Cell Biology of Intracellular Infection by Legionella pneumophila PDF
Document Details
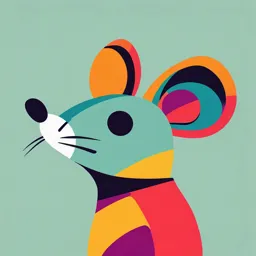
Uploaded by HonestChlorine9195
Koç University
Maëlle Molmeret, Dina M. Bitar, Lihui Han, Yousef Abu Kwaik
Tags
Summary
This review article examines the cell biology of intracellular infection by Legionella pneumophila, a pathogen linked to Legionnaires' disease. It explores the bacterium's unique ability to modify host cell biology and create a favorable environment for intracellular reproduction. The article discusses growth phase-regulated virulence and the critical role of the Dot/Icm secretion system.
Full Transcript
Microbes and Infection 6 (2004) 129–139 www.elsevier.com/locate/micinf Review Cell biology of the intracel...
Microbes and Infection 6 (2004) 129–139 www.elsevier.com/locate/micinf Review Cell biology of the intracellular infection by Legionella pneumophila Maëlle Molmeret a, Dina M. Bitar b,c, Lihui Han b,d, Yousef Abu Kwaik a,* a Department of Microbiology and Immunology, University of Louisville College of Medicine, Louisville, KY 40292, USA b Department of Microbiology and Immunology, University of Kentucky College of Medicine, Lexington, KY 40536, USA c Department of Medical Microbiology and Immunology, Faculty of Medicine, Al-Quds University, Jerusalem, 19356, Israel d Department of Microbiology and Immunology, College of Medicine, Shandong University, Shandong, Jinan 250012, China Abstract Legionella pneumophila has become a paradigm for facultative intracellular pathogens that modulate biogenesis of their phagosomes into replicative niches. The ability to alter host cell biology and tailor it into a hospitable host for intracellular proliferation is at the crux of the mechanism of pathogenesis of Legionnaires’ disease. © 2003 Elsevier SAS. All rights reserved. Keywords: Intracellular; Dot/icm; Secretion 1. Introduction gal transfer of DNA, which has been confirmed by conjuga- tion studies [9,10]. This apparatus has also been shown to be Legionella pneumophila, a Gram-negative bacillus ubiq- involved in proper maturation of the L. pneumophila- uitous in aquatic environments, is responsible for Legion- containing phagosome in mammalian and protozoan cells, naires’ disease. It is a facultative intracellular pathogen that directing the biogenesis of the specialized vacuole in which can replicate within eukaryotic host cells such as protozoa Legionella replicates [11–13]. The dot/icm genes are also and macrophages. In humans, L. pneumophila reaches the required for macropinocytosis in A/J mouse macrophages lungs after inhalation of contaminated aerosol droplets [1,2] , upregulation of phagocytosis in human-derived mac- (Fig. 1). Once in the lungs, L. pneumophila is ingested by rophages , induction of apoptosis in macrophages alveolar macrophages, which are the major site of bacterial [16–18] and pore formation-mediated cytotoxicity in both replication. This results in an acute and severe pneumonia, protozoan and mammalian cells [13,19–21]. Legionnaires’ disease. The unique intracellular fate of L. pneumophila is one of the interesting aspects of this organism. Unlike phagosomes 2. Growth phase-regulated virulence containing inert particles or avirulent bacteria, the L. pneumophila-containing vacuoles avoid the “default” en- In contrast to exponentially growing bacteria, L. pneumo- docytic pathway, recruiting rough endoplasmic reticulum phila obtained from post-exponential cultures expresses (RER) and mitochondria, to reside in a specialized vacuole traits that are correlated with virulence, including sodium allowing intracellular replication [3–7] (Fig. 2). The forma- sensitivity, cytotoxicity, osmotic resistance, motility, and the tion of this specialized vacuole is directed by the type IV capacity to evade phagosome–lysosome fusion [22–25]. secretion system encoded by the dot/icm genes. The dot During the replication phase within host cells, L. pneumo- (defect in organelle trafficking)/icm (intracellular multiplica- phila is sodium resistant and aflagellated [22–25]. When L. tion) loci consist of 23 genes located in two distinct regions pneumophila egresses from host cells, the bacteria are flag- of the L. pneumophila chromosome [8–10]. Analysis of the ellated and sodium sensitive [22–25]. It has been hypoth- predicted amino acid sequences of the dot/icm genes has esized that amino acid limitation in vitro induces the virulent revealed several characteristics that indicate a role in conju- phenotype [22–25]. When L. pneumophila enters into post- exponential growth phase or is subjected to amino acid limi- * Corresponding author. Tel.: +1-502-852-5351; fax: +1-502-852-7531. tation, the bacteria accumulate the stringent response signal, E-mail address: [email protected] (Y.A. Kwaik). guanosine 3′,5′-bispyrophosphate (ppGpp) through the © 2003 Elsevier SAS. All rights reserved. doi:10.1016/j.micinf.2003.11.004 130 M. Molmeret et al. / Microbes and Infection 6 (2004) 129–139 Fig. 1. The environmental life of L. pneumophila within