Atmospheric Science by Wallace & Hobbs, 2nd Edition PDF
Document Details
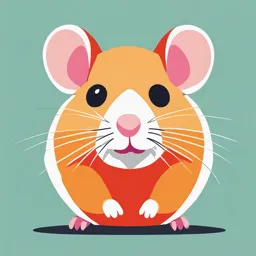
Uploaded by EngagingRevelation325
Gauhati University
2006
John M. Wallace, Peter V. Hobbs
Tags
Summary
This book, "Atmospheric Science" by Wallace and Hobbs, is a second edition that offers an introductory survey of atmospheric science. It covers fundamental concepts such as atmospheric thermodynamics, water vapor in the atmosphere, and climate, and delves into the dynamics of the Earth's atmosphere. It's a helpful resource for students and researchers interested in the field.
Full Transcript
P732951-Prelims.qxd 12/16/05 10:54 AM Page i Atmospheric Science Second Edition P732951-Prelims.qxd 12/16/05 10:54 AM Page ii This is Volume 92 in the INTERNATION...
P732951-Prelims.qxd 12/16/05 10:54 AM Page i Atmospheric Science Second Edition P732951-Prelims.qxd 12/16/05 10:54 AM Page ii This is Volume 92 in the INTERNATIONAL GEOPHYSICS SERIES A series of monographs and textbooks Edited by RENATA DMOWSKA, DENNIS HARTMANN, and H. THOMAS ROSSBY A complete list of books in this series appears at the end of this volume. P732951-Prelims.qxd 12/16/05 10:54 AM Page iii Atmospheric Science An Introductory Survey Second Edition John M. Wallace Peter V. Hobbs University of Washington AMSTERDAM BOSTON HEIDELBERG LONDON NEW YORK OXFORD PARIS SAN DIEGO SAN FRANCISCO SINGAPORE SYDNEY TOKYO Academic Press is an imprint of Elsevier P732951-Prelims.qxd 12/16/05 10:54 AM Page iv Acquisitions Editor: Jennifer Helé Project Manager: Jeff Freeland Marketing Manager: Linda Beattie Marketing Coordinator: Francine Ribeau Cover Art Direction: Cate Rickard Barr Text Design: Julio Esperas Composition: Integra Software Services Private Limited Cover Printer: Transcontinental Printing Interior Printer: Transcontinental Printing Academic Press is an imprint of Elsevier 30 Corporate Drive, Suite 400, Burlington, MA 01803, USA 525 B Street, Suite 1900, San Diego, California 92101-4495, USA 84 Theobald’s Road, London WC1X 8RR, UK This book is printed on acid-free paper. Copyright © 2006, Elsevier Inc. All rights reserved. No part of this publication may be reproduced or transmitted in any form or by any means, electronic or mechanical, including photocopy, recording, or any information storage and retrieval system, without permission in writing from the publisher. Permissions may be sought directly from Elsevier’s Science & Technology Rights Department in Oxford, UK: phone: (+44) 1865 843830, fax: (+44) 1865 853333, E-mail: [email protected]. You may also complete your request on-line via the Elsevier homepage (http:///elsevier.com), by selecting “Support & Contact” then “Copyright and Permission” and then “Obtaining Permissions.” Cover Photo Credits: Noboru Nakamura’s drawing, bearing the Latin inscription “Here watch the powerful machine of nature, formed by the air and other elements”, was inspired by mankind’s long-standing fascination with weather and climate. Some of the products of this machine are represented by the other images (left to right): annual mean sea surface temperature (courtesy of Todd Mitchell), a single cell thunderstorm cell over a tropical Pacific atoll (courtesy of Art Rangno), a supercell thunderstorm over Kansas (courtesy of Chris Kridler), and Antarctic sea ice (courtesy of Miles McPhee). Library of Congress Cataloging-in-Publication Data Wallace, John M. (John Michael), 1940– Atmospheric science : an introductory survey / John M. Wallace, Peter V. Hobbs.—2nd ed. p. cm. ISBN 0-12-732951-X 1. Atmosphere—Textbooks. 2. Atmospheric physics—Textbooks. 3. Atmospheric chemistry—Textbooks. 4. Meteorology—Textbooks. I. Hobbs, Peter Victor, 1936–2005 II. Title. QC861.3.W35 2006 551.5—dc22 2005034642 British Library Cataloguing-in-Publication Data A catalogue record for this book is available from the British Library. ISBN 13: 978-0-12-732951-2 ISBN 10: 0-12-732951-X For information on all Academic Press Publications visit our Web site at www.books.elsevier.com Printed in Canada 06 07 08 09 10 8 7 6 5 4 3 2 1 Working together to grow libraries in developing countries www.elsevier.com | www.bookaid.org | www.sabre.org P732951-Prelims.qxd 12/16/05 10:54 AM Page v In Memory of Peter V. Hobbs (1936–2005) I am the daughter of Earth and Water, And the nursling of the Sky; I pass through the pores of the ocean and shores; I change, but I cannot die. For after the rain when with never a stain The pavilion of Heaven is bare, And the winds and sunbeams with their convex gleams Build up the blue dome of air, I silently laugh at my own cenotaph, And out of the caverns of rain, Like a child from the womb, like a ghost from the tomb, I arise and unbuild it again. PERCY BYSSHE SHELLEY The Cloud v P732951-Prelims.qxd 12/16/05 10:54 AM Page vi P732951-Prelims.qxd 12/16/05 10:54 AM Page vii Contents Preface to the Second Edition xi 2.5.3 The Past Million Years 52 Preface to the First Edition xv 2.5.4 The Past 20,000 Years 54 2.6 Earth: The Habitable Planet 56 1 Introduction and Overview 1 Exercises 58 1.1 Scope of the Subject and Recent Highlights 1 3 Atmospheric Thermodynamics 63 1.2 Some Definitions and Terms of 3.1 Gas Laws 63 Reference 3 3.1.1 Virtual Temperature 66 1.3 A Brief Survey of the Atmosphere 6 3.2 The Hydrostatic Equation 67 1.3.1 Optical Properties 6 3.2.1 Geopotential 68 1.3.2 Mass 7 3.2.2 Scale Height and the Hypsometric 1.3.3 Chemical Composition 8 Equation 69 1.3.4 Vertical structure 9 3.2.3 Thickness and Heights of Constant 1.3.5 Winds 12 Pressure Surfaces 71 1.3.6 Precipitation 19 3.2.4 Reduction of Pressure to Sea Level 72 1.4 What’s Next? 21 3.3 The First Law of Thermodynamics 72 Exercises 22 3.3.1 Joule’s Law 74 3.3.2 Specific Heats 75 2 The Earth System 25 3.3.3 Enthalpy 75 2.1 Components of the Earth System 25 3.4 Adiabatic Processes 76 2.1.1 The Oceans 25 3.4.1 Concept of an Air Parcel 77 2.1.2 The Cryosphere 32 3.4.2 The Dry Adiabatic Lapse Rate 77 2.1.3 The Terrestrial Biosphere 35 3.4.3 Potential Temperature 77 2.1.4 The Earth’s Crust and Mantle 37 3.4.4 Thermodynamic Diagrams 78 2.1.5 Roles of Various Components 3.5 Water Vapor in Air 79 of the Earth System in Climate 38 3.5.1 Moisture Parameters 80 2.2 The Hydrologic Cycle 38 3.5.2 Latent Heats 84 2.3 The Carbon Cycle 41 3.5.3 Saturated Adiabatic and 2.3.1 Carbon in the Atmosphere 42 Pseudoadiabatic Processes 84 2.3.2 Carbon in the Biosphere 42 3.5.4 The Saturated Adiabatic Lapse Rate 84 2.3.3 Carbon in the Oceans 44 3.5.5 Equivalent Potential Temperature 2.3.4 Carbon in the Earth’s Crust 44 and Wet-Bulb Potential Temperature 85 2.4 Oxygen in the Earth System 45 3.5.6 Normand’s Rule 86 2.4.1 Sources of Free Oxygen 46 3.5.7 Net Effects of Ascent Followed 2.5 A Brief History of Climate and the by Descent 86 Earth System 48 3.6 Static Stability 88 2.5.1 Formation and Evolution of the 3.6.1 Unsaturated Air 88 Earth System 48 3.6.2 Saturated Air 91 2.5.2 The Past 100 Million Years 51 3.6.3 Conditional and Convective Instability 91 vii P732951-Prelims.qxd 12/16/05 10:54 AM Page viii viii Contents 3.7 The Second Law of Thermodynamics 5.4 Tropospheric Aerosols 169 and Entropy 93 5.4.1 Sources 170 3.7.1 The Carnot Cycle 93 5.4.2 Chemical Composition 172 3.7.2 Entropy 95 5.4.3 Transport 173 3.7.3 The Clausius–Clapeyron Equation 97 5.4.4 Sinks 173 3.7.4 Generalized Statement of the Second 5.4.5 Concentrations and