Sex Determination in Plants PDF
Document Details
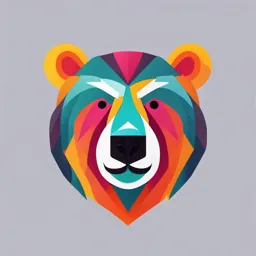
Uploaded by UndamagedSteelDrums1532
Tags
Summary
This document explores the diverse mechanisms of sex determination in plants. It examines the evolutionary perspectives and developmental genetic aspects of plant sexuality, ultimately highlighting the diversity of mechanisms observed across different plant lineages.
Full Transcript
**SEX DETERMINATION IN PLANTS** **INTRODUCTION:** **Land plants are remarkably diverse in their sexual systems, but the rich variety of ways in which they express their sexuality has largely been built on a conserved foundation that determines whether reproductive tissues produce egg cells or sper...
**SEX DETERMINATION IN PLANTS** **INTRODUCTION:** **Land plants are remarkably diverse in their sexual systems, but the rich variety of ways in which they express their sexuality has largely been built on a conserved foundation that determines whether reproductive tissues produce egg cells or sperm. In this sense, plants are similar to most other eukaryotes. But when should the cell cycle end in the ultimate production of eggs versus sperm, which individuals or flowers should make this decision, and how should the decision be acted upon in developmental genetic terms? The first of these questions (when?) recognises that different parts of a plant, at different times, might be channelled towards being male versus female, and implies that selection in a given lineage will often optimise a distribution of decisions among modules in the plant. The second question (who?) is prompted by the fact that the life cycle of all land plants involves an alteration of generations between haploid gametophytes and diploid sporophytes, and that one of these stages might take full or partial control of sex determination. The third question (how?) refers to the developmental genetic means by which sex is determined in a given lineage, with the subtext that there might be some sort of general answer we might hope to find from a survey among different lineages. In this Mini review, I address these questions by taking an evolutionary perspective --- we should expect sex to be determined in ways influenced both by a lineage's phylogenetic history, and by the action of selection on its populations' sex ratio or sex-allocation strategies, ultimately by modifying suitable gene networks. For conceptual reasons, I take a broad view of what we mean by 'sex determination'. Developmental biologists tend to use the term 'sex determination' when referring to the way in which male and female flowers develop in monoecious plants such as maize or melon. In contrast, geneticists tend to think of sex determination as the result of a genetic polymorphism (e.g., in species with sex chromosomes) that gives rise to the development of males or females in dioecious species, often initially through male- or female-sterility mutations. In this genetic sense, the term sex determination would not apply to monoecious species at all, because all individuals in a population could be genetically identical at loci determining sex-organ development. Although it is useful to remain alert to a possible distinction in meaning between genetic versus developmental sex determination, a key point is that the two meanings are not, in fact, as distinct as they might appear to be. As I discuss below, evolutionary transitions have frequently taken place between dioecy and monoecy, with the possibility that genes involved in developmental sex determination in monoecious populations may play a role in genetic sex determination in dioecious populations --- although this is yet to be established. Moreover, fully monoecious populations can simultaneously be genetically dimorphic, with a genetic class of hermaphrodites that emphasise their male function (without being exclusively male), and a second class of hermaphrodites that emphasise their female function. For these reasons, I blur the distinction between 'genetic' and 'developmental' definitions of sex determination, and consider them here together. The topic of plant sex determination has been reviewed in substantial detail in recent years. An important take-home message is that sex determination in plants is eclectic and varies a great deal among lineages. The contrast with animals is striking. Whereas much the same gene networks are deployed among highly divergent animals, the gene networks.** **Figure 1. Relationships between main clades and grades of land plants.