protozoa. (1) L. pneumophila from biofilms with other bacteria, or in suspension, infecting protozoa. (2) Following entry, L. pneumophila resides in a membrane-bound vacuole that recruits host cell organelles, such as the mitochondria and the RER, and does not fuse with lysosomes. (3) Mutants such as dot/icm mutants fuse to lysosomes 3b. L. pneumophila replicates within specialized vacuoles and reaches large numbers 3a. (4) The infectious particle is not known, but may include excreted legionella-filled vesicles, intact legionella-filled amoebae, or free legionellae that have lysed their host cell. (5) Transmission to humans occurs via mechanical means, such as faucets and showerheads. Infection in humans occurs by inhalation of the infectious particle and establishment of infection in the lungs. (6) Legionellae that have escaped their host cell may survive in suspension for long periods of time, reinfect other protozoa, or recolonize biofilms. Reprinted from ASM News. 66 (10) (2000) 609–616. M. Molmeret et al. / Microbes and Infection 6 (2004) 129–139 131 infection in this amoebae model. L. pneumophila in post-exponential phase becomes cytotoxic by an RpoS- independent pathway. To replicate efficiently in mac- rophages, both RpoS-independent and -dependent mecha- nisms are utilized by L. pneumophila. Thus, when nutrient levels and other conditions are favorable, L. pneu- mophila replicates within host cells, and when amino acids become rare, intracellular bacteria express several traits that permit escape from the host cell, survival in the environment and the transmission to a new host. The relA mutant, producing an undetectable level of ppGpp in the cells during stationary phase, is unable to produce pigment as it becomes flagellated. Although a previ- ous study has shown that RpoS, which accumulates when RelA is activated, is required for intracellular growth in A. castellanii , Zusman et al. have shown that the relA gene product is dispensable for intracellular growth in HL-60-derived human macrophages and in A. castellanii. Moreover, it has also been shown that RelA and RpoS have minor effects on expression of some of the dot/icm genes (icmT, R, Q, P, M, J, F, V, W). Thus, the role of RpoS in the intracellular infection seems to be host cell specific. 3. Adherence and entry into mammalian cells Invasion and intracellular replication of L. pneumophila Fig. 2. Transmission electron micrographs of the infection of A. polyphaga within pulmonary cells in the alveoli are the hallmark of (top panel) and U937 macrophages (bottom panel) by L. pneumophila. Legionnaires’ disease. The alveolar wall is composed of Coiling phagocytosis (A and B); formation of the RER-surrounded phago- macrophages and type I and type II epithelial cells. The some (C and D); and late stages of the infection (E and F). Note that in E and F, there is no intact phagosomal membrane. Reprinted from ASM News. 66 attachment of L. pneumophila in macrophages has been (10) (2000) 609–616. shown to be mediated, at least in part, through attachment of complement-coated bacteria to the complement receptor ppGpp synthetase, RelA. When relA from Escherichia , although non-complement-mediated uptake also occurs coli is expressed in L. pneumophila, ppGpp accumulates and [32,33]. The host cell receptor involved in non-complement- the bacteria express virulent traits independently of nutrient mediated uptake in macrophages and epithelial cells is not supply or cell density. The accumulation of ppGpp known. increases the amount of the stationary-phase sigma factor Uptake of L. pneumophila by monocytes and macro- RpoS, which triggers the expression of the stationary-phase phages has been shown to occur through conventional and genes. An rpoS mutant of L. pneumophila replicates coiling phagocytosis [6,32,34–36] (Fig. 2). Since heat-killed within HL60 and THP-1 monocytic cell lines, but is attenu- and formalin-killed L. pneumophila are also taken up by ated in Acanthamoeba castellanii. In bone marrow- coiling phagocytosis but are targeted to the lysosomes , derived macrophages from A/J mice, L. pneumophila rpoS this mode of uptake may not play a role in subsequent mutants replicate poorly because they traffic rapidly to a late trafficking of the bacteria. Many clinical isolates of L. pneu- endosome-like compartment. However, Bachman et al. mophila have been shown to be taken up exclusively by used a different L. pneumophila strain, Lp02, whereas Hales conventional phagocytosis [32,34]. In addition, other species et al. used JR32. It has been shown that some genotypic and of legionellae, such as L. micdadei, which is the second most phenotypic differences exist between the AA100, JR32 and common species of legionellae that causes Legionnaires’ Lp01 stains, which are the most commonly used in the disease, is taken up exclusively by conventional phagocytosis virulence studies. AA100 is clearly the most virulent,. The bacterial ligand that mediates the coiling mode of whereas Lp01 is the least. Therefore, the differences between