Size Distributions 174 Law of Thermodynamics 100 5.4.6 Residence Times 176 Exercises 102 5.5 Air Pollution 176 5.5.1 Some Sources of Pollutants 177 4 Radiative Transfer 113 5.5.2 Smogs 179 4.1 The Spectrum of Radiation 113 5.5.3 Regional and Global Pollution 181 4.2 Quantitative Description of Radiation 114 5.6 Tropospheric Chemical Cycles 182 4.3 Blackbody Radiation 117 5.6.1 The Nitrogen Cycle 182 4.3.1 The Planck Function 117 5.6.2 The Sulfur Cycle 183 4.3.2 Wien’s Displacement Law 118 5.7 Stratospheric Chemistry 184 4.3.3 The Stefan–Boltzmann Law 119 5.7.1 Unperturbed Stratospheric Ozone 185 4.3.4 Radiative Properties of 5.7.2 Anthropogenic Perturbations to Nonblack Materials 120 Stratospheric Ozone: The Ozone Hole 190 4.3.5 Kirchhoff’s Law 121 5.7.3 Stratospheric Aerosols; Sulfur in the 4.3.6 The Greenhouse Effect 121 Stratosphere 196 4.4 Physics of Scattering and Absorption Exercises 198 and Emission 122 4.4.1 Scattering by Air Molecules and 6 Cloud Microphysics 209 Particles 123 6.1 Nucleation of Water Vapor Condensation 209 4.4.2 Absorption by Particles 126 6.1.1 Theory 210 4.4.3 Absorption and Emission by 6.1.2 Cloud Condensation Nuclei 214 Gas Molecules 127 6.2 Microstructures of Warm Clouds 215 4.5 Radiative Transfer in Planetary 6.3 Cloud Liquid Water Content and Atmospheres 130 Entrainment 218 4.5.1 Beer’s Law 130 6.4 Growth of Cloud Droplets in Warm Clouds 221 4.5.2 Reflection and Absorption by a Layer 6.4.1 Growth by Condensation 221 of the Atmosphere 133 6.4.2 Growth by Collection 224 4.5.3 Absorption and Emission of Infrared 6.4.3 Bridging the Gap between Droplet Radiation in Cloud-Free Air 134 Growth by Condensation and 4.5.4 Vertical Profiles of Radiative Collision–Coalescence 228 Heating Rate 138 6.5 Microphysics of Cold Clouds 232 4.5.5 Passive Remote Sensing by Satellites 139 6.5.1 Nucleation of Ice Particles; Ice Nuclei 232 4.6 Radiation Balance at the Top of the 6.5.2 Concentrations of Ice Particles in Atmosphere 144 Clouds; Ice Multiplication 236 Exercises 145 6.5.3 Growth of Ice Particles in Clouds 238 6.5.4 Formation of Precipitation in Cold 5 Atmospheric Chemistry 153 Clouds 243 5.1 Composition of Tropospheric Air 153 6.5.5 Classification of Solid Precipitation 245 5.2 Sources, Transport, and Sinks of Trace Gases 157 6.6 Artificial Modification of Clouds and 5.2.1 Sources 157 Precipitation 245 5.2.2 Transport 160 6.6.1 Modification of Warm Clouds 245 5.2.3 Sinks 162 6.6.2 Modification of Cold Clouds 247 5.3 Some Important Tropospheric Trace Gases 162 6.6.3 Inadvertent Modification 250 5.3.1 The Hydroxyl Radical 162 6.7 Thunderstorm Electrification 252 5.3.2 Some Reactive Nitrogen Compounds 163 6.7.1 Charge Generation 252 5.3.3 Organic Compounds 164 6.7.2 Lightning and Thunder 254 5.3.4 Carbon Monoxide 165 6.7.3 The Global Electrical Circuit 256 5.3.5 Ozone 165 6.8 Cloud and Precipitation Chemistry 259 5.3.6 Hydrogen Compounds 168 6.8.1 Overview 259 5.3.7 Sulfur Gases 168 6.8.2 Transport of Particles and Gases 259 P732951-Prelims.qxd 12/16/05 10:54 AM Page ix Contents ix 6.8.3 Nucleation Scavenging 260 8.2.3 Cold Air Damming 341 6.8.4 Dissolution of Gases in Cloud Droplets 260 8.2.4 Terrain-Induced Windstorms 342 6.8.5 Aqueous-Phase Chemical Reactions 261 8.2.5 Orographic Influences on 6.8.6 Precipitation Scavenging 261 Precipitation 343 6.8.7 Sources of Sulfate in Precipitation 262 8.3 Deep Convection 344 6.8.8 Chemical Composition of Rain 262 8.3.1 Environmental Controls 345 6.8.9 Production of Aerosol by Clouds 262 8.3.2 Structure and Evolution of Exercises 263 Convective Storms 349 8.3.3 Damaging Winds Associated with 7 Atmospheric Dynamics 271 Convective Storms 356 7.1 Kinematics of the Large-Scale Horizontal 8.3.4 Mesoscale Convective Systems 363 Flow 271 8.4 Tropical Cyclones 366 7.1.1 Elementary Kinematic Properties 8.4.1 Structure, Thermodynamics, of the Flow 271 and Dynamics 366 7.1.2 Vorticity and Divergence 272 8.4.2 Genesis and Life Cycle 369 7.1.3 Deformation 274 8.4.3 Storm Surges 370 7.1.4 Streamlines versus Trajectories 275 Exercises 371 7.2 Dynamics of Horizontal Flow 276 7.2.1 Apparent Forces 276 9 The Atmospheric Boundary Layer 375 7.2.2 Real Forces 279 9.1 Turbulence 376 7.2.3 The Horizontal Equation of Motion 280 9.1.1 Eddies and Thermals 376 7.2.4 The Geostrophic Wind 281 9.1.2 Statistical Description of Turbulence 378 7.2.5 The Effect of Friction 281 9.1.3 Turbulence Kinetic Energy and 7.2.6 The Gradient Wind 282 Turbulence Intensity 379 7.2.7 The Thermal Wind 283 9.1.4 Turbulent Transport and Fluxes 381 7.2.8 Suppression of Vertical Motions 9.1.5 Turbulence Closure 382 by Planetary Rotation 286 9.1.6 Turbulence Scales and Similarity 7.2.9 A Conservation Law for Vorticity 286 Theory 383 7.2.10 Potential Vorticity 289 9.2 The Surface Energy Balance 385 7.3 Primitive Equations 290 9.2.1 Radiative Fluxes 385 7.3.1 Pressure as a Vertical Coordinate 291 9.2.2 Surface Energy Balance over Land 386 7.3.2 Hydrostatic Balance 291 9.2.3 The Bulk Aerodynamic Formulae 387 7.3.3 The Thermodynamic Energy Equation 291 9.2.4 The Global Surface Energy Balance 390 7.3.4 Inference of the Vertical Motion Field 293 9.3 Vertical Structure 391 7.3.5 Solution of the Primitive Equations 295 9.3.1 Temperature 391 7.3.6 An Application of Primitive Equations 296 9.3.2 Humidity 392 7.4 The Atmospheric General Circulation 297 9.3.3 Winds 393 7.4.1 The Kinetic Energy Cycle 298 9.3.4 Day-to-Day and Regional Variations 7.4.2 The Atmosphere as a Heat Engine 300 in Boundary-Layer Structure 395 7.5 Numerical Weather Prediction 300 9.3.5 Nonlocal Influence of Stratification Exercises 304 on Turbulence and Stability 396 9.4 Evolution 398 8 Weather Systems 313 9.4.1 Entrainment 398 8.1 Extratropical Cyclones 313 9.4.2 Boundary-Layer Growth 399 8.1.1 An Overview 313 9.4.3 Cloud-Topped Boundary Layer 8.1.2 Fronts and Surface Weather 318 over Land 401 8.1.3 Vertical Structure 328 9.4.4 The Marine Boundary Layer 401 8.1.4 Air Trajectories 334 9.4.5 Stormy Weather 404 8.1.5 In Search of the Perfect Storm 336 9.5 Special Effects 404 8.1.6 Top–Down Influences 337 9.5.1 Terrain Effects 404 8.1.7 Influence of Latent Heat Release 338 9.5.2 Sea Breezes 408 8.2 Orographic Effects 340 9.5.3 Forest Canopy Effects 410 8.2.1 Lee Cyclogenesis and Lee Troughing 340 9.5.4 Urban Effects 411 8.2.2 Rossby Wave Propagation along 9.6 The Boundary Layer in Context 411 Sloping Terrain 340 Exercises 413 P732951-Prelims.qxd 12/16/05 10:54 AM Page x x Contents 10 Climate Dynamics 419 10.3.2 Climate Feedbacks 447 10.1 The Present-Day Climate 419 10.4 Greenhouse Warming 451 10.1.1 Annual Mean Conditions 419 10.4.1 The Buildup of Greenhouse Gases 451 10.1.2 Dependence on Time of Day 422 10.4.2 Is Human-Induced Greenhouse 10.1.3 Seasonal Dependence 423 Warming Already Evident? 