultimately, a signal transduction pathway leads to regulation of one or more cellular activities (i.e., change in cell shape, stimulate cell division) Underlying molecular response may occur in nucleus or in cytoplasm Reception Transduction Response Regulation of response A response to a signal might not necessarily be simply “on” or “off” There are four aspects of regulating a response to consider: 1. Amplification of the signal (and thus the response) 2. Specificity of the response 3. Overall efficiency of response, enhanced by scaffolding proteins 4. Termination of the signal Remember: Cells don’t just receive one signal at a time… Coordination of signals is key for proper cell response Apoptosis Programmed (controlled) cell death Cellular components are systematically broken down and digested by scavenger cells Cell death is contained Prevents enzymes from leaking out of dying cells and damaging neighbouring cells Molecular basis of apoptosis Caspases are the main proteases (enzymes that cut up proteins) that carry out apoptosis Caspase cascade Apoptosis can be triggered by external or internal signals, including: Extracellular death-signaling ligands DNA damage in the nucleus Protein misfolding in endoplasmic reticulum Avoidance of apoptotic pathways is key for cell survival But what if a cell is supposed to die? Chapter 12 – Cell cycle / Mitosis Most cell division results in genetically identical daughter cells The mitotic phase alternates with interphase in the cell cycle The eukaryotic cell cycle is regulated by a molecular control system Cancer is characterized as a disease in which cell cycle is unregulated How do cells divide? Eukaryotic cell division consists of Mitosis: the division of the genetic material Cytokinesis: the division of the cytoplasm Meiosis: specialized cell division used to create gametes; yields non-identical, haploid cells When cells divide, the genetic material must be replicated and divided Therefore, cell division is often discussed in the context of chromosomes and their movements Chromosomes Most cell division results in daughter cells with identical genetic information (DNA) as the parent cell, and as each other The exception is meiosis DNA is packaged into chromosomes Chromosomes consist of chromatin Replicated Complex of DNA and protein Condenses during cell division (Replicated) Chromosomes vs. Chromatids A replicated chromosome is composed of two sister chromatids Held together by cohesins Sister chromatids Centromere – constricted region Present in replicated chromosomes Links sister chromatids together Very important for cell division Centromere Site of microtubule attachment When the chromatids separate, each chromatid is referred to as a chromosome Replicated chromosome Chromosome Chromosome Phases of the cell cycle Cell cycle consists of: Mitotic (M) phase Mitosis (Division of genetic material) Cytokinesis (Division of cytoplasm) Interphase (cell growth and copying of chromosomes in preparation for cell division; consists of 3 subphases) G1 phase (“first gap”) S phase (“synthesis”) G2 phase (“second gap”) Interphase G1 phase: Cells exit M phase and start to grow Synthesis of proteins, production of organelles, etc. S phase: Replicates DNA in preparation for mitosis Ploidy does not change! G2 phase: Cell resumes growth, similar to G1 Mitotic (M) phase Mitosis is conventionally divided into five phases: Prophase Prometaphase Metaphase Anaphase Telophase Cytokinesis overlaps latter stages of mitosis Different mechanisms of cell cycle Mitotic spindle During prometaphase, some spindle microtubules attach to kinetochores and begin to move chromosomes Called kinetochore microtubules Kinetochores Protein complexes that form in association with the centromeres of chromosomes At metaphase, chromosomes all lined up at the metaphase plate (the midway point between the two spindle poles) Cytokinesis In animal cells, cytokinesis involves formation of a cleavage furrow Contractile ring A ring of microfilaments (actin) forms at the equator Non-muscle myosin interacts with actin to pinch, creating the cleavage furrow In plant cells, a cell plate forms during cytokinesis Vesicles form; fuse to create cell plate Cell cycle control system The sequential events of the cell cycle are directed by a distinct cell cycle control system, which is analogous to a clock Regulated by both internal and external controls The cell cycle pauses at specific checkpoints until a go-ahead signal is received The G1, G2 and M checkpoints are three of the most important and best understood Cyclins and cyclin-dependent kinases Two types of regulatory proteins involved in cell cycle control: Cyclins Cyclin-dependent kinases (Cdks) Note: many types of cyclins and Cdks exist Cyclins control Cdk activity Binding of cyclin activates Cdk Activity of Cdk is proportional to the concentration of cyclin present Questions?