** Determining sex even among angiosperms, which have diverged much more recently, appear to be very diverse indeed. Consideration of the nature of plant sexuality, as well as the history of transitions between sexual systems, can help to comprehend this diversity. In this Minireview, I begin with an overview of a few salient features of sex determination in the major land-plant lineages (Figure 1). I then consider what we might infer about plant sex determination from the fact that so many plants are hermaphroditic. Functional hermaphroditism in flowering plants takes a number of forms, ranging from the case where all flowers are 'perfect', with both male and female functions in each flower, to 'monoecy', where individuals are hermaphroditic but each f lower only develops functional male (stamens) or female organs (carpels). Much can be learned about sex determination in plants by comparing monoecy and dioecy. In both of these sexual systems, flowers are either male(staminate) or female(pistillate), but they differ in whether both sexes can be found in the same genetic individuals (monoecy) or only in separate genetic individuals (dioecy). Finally, I reflect on the implications for our understanding of sex determination of the fact that plant gender is very often a highly plastic, quantitative trait **Sex Determination in the Major Land-plant Lineages** The evolution of sexual reproduction constituted a major transition in the evolution of life, and occurred well before plants first ventured onto land some 470 million years ago. By that time, the ancestors of land plants already had differentiated male and female gametes (spermatozoids and eggs), and different sex organs to produce them (archegonia and antheridia). The first land plants had also already evolved an alteration of haploid and diploid generations (the gametophytes and sporophytes). In all land plants, the differentiation of sex organs into egg- or sperm-producing tissues occurs in the haploid gametophytic phase. However, which phase has control over the decision, and how the decision is enacted, varies. For an overview of sex determination in land plants, it is convenient to categorise their diversity into three groups --- a nonvascular group, including mosses, liver worts and hornworts ('bryophytes' in the broad sense); the vascular non-seed producing group, including lycophytes and monilophytes; and the clade of (vascular) seed plants, including extant gymnosperms and flowering plants (angiosperms;Figure 1). In non-seed plants, gametophytes may be male or female (in 'dioicous') species, with separate individuals producing either antheridia or archegonia, or they may be hermaphroditic (in 'monoicous' species), with both antheridia and archegonia present in the same individuals. Here, sex determination is a matter of the differentiation of cells and tissues in different parts of the same individuals. In dioicous species, separate male and female gametophytes germinate from spores of the same size. Sexual dimorphism nevertheless can be extreme. In some cases, dwarf male gametophytes grow on larger female gametophytes. In many bryophytes, sex is determined in gametophytes by (U and V) sex chromosome In ferns and lycophytes, the role and target of sex determination differ between 'homosporous' species, in which sporophytes all produce spores of the same size (as in bryophytes), and 'heterosporous' species, in which sporophytes produce both small 'microspores' and larger 'megaspores'. In some homosporous ferns, gametophytes are all functionally hermaphroditic, with both antheridia and archegonia. In these cases, sex determination is a question of cellular and tissue differentiation into different male and female 'gametangia'. In other homosporous species, gametophytes may develop a unisexual function (producing either only antheridia or only archegonia). Here, the sex-determination pathway targets separate, individual, gametophytes. However, the gametophytes differentiate in response to environmental cues perceived soon after spore germination (Figure 2A), rather than in response to a genetic polymorphism, as in some mosses mentioned above. Indeed, to my knowledge, sex chromosomes have not been described for any ferns or lycophytes \[6\]. In 'heterosporous' ferns and lycophytes, sex determination acts through the size of the spore from which the gametophytes germinate. The gametophytes do not differ genetically, and the sporophyte controls the sex of its gametophytes by regulating the spore-producing 'sporangia' to produce either small 'micro spores', which develop into male (micro-) gametophytes, or larger 'megaspores', which develop into female(mega-)gametophytes. Heterospory has evolved several times in vascular land plants, in ferns and lycophytes, as well as independently in the lineage that gave rise to seed plants \[14\]. In angiosperms (flowering plants) and gymnosperms, the gametophytic phase has become even more highly modified and reduced, and differentiation between the male and female structures takes place in sporophytic structures in which the gametophytes form. In extant seed plants, the role of sex determination is thus passed from the gametophytes entirely to their diploid sporophyte 'hosts'. Seed-plant sporophytes may ultimately be functionally hermaphroditic, producing both pollen (modified and highly reduced male gametophytes) and ovules (which contain modified female gametophytes), or they may be male or female, producing only pollen or ovules. In contrast to non-seed plants, seed-plant gametophytes are always either male or female, and it is the sporophyte that determines their sex. The developmental machinery of sex determination in seed plants therefore governs which gametophytes a given diploid sporophyte individual produces, where on the plant they will occur, and in which proportions.  **Figure 2. Three contrasting sex-determining pathways in land plants.** Black and grey text and symbols indicate genes and functions switched on and off, respectively. Arrows and 'flat-ended' arrows indicate promotion or suppression of activity, respectively. (A) Environmental determination of sex in the homosporous fern Ceratopteris richardii. Sex determination depends on the outcome of two antagonistically interacting genes, FEM1 and TRA: in the absence of an exogenous signal from the hormone antheridiogen, the gene TRA is expressed; this both elicits the development of a female (or her maphrodite) gametophyte, and suppresses the expression of FEM1 through an intermediary MAN1. In the presence of exogenous antheridiogen, the gene HER is expressed; HER suppresses the expression of TRA (and, in turn, of MAN1), allowing the expression of FEM1. FEM1 promotes both the develop ment of a male gametophyte and the expression of the gene NOT1, which in turn suppresses TRA. Images courtesy of Jody Banks. (B) Chromosomal determination of sex in persimmons. In XY individuals (males), expression of OGI, a small RNA, targets and suppresses a second autosomal gene MeGI, a transcription factor that suppresses stamen development. In XX individuals, which lack OGI, MeGI is expressed, suppressing stamen development. Images courtesy of Jeff Pippen. (C) Sex determination in monoecious melons. **Figure 3. The quantitative (and potentially plastic) nature of sex determination in plants.** A. Plots of the proportion of female flowers in two dioecious species, taken from the work of D. Lloyd (republished with permission from John Wiley and Sons, Inc., \[50\]), who first emphasized the importance of quantitative gender in plants. In both plots, the distribution of gender is plotted as the relative rank gender of individuals sampled in the population, with closed and open circles representing male and female plants, respectively. Left panel: Gingidia montana; right panel: Leptinella dendyi. B. Chromosomal sex determination in the plant Mercurialis annua, showing an XX female (left), an XY males (centre), and an XY male with 'leaky' gender expression, i.e., a male producing both male and female flowers and fruits. Blue arrows indicate male (staminate) flowers; red arrows indicate female (pistillate) flowers. Images courtesy of JohnBaker(left and centre right),Guillaume Cossard (centre left) and Xinji Li (right) determination have thus evolved many times independently. But generalisation is nonetheless possible if we consider how transitions to dioecy have likely occurred. First, dioecy must evolve through the positive selection of both male and female sterility mutations in what are destined to become the sex-determination genes. Theory predicts that male-sterility mutations are most likely to spread in hermaphrodite populations first, largely because they prevent self-fertilizing, and thus from producing progeny that might suffer inbreeding depression, but also because it is difficult for female-sterility mutations to spread in partially selfing populations. The 'gynodioecious' population that results may then evolve towards full dioecy, for example, in response to selection for sexual specialisation, either with the subsequent spread of a female-sterility mutation, or through the gradual shift in sex allocation of non-male-sterile hermaphrodites towards greater maleness (the production of fewer seeds and more male flowers or pollen) The two likely alternative paths from gynodioecy to dioecy have important implications for how sex might be determined. In the case of the spread of a female-sterility mutation in a gyno dioecious population, the gene affected must become tightly linked to the male-sterility mutation on a single (sex) chromo some, because otherwise segregation or recombination could create genotypes that carry and express both male and female sterility at the same time. Alternatively, the female-sterility mutation could show epistatic expression, masculinising only those individuals not expressing the male-sterility mutation. If male sterility is recessive, as is most probable for a loss-of-function mutation, females should be homozygous (and thus XX in the case of a sex chromo some), and hermaphrodites or males should be heterozygous (and thus XY, with female sterility or male enhancement genes being located on the Y). Little is known about the genes that cause male and female sterility in dioecious plants, but it seems that genetic linkage between them on a sex chromo some is common, as exemplified by recent work on white campion(Silenelatifolia), wild strawberry (Fragaria virginiana) and broadleaf arrowhead (Sagittaria latifolia). Papaya (Carica papaya) also has an XY sex-chromosome system, but a modified Y h chromosome is also present, and that fails to sup press the individual's female function, allowing hermaphrodites to develop, and suggesting that the normal Y in papaya males indeed harbours a female suppressor. Modified Y chromo somes are similarly known in Silene latifolia and Vitis vinifera. In the annual mercury (Mercurialis annua), females have no Y chromosome ,and have acquired a male function