455 10.2 Climate Variability 425 10.4.3 Projections of Future 10.2.1 Internally Generated Climate Human-Induced Greenhouse Variability 429 Warming 457 10.2.2 Coupled Climate Variability 431 10.5 Climate Monitoring and Prediction 458 10.2.3 Externally Forced Climate Variability 439 Exercises 460 10.3 Climate Equilibria, Sensitivity, and Constants and Conversions for Feedbacks 443 Atmospheric Science 467 10.3.1 Transient versus Equilibrium Abbreviations Used in Captions 469 Response 446 Index 471 P732951-Prelims.qxd 12/16/05 10:54 AM Page xi Preface to the Second 2 Edition In the 30 years that have passed since we embarked Storms, the Global Energy Balance, and the General on the first edition of this book, atmospheric science Circulation have been dropped, but much of the has developed into a major field of study with far- material that was contained in them has been moved reaching scientific and societal implications. Topics to other chapters. The coverage of atmospheric such as climate and atmospheric chemistry, which dynamics, radiative transfer, atmospheric electricity, were not deemed sufficiently important to warrant convective storms, and tropical cyclones has been chapters of their own 30 years ago, are now major expanded. The treatment of atmospheric thermody- branches of the discipline. More traditional topics namics has been modernized by using the skew such as weather forecasting, understanding the T ln p chart as the primary format for plotting processes that lead to severe storms, and the radia- soundings. The second edition contains many more tion balance of the Earth have been placed on firmer illustrations, most of which are in color. foundations. Satellite-borne sensors that were in the A popular feature of the first edition that is early stages of development 30 years ago are now retained in the second edition is the inclusion of providing comprehensive observations of Earth’s quantitative exercises with complete solutions atmosphere. Those who have witnessed these accom- embedded in the text of each chapter, as well as plishments and contributed to them, if only in minor additional exercises for the student at the end of ways, have been fortunate indeed. each chapter. The second edition retains these As we drafted new section after new section features. In addition, we have included many new describing these exciting new developments, we began exercises at the end of the chapters, and (available to wonder whether we would still be capable of cram- to instructors only) a nearly complete set of solu- ming a summary of the entire field of atmospheric sci- tions for the exercises. Boxes are used in the second ence into a book light enough to be carried in a edition as a vehicle for presenting topics or lines of student’s backpack. This second edition does, in fact, reasoning that are outside the mainstream of the contain much more material than its predecessor, but text. For example, in Chapter 3 a qualitative statisti- thanks to the double column formatting and the sup- cal mechanics interpretation of the gas laws and the plementary Web site, it is not correspondingly heavier. first law of thermodynamics is presented in a series In deciding which of the recent developments to of boxes. include and which ones to leave out, we have elected Academic Press is providing two web sites in sup- to emphasize fundamental principles that will stand port of the book. The first web site includes informa- students in good stead throughout their careers, tion and resources for all readers, including a eschewing unnecessary details, interesting though they printable, blank skew T-ln p chart, answers to most of may be, and important to the specialist. the exercises, additional solved exercises that we did The second edition contains new chapters on not have space to include in the printed text, errata, an atmospheric chemistry, the atmospheric boundary appendix on global weather observations and data layer, the Earth system, and climate dynamics. The assimilation, and climate data for use in the exercises. chapters in the first edition entitled Clouds and The second web site, which will be accessible only to xi P732951-Prelims.qxd 12/16/05 10:54 AM Page xii xii Preface to the Second Edition instructors, contains a short instructor’s guide, solu- Despite his illness, Peter continued to revise his tions to most of the exercises, electronic versions of chapters and offer helpful feedback on mine. Even most of the figures that appear in the book, and elec- after he was no longer able to engage in spirited tronic versions of a set of supplementary figures that debates about the content of the book, he continued may be useful in customizing classroom presentations. to wield his infamous red pen, pointing out grammat- To use the book as a text for a broad survey ical mistakes and editorial inconsistencies in my course, the instructor would need to be selective, chapters. A few months before his death, July 25, omitting much of the more advanced material from 2005, we enjoyed a party celebrating (albeit a bit pre- the quantitative Chapters 3, 4, 5, and 7, as well as sec- maturely) the completion of the project that he had tions of other, more descriptive chapters. Selected initiated. chapters of the book can be used as a text for several For the dedication of the first edition, it was different kinds of courses. For example, Chapters 3–6 Peter’s choice to use Shelley’s poem, “Clouds,” a could be used in support of an atmospheric physics visual metaphor for life, death, and renewal. For the and chemistry course; Chapters 1, 3, 7, and 8 for a second edition, I have chosen the same poem, this course emphasizing weather; and Chapters 1 and 2 time in memory of Peter. and parts of 3, 4, and 9 and Chapter 10 in support of Several members of Peter’s “Cloud and Aerosol a course on climate in a geosciences curriculum. Research Group” were instrumental in preparing the Corrigenda and suggestions for the instructor’s book for publication. Debra Wolf managed the man- guide will be gratefully received. uscript and produced many of the illustrations, Judith Opacki obtained most of the permissions, Arthur Rangno provided several cloud photos, and he and Mark Stoelinga provided valuable scientific advice. John M. Wallace Peter and I are indebted to numerous individuals Peter V. Hobbs who have generously contributed to the design, con- Seattle, January 2005 tent, and production of this edition. Roland Stull at the University of British Columbia is the primary author of Chapter 9 (the Atmospheric Boundary Layer). Three of our colleagues in the Department Acknowledgments of Atmospheric Sciences at the University of In 1972, I accepted Peter Hobbs’ invitation to collab- Washington served as advisors for portions of other orate with him in writing an introductory atmospheric chapters. Qiang Fu advised us on the design of science textbook. We agreed that he would take the Chapter 4 (Radiative Transfer) and provided some of lead in drafting the thermodynamics and cloud the material for it. Lynn McMurdie selected the case physics chapters and I would be primarily responsible study presented in Section 8.1 (Extratropical for the chapters dealing with radiative transfer, syn- Cyclones) and advised us on the content for that sec- optic meteorology, and dynamic meteorology. Over tion. Robert A. Houze advised us on the design and the course of the following few years we struggled content of Section 8.3 (Convective Storms) and to reconcile his penchant for rigor and logic with Section 8.4 (Tropical Cyclones). Other colleagues, my more intuitive, visually based writing style. These Stephen Warren, Clifford Mass, Lyatt Jaegle, Andrew spirited negotiations tested and ultimately cemented Rice, Marcia Baker, David Catling, Joel Thornton, and our friendship and led to a text that was better than Greg Hakim, read and provided valuable feedback on either of us could have produced working in isolation. early drafts of chapters. Others who provided valuable Three years ago, on a walk together in the rain, feedback and technical advice on specific parts of Peter warned me that if I wanted to produce that long the manuscript include Edward Sarachik, Igor overdue second edition, we needed to get started Kamenkovich, Richard Gammon, Joellen Russell, soon because he was contemplating retirement in a Conway Leovy, Norbert Untersteiner, Kenneth Beard, few years. When I agreed, he immediately set to work William Cotton, Hermann Gerber, Shuyi Chen, on his chapters, including an entirely new chapter on Howard Bluestein, Robert Wood, Adrian Simmons, atmospheric chemistry, and completed drafts of them Michael King, David Thompson, Judith Lean, Alan by the end of 2003. Soon afterward, he was diagnosed Robock, Peter Lynch, Paquita Zuidema, Cody with pancreatic cancer. Kirkpatrick, and J. R. Bates. I also thank the graduate P732951-Prelims.qxd 12/16/05 10:54 AM Page xiii Preface to the Second Edition xiii students who volunteered their help in identifying photographs of clouds and other atmospheric phe- errors, inconsistencies, and confusing passages in the nomena that appear in the book were generously numerous drafts of the manuscript. provided free of charge. Jennifer Adams, a research scientist at the Center I am deeply indebted to Qiang Fu and Peter Lynch for Ocean–Land–Atmosphere Studies (COLA), pro- who generously volunteered their time to correct duced most of the illustrations that appear in Section errors in the equations, as well as James Booth, Joe 8.1, under funding provided by COLA and using Casola, Ioana Dima, Chaim Garfinkel, David graphics software (GrADS) developed at COLA. Reidmiller Kevin Rennert, Rei Ueyama, Justin Some of the design elements in the illustrations were Wettstein and Reddy Yatavelli who identified many provided by David W. Ehlert. Debra Wolf, Candace errors in the cross-referencing. Gudmundson, Kay Dewar, and Michael Macaulay Finally, I thank Peter’s wife, Sylvia, and my wife, and Beth Tully prepared many of the illustrations. Susan, for their forbearance during the many Steven Cavallo and Robert Nicholas provided the evenings and weekends in which we were preoccu- table of units and numerical values. Most of the pied with this project. P732951-Prelims.qxd 12/16/05 10:54 AM Page xiv P732951-Prelims.qxd 12/16/05 10:54 AM Page xv Preface to the First Edition 2 This book has been written in response to a need for discussions, which are an integral part of many intro- a text to support several of the introductory courses ductory survey courses. in atmospheric sciences commonly taught in univer- The book is divided into nine chapters. Most of the sities, namely introductory survey courses at the jun- basic theoretical material is covered in the even- ior or senior undergraduate level and beginning numbered chapters (2, 4, 6, and 8). Chapters 1 and 3 graduate level, the undergraduate physical meteorol- are almost entirely descriptive, while Chapters 5, 7, ogy course, and the undergraduate synoptic labora- and 9 are mainly interpretive in character. Much of tory. These courses serve to introduce the student to the material in the odd-numbered chapters is the fundamental physical principles upon which the straightforward enough to be covered by means of atmospheric sciences are based and to provide an reading assignments, especially in graduate courses. elementary description and interpretation of the However, even with extensive use of reading assign- wide range of atmospheric phenomena dealt with in ments we recognize that it may not be possible to detail in more advanced courses. In planning the completely cover a book of this length in a one- book we have assumed that students enrolled in such semester undergraduate course. In order to facilitate courses have already had some exposure to calculus the use of the book for such courses, we have pur- and physics at the first-year college level and to posely arranged the theoretical chapters in such a chemistry at the high school level. way that certain of the more difficult sections can be The subject material is almost evenly divided omitted without serious loss of continuity. These sec- between physical and dynamical meteorology. In the tions are indicated by means of footnotes. general area of physical meteorology we have intro- Descriptive and interpretive material in the other duced the basic principles of atmospheric hydrostat- chapters can be omitted at the option of the instruc- ics and thermodynamics, cloud physics, and radiative tor. transfer (Chapters 2, 4, and 6, respectively). In addi- The book contains 150 numerical problems and tion, we have covered selected topics in atmospheric 208 qualitative problems that illustrate the applica- chemistry, aerosol physics, atmospheric electricity, tion of basic physical principles to problems in the aeronomy, and physical climatology. Coverage of atmospheric sciences. In addition, the solutions of 48 dynamical meteorology consists of a description of of the numerical problems are incorporated into the large-scale atmospheric motions and an elementary text. We have purposely designed problems that interpretation of the general circulation (Chapters 3, require a minimum amount of mathematical manipu- 8, and 9, respectively). In the discussion of clouds and lation in order to place primary emphasis on the storms (Chapter 5) we have attempted to integrate proper application of physical principles. Universal material from physical and dynamical meteorology. constants and other data needed for the solution of In arranging the chapters we have purposely placed quantitative problems are given on pages xvi–xvii. the material on synoptic meteorology near the begin- It should be noted that many of the qualitative ning of the book (Chapter 3) in order to have it problems at the ends of the chapters require some available as an introduction to the daily weather map original thinking on the part of the student. We have xv P732951-Prelims.qxd 12/16/05 10:54 AM Page xvi xvi Preface to the First Edition found such questions useful as a means of stimulat- scholarly activities that contributed to this book. ing classroom discussion and helping the students to While working on the book, one of us (J.M.W.) was prepare for examinations. privileged to spend 6 months on an exchange visit to Throughout the book we have consistently used SI the Computer Center of the Siberian Branch of the units, which are rapidly gaining acceptance within the Soviet Academy of Sciences, Novosibirsk, USSR, atmospheric sciences community. and a year at the US National Center for A list of units and symbols is given on pages Atmospheric Sciences under the auspices of the xv–xvi. Advanced Study Program. The staff members and The book contains biographical footnotes that visitors at both of these institutions made many summarize the lives and work of scientists who have important contributions to the scientific content of made major contributions to the atmospheric sci- the book. Thanks go also to many other individuals ences. Brief as these are, it is hoped that they will in the scientific community who provided help and give the student a sense of the long history of mete- guidance. orology and its firm foundations in the physical sci- We wish especially to express our gratitude