in some populations (Figure 3B), suggesting either that male-sterility mutations on an X chromosome have incomplete expression in these individuals, or that there are loci elsewhere in the genome that can partially restore male function in females. The two-locus model for the evolution of sex determination and dioecy makes intuitive sense and has received some support , but sex is determined in many species by a single switch gene. In Caucasian persimmon (Diospyros lotus), for example, the anther fertility is determined by transcription of a Y-linked sequence encoding a small RNA that targets and sup presses expression of an unlinked autosomal feminizing gene (Figure 2B). In other words, although two loci are involved at the top of the sex-determination cascade, they are unlinked to one another, and only one of them now acts as the switch gene in the gene network. It is not yet known how such develop mental gene networks have been assembled during the evolution of dioecy. For example, did they evolve through the initial spread of (linked) male- and female-sterility mutations, as supposed by theory ? Or might they have evolved through the spread of a single sex-determining gene distinguishing between females and hermaphrodites, say, followed by the fixation of mutations expressed only in hermaphrodites that reduce the female allocation of the hermaphrodites? The plausibility of single-locus sex determination in some species is also suggested by the ontogeny of male and female sterility. In their review, Diggle et al. noted that stamen abortion in a given species tends to occur at approximately the same time as carpel abortion. This pattern is not what we would immediately expect if sexual organ abortion results from the expression of independent male- and female-sterility mutations, unless it reflects a wide distribution of times since the evolution of dioecy in the respective lineages. Alternatively, the pattern hints at the possibility that a single underlying switch acts within a gene network to suppress one or the other sexual function at about the same time of floral differentiation. Such a scenario has been elucidated in monoecious melons, although again it is by no means clear how it evolved. In melons, and in maize, the sexual identity of flowers appears to result from the expression of genes in different networks that are responsive to positional information mediated by hormones. In melons, gene expression at one locus causes suppression of carpel development (female function), whereas expression at a different locus suppresses stamen development (the male function). Expression of an upstream gene that is thought to be sensitive to local ethylene levels determines which of those two genes is switched on (Figure 2C). In maize, another monoecious species, different mutant classes have been identified that suppress stamen or carpel development in female (ears) or male inflorescences (tassels), respectively. Just as male and female flowers in melons tend to develop in different parts of the plant, ears and tassels in maize develop in leaf axils and at the plant apex, respectively; the gender distribution within the plant is responsive to gradients in plant hormone levels, and con forms to adaptive expectations for a wind-pollinated plant. **The Quantitative and Plastic Nature of Plant Sex Determination** Plant gender is fundamentally a quantitative trait. Whereas individuals in most gonochoristic animals are either fully male or female, gender in plants is often better viewed on a quantitative scale, measured in terms of the relative allocation to each sexual function, or the proportion of genes transmitted through sperm versus eggs (Figure 3A). Many dioecious species, including those with well-developed sex chromosomes, show 'leaky' or 'inconstant' gender expression, with males and females producing flowers of the other gender. Far from being an aberrant feature of plant reproduction, such phenotypes are typically fully functional for both sexes (e.g., Figure 3B). The quantitative distribution of gender in a population is under strong selection and can evolve rapidly when circumstances change --- for example, when males are lost from a population. Such shifts in sex allocation can occur at any level in the modular hier archy of plant development, from the number of pollen grains produced in anthers, to the number of floral or inflorescence primordia that develop with male versus female functions. Such variation allows for multiple pathways to sex differentiation and potentially sex determination (Figure 3).