to col- ences. As a matter of policy we have included leagues in our own department who provided a con- footnotes only for individuals who are deceased or tinuous source of moral support, constructive retired. criticism, and stimulating ideas. Finally, we acknowl- We express our gratitude to the University of edge the help received from many individuals who Washington and the National Science Foundation aided in the preparation of the final manuscript, as for their support of our teaching, research, and other well as the many interim manuscripts that preceded it. P732951-Ch01 11/03/05 04:56 PM Page 1 Introduction and Overview 1 1.1 Scope of the Subject “nowcasting”), and departures of the climate (i.e., the statistics of weather) from seasonally adjusted and Recent Highlights normal values out to a year in advance. Atmospheric science is a relatively new, applied disci- Weather forecasting has provided not only the pline that is concerned with the structure and evolu- intellectual motivation for the development of tion of the planetary atmospheres and with the wide atmospheric science, but also much of the infra- range of phenomena that occur within them. To the structure. What began in the late 19th century as an extent that it focuses mainly on the Earth’s atmos- assemblage of regional collection centers for real phere, atmospheric science can be regarded as one of time teletype transmissions of observations of sur- the Earth or geosciences, each of which represents a face weather variables has evolved into a sophisti- particular fusion of elements of physics, chemistry, cated observing system in which satellite and in situ and fluid dynamics. measurements of many surface and upper air vari- The historical development of atmospheric sci- ables are merged (or assimilated) in a dynamically ences, particularly during the 20th century, has consistent way to produce optimal estimates of been driven by the need for more accurate weather their respective three-dimensional fields over the forecasts. In popular usage the term “meteorolo- entire globe. This global, real time atmospheric gist,” a synonym for atmospheric scientist, means dataset is the envy of oceanographers and other “weather forecaster.” During the past century, geo- and planetary scientists: it represents both an weather forecasting has evolved from an art that extraordinary technological achievement and an relied solely on experience and intuition into a sci- exemplar of the benefits that can derive from inter- ence that relies on numerical models based on the national cooperation. Today’s global weather conservation of mass, momentum, and energy. The observing system is a vital component of a broader increasing sophistication of the models has led to Earth observing system, which supports a wide dramatic improvements in forecast skill, as docu- variety of scientific endeavors, including climate mented in Fig. 1.1. Today’s weather forecasts monitoring and studies of ecosystems on a global address not only the deterministic, day-to-day evo- scale. lution of weather patterns over the course of the A newer, but increasingly important organizing next week or two, but also the likelihood of haz- theme in atmospheric science is atmospheric ardous weather events (e.g., severe thunderstorms, chemistry. A generation ago, the principal focus of freezing rain) on an hour-by-hour basis (so called this field was urban air quality. The field experienced 1 P732951-Ch01 11/03/05 04:56 PM Page 2 2 Introduction and Overview 100 Day 3 90 Day 5 Level of skill (%) 80 70 Day 7 60 50 40 Northern hemisphere Southern hemisphere 30 85 90 95 00 05 Year Fig. 1.1 Improvement of forecast skill with time from 1981 to 2003. The ordinate is a measure of forecast skill, where 100% represents a perfect forecast of the hemispheric flow pattern at the 5-km level. The upper pair of curves is for 3-day forecasts, the middle pair for 5-day forecasts, and the lower pair for 7-day forecasts. In each pair, the upper curve that marks the top of the band of shading represents the skill averaged over the north- ern hemisphere and the lower curve represents the skill averaged over the southern hemisphere. Note the continually improving Fig. 1.2 The Antarctic ozone hole induced by the buildup of skill levels (e.g., today’s 5-day forecasts of the northern hemi- synthetic chlorofluorocarbons, as reflected in the distribution sphere flow pattern are nearly as skillful as the 3-day forecasts of of vertically integrated ozone over high latitudes of the south- 20 years ago). The more rapid increase in skill in the southern ern hemisphere in September, 2000. Blue shading represents hemisphere reflects the progress that has been made in assimi- substantially reduced values of total ozone relative to the sur- lating satellite data into the forecast models. [Updated from rounding region rendered in green and yellow. [Based on data Quart. J. Royal Met. Soc., 128, p. 652 (2002). Courtesy of the from NASA TOMS Science Team; figure produced by NASA’s European Centre for Medium-Range Weather Forecasting.] Scientific Visualization Studio.] a renaissance during the 1970s when it was discov- widely used for refrigeration and various industrial ered that forests and organisms living in lakes over purposes. As in the acid rain problem, heteroge- parts of northern Europe, the northeastern United neous chemical reactions involving cloud droplets States, and eastern Canada were being harmed by were implicated, but in the case of the “ozone hole” acid rain caused by sulfur dioxide emissions from they were taking place in wispy polar stratospheric coal-fired electric power plants located hundreds clouds. Knowledge gained from atmospheric chem- and, in some cases, thousands of kilometers upwind. istry research has been instrumental in the design The sources of the acidity are gaseous oxides of sul- of policies to control and ultimately reverse the fur and nitrogen (SO2, NO, NO2, and N2O5) that dis- spread of acid rain and the ozone hole. The unre- solve in microscopic cloud droplets to form weak solved scientific issues surrounding greenhouse solutions of sulfuric and nitric acids that may reach warming caused by the buildup of carbon dioxide the ground as raindrops. (Fig. 1.3) and other trace gases released into the There is also mounting evidence of the influence atmosphere by human activities pose a new chal- of human activity on the composition of the global lenge for atmospheric chemistry and for the atmosphere. A major discovery of the 1980s was broader field of geochemistry. the Antarctic “ozone hole”: the disappearance of Atmospheric science also encompasses the much of the stratospheric ozone layer over the emerging field of climate dynamics. As recently as a southern polar cap each spring (Fig. 1.2). The ozone generation ago, climatic change was viewed by most destruction was found to be caused by the break- atmospheric scientists as occurring on such long down of chlorofluorocarbons (CFCs), a family of timescales that, for most purposes, today’s climate synthetic gases that was becoming increasingly could be described in terms of a standard set of P732951-Ch01 11/03/05 04:56 PM Page 3 1.2 Some Definitions and Terms of Reference 3 concentration(ppm by volume) 380 the nature and causes of climate variability, the atmos- 370 phere must be treated as a component of the Earth Atmospheric CO2 360 system. 350 Mauna Loa 340 South Pole 330 320 1.2 Some Definitions and Terms 310 58 60 65 70 75 80 85 90 95 00 02 of Reference Year Even though the Earth is not perfectly spherical, Fig. 1.3 Time series showing the upward trend in monthly atmospheric phenomena are adequately represented mean atmospheric CO2 concentrations (in parts per million in terms of a spherical coordinate system, rotating by volume) at Mauna Loa and the South Pole due to the with the Earth, as illustrated in Fig. 1.4. The coordi- burning of fossil fuels. A pronounced annual cycle is also evi- dent at Mauna Loa, with minimum values in the summer. nates are latitude , longitude , and height z above [Based on data of C. D. Keeling. Courtesy of Todd P. sea level, z.1 The angles are often replaced by the Mitchell.] distances dx r d cos (1.1) and statistics, such as January climatological-mean (or “normal”) temperature. In effect, climatology and dy r d climate change were considered to be separate subfields, the former a branch of atmospheric where x is distance east of the Greenwich meridian sciences and the latter largely the province of disci- along a latitude circle, y is distance north of the plines such as geology, paleobotany, and geochem- istry. Among the factors that have contributed to the emergence of a more holistic, dynamic view of climate are: North Pole r co documentation of a coherent pattern of year-to- sφ year climate variations over large areas of the P globe that occurs in association with El Niño r (Section 10.2). =0 φ proxy evidence, based on a variety of sources O (ocean sediment cores and ice cores, in particular), E q u a t or φ=0 indicating that large, spatially coherent climatic changes have occurred on time scales of a century or even less (Section 2.6.4). the rise of the global-mean surface air temperature during the 20th century and South Pole projections of a larger rise during the 21st century due to human activities (Section 10.4). Fig. 1.4 Coordinate system used in atmospheric science. Angle is latitude, defined as positive in the northern hemi- sphere and negative in the southern hemisphere, and is lon- Like some aspects of atmospheric chemistry, climate gitude relative to the Greenwich meridian, positive eastward. dynamics is inherently multidisciplinary: to understand The radial coordinate (not shown) is height above sea level. 1 Oceanographers and applied mathematicians often use the colatitude 2 instead of . P732951-Ch01 11/03/05 04:56 PM Page 4 4 Introduction and Overview dy d v RE (the meridional velocity component), dt dt and dz dr w (the vertical velocity component). d dt where z is height above mean sea level. The adjec- tives zonal and meridional are also commonly used in reference to averages, gradients, and cross sections. For example, a zonal average denotes an average around latitude circles; a meridional cross section denotes a north–south slice through the atmosphere. The horizontal velocity vector V is given by V ui vj, where i and j are the unit vectors in the zonal and meridional directions, respectively. Positive and negative zonal veloci- ties are referred to as westerly (from the west) Fig. 1.5 The limb of the Earth, as viewed from space in visi- and easterly (from the east) winds, respectively; ble satellite imagery. The white layer is mainly light scattered positive and negative meridional velocities are from atmospheric aerosols and the overlying blue layer is referred to as southerly and northerly winds (in mainly light scattered by air molecules. [NASA Gemini-4 both northern and southern hemispheres, respec- photo. Photograph courtesy of NASA.] tively.2 For scales of motion in the Earth’s atmos- phere in excess of 100 km, the length scale greatly exceeds the depth scale, and typical magnitudes of the horizontal velocity component V exceed equator, and r is the distance from the center of the those of the vertical velocity component w by sev- Earth. At the Earth’s surface a degree of latitude is eral orders of magnitude. For these scales the term equivalent to 111 km (or 60 nautical miles). wind is synonymous with horizontal velocity Because 99.9% of the mass of the atmosphere is component. The SI unit for velocity (or speed) is concentrated within the lowest 50 km, a layer m s1. One meter per second is equivalent to with a thickness less than 1% of the radius of the 1.95 knots (1 knot 1 nautical mile per hour). Earth, r, is nearly always replaced by the mean Vertical velocities in large-scale atmospheric radius of the Earth (6.37 106 m), which we motions are often expressed in units of cm s1: denote by the symbol RE. Images of the limb of the 1 cm s1 is roughly equivalent to a vertical dis- Earth (Fig. 1.5) emphasize how thin the atmos- placement of 1 kilometer per day. phere really is. Throughout this book, the local derivative t The three velocity components used in describing refers to the rate of change at a fixed point in rotat- atmospheric motions are defined as ing (x, y, z) space and the total time derivative ddt refers to the rate of change following an air parcel as dx d (the zonal velocity it moves along its three-dimensional trajectory u RE cos (1.2) dt dt component) through the atmosphere. These so-called Eulerian3 2 Dictionaries offer contradictory definitions of these terms, derived from different traditions. 3 Leonhard Euler (1707–1783) Swiss mathematician. Held appointments at the St. Petersburg Academy of Sciences and the Berlin Academy. Introduced the mathematical symbols e, i, and f(x). Made fundamental contributions in optics, mechanics, electricity, and mag- netism, differential equations, and number theory. First to describe motions in a rotating coordinate system. Continued to work produc- tively after losing his sight by virtue of his extraordinary memory. P732951-Ch01 11/03/05 04:56 PM Page 5 1.2 Some Definitions and Terms of Reference 5 and Lagrangian4 rates of change are related by the of pressure is 1 N m2 1 kg m1 s2 1 pascal chain rule (Pa). Prior to the adoption of SI units, atmospheric pressure was expressed in millibars (mb), where d 1 bar 106 g cm1 s2 106 dynes. In the interests u w dt t x y z of retaining the numerical values of pressure that atmospheric scientists and the public have become which can be rewritten in the form accustomed to, atmospheric pressure is usually expressed in units of hundreds of (i.e., hecto) pascals d (hPa).5 Density is expressed in units of kg m3 and u w (1.3) t dt x y z temperature in units of °C or K, depending on the context, with °C for temperature differences and K The terms involving velocities in Eq. (1.3), including for the values of temperature itself. Energy is the minus signs in front of them, are referred to as expressed in units of joules (J kg m2 s2). advection terms. At a fixed point in space the Atmospheric phenomena with timescales shorter Eulerian and Lagrangian rates of change of a vari- than a few weeks, which corresponds to the theoreti- able differ by virtue of the advection of air from cal limit of the range of deterministic (day-by-day) upstream, which carries with it higher or lower values weather forecasting, are usually regarded as relating of. For a hypothetical conservative tracer, the to weather, and phenomena on longer timescales Lagrangian rate of change is identically equal to as relating to climate. Hence, the adage (intended zero, and the Eulerian rate of change is to apply to events a month or more in the future): “Climate is what you expect; weather is what you get.” Atmospheric variability on timescales of u w t x y z months or longer is referred to as climate variability, and statistics relating to conditions in a typical (as The fundamental thermodynamic variables are opposed to a particular) season or year are referred pressure p, density , and temperature T. The SI unit to as climatological-mean statistics. 