\ While the distribution of quantitative gender in a population may have a strong genetic component, individuals can also modify their sex expression in response to context-dependent opportunities or costs. Plastic sex expression applies not only to the quantitative nature of sex allocation in functional her maphrodites, but, more rarely, to complete sex change. In jack-in-the-pulpit (Arisaema species), young plants are fully male, whereas, in later seasons, they become fully female or her maphroditic. Such 'sexual diphasy' is expected to evolve both if the marginal benefits of reproducing as male versus female change with plant size, and if there are additional benefits to sexual specialisation at a particular point in time (e.g., if fitness is compromised by interference between simultaneous male and female functions).Plasticity in sex allocation, and sexual diphasy specifically, indicate that sex may be determined not by a genetic dimorphism, but by the differential expression of genes shared by all individuals in a population in response to physiological and environmental cues. Sex in jack-in-the-pulpit is likely to be responsive to intrinsic physiological signals reporting the resource status of the plant, but also to exogenous cues. Such responses have been documented for many angiosperm species, although angiosperms do not seem to have evolved fully environmental sex determination (ESD) that might parallel, for example, temperature-dependent sex determination in some reptiles. In contrast, some homosporous ferns do show true ESD.When the haploid spores of the fern Ceratopteris richardii germinate, for example, they develop into either male or female gametophytes, depending on the presence of potential mates(Figure2A).In the absence of mates, gametophytes produce both antheridia and archegonia. They also release into the water around them the hormone antheridiogen, which causes nearby gametophytes to develop as miniature males. Some of the underlying genes involved in the switch between hermaphroditic and male pathways have been identified. Mutations at these loci render gametophytes insensitive to antheridiogen, and their sex is thus determined genetically by the mutations they carry (Figure 2A). In principle, the spread of such mutations in a natural population could conceivably convert ESD into genetic sex determination(GSD).In monoecious melons cited above, or in maize, in which the sex of individual flowers or inflorescences depends on their location in the plant, genetic manipulation of mutants can render a population effectively dioecious in similar ways, that is, experimental manipulation can convert a type of hormone-sensitive, tissue-specific ESD into GSD. Such examples show that ESD and GST may be readily interconverted**.** **Conclusions** Much has been learned about how sex is determined in a handful of plant species. Although detailed analysis of a wider taxonomic sample will no doubt be revealing, it is already becoming clear that different gene networks probably determine sex in different monoecious and dioecious lineages, and that generalisations are thus unlikely to emerge in terms of the classes of genes involved. However, the very diversity of sex-determination mechanisms in plants reflects the important fact that separate sexes tend to have evolved independently in different lineages, and much more recently than in most animal lineages. This diversity should not surprise us. But are there any general patterns in how gene networks come to be assembled that involve the co-ordination of both male-and female-sterility mutations, often in different parts of the plant organism? The two-locus model for the evolution of sex chromosomes and sex determination is satisfying in its potential generality and logical coherence. In contrast, we have no similarly general proposition for how single-locus sex switches become embedded in necessarily complex networks. For instance, do dioecious species employ the same developmental pathways as the monoecious species from which they are derived? Ad dressing these questions should be a major goal for future research, for example, through a comparative analysis of gene networks in related species that express their gender differently. The questions of when and where on a plant which sex is expressed has long been accessible through analysis of competing allocation strategies in an evolutionary framework. The theoretical underpinnings for such an understanding are well-estab lished, and observed patterns of sex expression and sex allocation often conform nicely to expectations-- though many puzzles remain. Because sex determination directly affects an important currency of fitness(reproductive success),it is not surprising that its responses to selection can be rapid and dramatic. An interesting question that deserves further attention here concerns shifts between plasticity and genetic polymorphism. How often plasticity in sex expression acts as a capacitor for the evolution of genetically separate sexes is not known, but the facility with which these modes of sex determination can be interconverted experimentally suggests that they do not reflect fundamental evolutionary constraints. Nonetheless, it remains puzzling as to why a chromosomal polymorphism that determines sex in the gametophytes of bryophytes has never re placed environmental sex determination in ferns or lycophytes. It is tempting to think that the distinction is linked to the ephemeral nature of fern but not bryophyte gametophytes \[6\], but why there should be such a link is not obvious. **REFERENCES** - [**https://pubmed.ncbi.nlm.nih.gov/**](https://pubmed.ncbi.nlm.nih.gov/) - [**https://onlinelibrary.wiley.com**](https://onlinelibrary.wiley.com) - [**https://aspb.org**](https://aspb.org)