1.1 Atmospheric Predictability and Chaos Atmospheric motions are inherently unpredictable weather forecast model that provided one of the as an initial value problem (i.e., as a system of equa- most lucid early demonstrations of this type of tions integrated forward in time from specified ini- behavior. tial conditions) beyond a few weeks. Beyond that In 1960, Professor Edward N. Lorenz in the time frame, uncertainties in the forecasts, no matter Department of Meteorology at MIT decided to how small they might be in the initial conditions, rerun an experiment with a simplified atmospheric become as large as the observed variations in model in order to extend his “weather forecast” atmospheric flow patterns. Such exquisite sensitivity farther out into the future. To his surprise, he to initial conditions is characteristic of a broad class found that he was unable to duplicate his previous of mathematical models of real phenomena, forecast. Even though the code and the prescribed referred to as chaotic nonlinear systems. In fact, it initial conditions in the two experiments were was the growth of errors in a highly simplified identical, the states of the model in the two fore- Continued on next page 4 Joseph Lagrange (1736–1813) French mathematician and mathematical physicist. Served as director of the Berlin Academy, succeed- ing Euler in that role. Developed the calculus of variations and also made important contributions to differential equations and number theory. Reputed to have told his students “Read Euler, read Euler, he is our master in everything.” 5 Although the pressure will usually be expressed in hectopascals (hPa) in the text, it should be converted to pascals (Pa) when work- ing quantitative exercises that involve a mix of units. P732951-Ch01 11/03/05 04:56 PM Page 6 6 Introduction and Overview 1.1 Continued casts diverged, over the course of the first few hundred time steps, to the point that they were no more like one another than randomly chosen states in experiments started from entirely differ- ent initial conditions. Lorenz eventually discov- ered that the computer he was using was introducing round-off errors in the last significant digit that were different each time he ran the experiment. Differences between the “weather patterns” in the different runs were virtually indis- tinguishable at first, but they grew with each time step until they eventually became as large as the range of variations in the individual model runs. Lorenz’s model exhibited another distinctive and quite unexpected form of behavior. For long periods of (simulated) time it would oscillate around some “climatological-mean” state. Then, for no apparent reason, the state of the model would undergo an abrupt “regime shift” and begin to oscillate around another quite different state, as illustrated in Fig. 1.6. Lorenz’s model exhibited two such preferred “cli- mate regimes.” When the state of the model resided within one of these regimes, the “weather” exhibited quasi-periodic oscillations and consequently was predictable quite far into the future. However, the shifts between regimes were abrupt, irregular, and inherently unpredictable beyond a few simulated Fig. 1.6 The history of the state of the model used days. Lorenz referred to the two climates in the by Lorenz can be represented as a trajectory in a three- model as attractors. dimensional space defined by the amplitudes of the model’s The behavior of the real atmosphere is much three dependent variables. Regime-like behavior is clearly more complicated than that of the highly simplified apparent in this rendition. Oscillations around the two dif- model used by Lorenz in his experiments. Whether ferent “climate attractors” correspond to the two, distinctly the Earth’s climate exhibits such regime-like behav- different sets of spirals, which lie in two different planes in ior, with multiple “attractors,” or whether it should the three-dimensional phase space. Transitions between the be viewed as varying about a single state that varies two regimes occur relatively infrequently. [Permission to use in time in response to solar, orbital, volcanic, and figure from Nature, 406, p. 949 (2000). © Copyright 2000 anthropogenic forcing is a matter of ongoing debate. Nature Publishing Group. Courtesy of Paul Bourke.] 1.3 A Brief Survey of the the conventions that will be used in performing calculations involving amounts of mass and rates Atmosphere of movement. The remainder of this chapter provides an overview of the optical properties, composition, and vertical 1.3.1 Optical Properties structure of the Earth’s atmosphere, the major wind systems, and the climatological-mean distribution of The Earth’s atmosphere is relatively transparent precipitation. It introduces some of the terminology to incoming solar radiation and opaque to outgoing that will be used in subsequent chapters and some of radiation emitted by the Earth’s surface. The blocking P732951-Ch01 11/03/05 04:56 PM Page 7 1.3 A Brief Survey of the Atmosphere 7 of outgoing radiation by the atmosphere, popularly whiteness (or reflectivity) of that region as viewed referred to as the greenhouse effect, keeps the surface from space. Due to the presence of clouds and of the Earth warmer than it would be in the absence aerosols in the Earth’s atmosphere, 22% of the of an atmosphere. Much of the absorption and ree- incoming solar radiation is backscattered to space mission of outgoing radiation are due to air mole- without being absorbed. The backscattering of radia- cules, but cloud droplets also play a significant role. tion by clouds and aerosols has a cooling effect on The radiation emitted to space by air molecules and climate at the Earth’s surface, which opposes the cloud droplets provides a basis for remote sensing of greenhouse effect. the three-dimensional distribution of temperature and various atmospheric constituents using satellite- borne sensors. 1.3.2 Mass The atmosphere also scatters the radiation that At any point on the Earth’s surface, the atmosphere passes through it, giving rise to a wide range of exerts a downward force on the underlying surface optical effects. The blueness of the outer atmosphere due to the Earth’s gravitational attraction. The down- in Fig. 1.5 is due to the preferential scattering of ward force, (i.e., the weight) of a unit volume of air incoming short wavelength (solar) radiation by air with density is given by molecules, and the whiteness of lower layers is due to scattering from cloud droplets and atmospheric F (1.4) aerosols (i.e., particles). The backscattering of solar radiation off the top of the deck of low clouds off the where is the acceleration due to gravity. California coast in Fig. 1.7 greatly enhances the Integrating Eq. (1.4) from the Earth’s surface to the “top” of the atmosphere, we obtain the atmos- pheric pressure on the Earth’s surface ps due to the weight (per unit area) of the air in the overlying column ps 0 dz (1.5) Neglecting the small variation of g with latitude, longitude and height, setting it equal to its mean value of 0 9.807 m s2, we can take it outside the integral, in which case, Eq. (1.5) can be writ- ten as ps m0 (1.6) where m dz is the vertically integrated mass 0 per unit area of the overlying air. Exercise 1.1 The globally averaged surface pressure is 985 hPa. Estimate the mass of the atmosphere. Solution: From Eq. (1.6), it follows that ps m 0 Fig. 1.7 A deck of low clouds off the coast of California, as viewed in reflected visible radiation. [NASA MODIS imagery. where the overbars denote averages over the surface Photograph courtesy of NASA.] of the Earth. In applying this relationship the pressure P732951-Ch01 11/03/05 04:56 PM Page 8 8 Introduction and Overview must be expressed in pascals (Pa). Substituting numer- mass of a constituent is computed by weighting its ical values we obtain fractional concentration by volume by its molecular weight, i.e., 985 102 PahPa m 1.004 104 kg m2 9.807 mi nM i i (1.7) mi niMi The mass of the atmosphere is where mi is the mass, ni the number of molecules, and Matm 4RE2 m Mi the molecular weight of the ith constituent, and 4 (6.37 106)2 m2 1.004 104 kg m2 the summations are over all constituents. 5.10 1014 m2 1.004 104 kg m2 Diatomic nitrogen (N2) and oxygen (O2) are the 5.10 1018 kg6 dominant constituents of the Earth’s atmosphere, and argon (Ar) is present in much higher concentra- tions than the other noble gases (neon, helium, kryp- ton, and xenon). Water vapor, which accounts for 1.3.3 Chemical Composition roughly 0.25% of the mass of the atmosphere, is a The atmosphere is composed of a mixture of gases highly variable constituent, with concentrations rang- in the proportions shown in Table 1.1, where frac- ing from around 10 parts per million by volume tional concentration by volume is the same as that (ppmv) in the coldest regions of the Earth’s atmos- based on numbers of molecules, or partial pressures phere up to as much as 5% by volume in hot, humid exerted by the gases, as will be explained more air masses; a range of more than three orders of mag- fully in Section 3.1. The fractional concentration by nitude. Because of the large variability of water vapor concentrations in air, it is customary to list the percentages of the various constituents in relation to dry air. Ozone concentrations are also highly vari- Table 1.1 Fractional concentrations by volume of the major able. Exposure to ozone concentrations >0.1 ppmv is gaseous constituents of the Earth’s atmosphere up to an considered hazardous to human health. altitude of 105 km, with respect to dry air For reasons that will be explained in §4.4, gas mol- ecules with certain structures are highly effective at Fractional Molecular concentration trapping outgoing radiation. The most important of Constituenta weight by volume these so-called greenhouse gases are water vapor, car- bon dioxide, and ozone. Trace constituents CH4, N2O, Nitrogen (N2) 28.013 78.08% CO, and chlorofluorocarbons (CFCs) are also signifi- Oxygen (O2) 32.000 20.95% cant contributors to the greenhouse effect. Argon (Ar) 39.95 0.93% Among the atmosphere’s trace gaseous con- Water vapor (H2O) 18.02 0–5% stituents are molecules containing carbon, nitrogen, Carbon dioxide (CO2) 44.01 380 ppm and sulfur atoms that were formerly incorporated Neon (Ne) 20.18 18 ppm into the cells of living organisms. These gases enter Helium (He) 4.00 5 ppm the atmosphere through the burning of plant matter and fossil fuels, emissions from plants, and the decay Methane (CH4) 16.04 1.75 ppm of plants and animals. The chemical transformations Krypton (Kr) 83.80 1 ppm that remove these chemicals from the atmosphere Hydrogen (H2) 2.02 0.5 ppm involve oxidation, with the hydroxyl (OH) radical Nitrous oxide (N2O) 56.03 0.3 ppm playing an important role. Some of the nitrogen and Ozone (O3) 48.00 0–0.1 ppm sulfur compounds are converted into particles that a So called greenhouse gases are indicated by bold-faced type. For more detailed are eventually “scavenged” by raindrops, which con- information on minor constituents, see Table 5.1. tribute to acid deposition at the Earth’s surface. 6 When the vertical and meridional variations in and the meridional variations in the radius of the earth are accounted for, the mass per unit area and the total mass of the atmosphere are 0.4% larger than the estimates derived here. P732951-Ch01 11/03/05 04:56 PM Page 9 1.3 A Brief Survey of the Atmosphere 9 Although aerosols and cloud droplets account for Because the pressure at a given height in the only a minute fraction of the mass of the atmos- atmosphere is a measure of the mass that lies above phere, they mediate the condensation of water vapor that level, it is sometimes used as a vertical coordi- in the atmospheric branch of the hydrologic cycle, nate in lieu of height. In terms of mass, the 500-hPa they participate in and serve as sites for important level, situated at a height of around 5.5 km above chemical reactions, and they give rise to electrical sea level, is roughly halfway up to the top the charge separation and a variety of atmospheric opti- atmosphere. cal effects. Density decreases with height in the same manner as pressure. These vertical variations in pressure and 1.3.4 Vertical structure density are much larger than the corresponding hori- To within a few percent, the density of air at sea level zontal and time variations. Hence it is useful to is 1.25 kg m3. Pressure p and density decrease define a standard atmosphere, which represents the nearly exponentially with height, i.e., horizontally and temporally averaged structure of the atmosphere as a function of height only, as shown p p0ezH (1.8) in Fig. 1.8. The nearly exponential height dependence of pressure and density can be inferred from the fact where H, the e-folding depth, is referred to as the that the observed vertical profiles of pressure and scale height and p0 is the pressure at some reference density on these semilog plots closely resemble level, which is usually taken as sea level (z 0). In straight lines. The reader is invited to verify in the lowest 100 km of the atmosphere, the scale height Exercise 1.14 at the end of this chapter that the cor- ranges roughly from 7 to 8 km. Dividing Eq. (1.8) by responding 10-folding depth for pressure and density p0 and taking the natural logarithms yields is 17 km. p z Exercise 1.3 Assuming an exponential pressure ln (1.9) p0 H and density dependence with H 7.5 km, estimate the heights in the atmosphere at which (a) the air This relationship is useful for estimating the height of density is equal to 1 kg m3 and (b) the height at various pressure levels in the Earth’s atmosphere. which the pressure is equal to 1 hPa. Exercise 1.2 At approximately what height above sea level zm does half the mass of the atmosphere lie 160 above and the other half lie below? [Hint: Assume an exponential pressure dependence with H 8 km 140 Height above sea level (km) and neglect the small vertical variation of g with 120 height.] 100 Solution: Let pm be the pressure level that half 80 the mass of the atmosphere lies above and half lies below. The pressure at the Earth’s surface is equal