phagocytosis is not known. Moreover, the phagocytic recep- L. pneumophila strains and/or between the host cells may tor that binds the bacteria seems to play some role in deter- explain the different intracellular growth observed for the mining the fate of the intracellular bacteria, since opsoniza- rpoS mutants. Sodium sensitivity and maximal expression of tion with antibodies reduces intracellular growth [31,37,38]. flagellin also require RpoS. Therefore, some RpoS- Studies have focused on the genetic aspects of the uptake regulated traits could be critical for efficient transmission or of L. pneumophila into its host cells. L. pneumophila mutants 132 M. Molmeret et al. / Microbes and Infection 6 (2004) 129–139 impaired in different loci, such as rtxA and enhC, display tion system is ancestrally related to type IV secretion systems significantly reduced entry into host cells, compared with that mediate conjugal DNA transfer between bacteria , L. wild-type bacteria. It has been shown recently that the pneumophila may utilize this transporter to transfer macro- enhanced phagocytosis of L. pneumophila by mammalian molecules into the host cell to evade endocytic fusion [8,10]. cells is dot/icm dependent. Interestingly, the dot/icm The dot/icm loci may be involved in the insertion of a pore in genes delay uptake and induce macropinocytosis in A/J the host cellular membrane through which the effector pro- mouse macrophages. With the exception of A/J mice, teins are exported into the host cell [52,53]. The effector most of the inbred mouse strains are not permissive to L. molecules involved in intracellular trafficking and evasion of pneumophila infection; neither are macrophages isolated the lysosomal fusion within mammalian cells are cis-acting from these mice [40,41]. Macropinosomes containing L. on the phagosome but do not alter endocytic fusion in the rest pneumophila in A/J mouse macrophages are induced tran- of the cell. With few exceptions, the function of indi- siently and shrink rapidly (5–15 min) , and this mode of vidual Dot/Icm proteins is unknown. uptake is linked to the lgn1 locus on chromosome 13 of mice The DotA protein was the first to be described. It is a [14,42,43]. In macrophages of non-permissive strains of polytopic inner membrane protein with eight hydrophobic mice, the macropinocytic uptake of L. pneumophila is re- transmembrane domains. The dotA mutants are defec- duced. The lgn1 allele makes the bacteria behave as if tive in all virulence activities that require the Dot/Icm com- they were lacking the dot/icm system. Thus, the lgn1 plex [12,45,53,55,57,58]. These data are supported by the allele is required for dot/icm-dependent macropinocytosis fact that the DotA sequence possesses significant similarities and delayed uptake by mouse macrophages. Whether to that of TraY [59,60], a component of the type IV trans- this mode of uptake plays a role in subsequent trafficking of porter required for conjugal transfer of plasmids ColIbP9 and L. pneumophila is not known. R64. However, Nagai and Roy have shown that the DotA protein is also secreted through the Dot/Icm transporter into the culture supernatant during growth of L. pneumophila 4. Intracellular fate of L. pneumophila in liquid broth via a functional type IV secretion system. Electron micrographs also show that purified DotA can form 4.1. Intracellular replication within host cells an oligomer [56,61]. Similarly to the protozoan infection, within 5 min follow- As DotH/IcmK and DotO/IcmB in growing L. pneumo- ing entry of the bacteria into macrophages and monocytes, phila cultures are mainly associated with the membranous the L. pneumophila phagosome is surrounded by host cell fraction, and as dot/icm products may be required during organelles such as mitochondria, vesicles, and the RER direct contact with host cells, the location of DotH and DotO [5,44,45] (Fig. 2). Also similarly to the trafficking of L. proteins on the surface of L. pneumophila has been examined pneumophila within protozoa , it has been shown that the. These proteins are surface exposed and associated with phagosome within mammalian macrophages does not fuse to a fibrous structure on L. pneumophila after exposure to bone lysosomes [4,6,7] (Fig. 2), at least at the early stage of the marrow-derived macrophages. In contrast, during broth cul- infection. The role of the RER in the intracellular infec- ture, this fibrous structure is absent. However, using tion is not known, but the RER is not required as a source of dotA, dotB, dotH, dotO mutants, it has been shown that the proteins for the bacteria. Interestingly, examination of exposure of DotO/DotH on the bacterial surface is not depen- the intracellular infection of macrophages, alveolar epithelial dent on other Dot/Icm proteins, including the DotH protein cells, and protozoa by another Legionella species, L. micda- for the DotO exposure, and vice versa. This result shows dei, showed that within all of these host cells, the bacteria that the surface exposure of DotH and DotO after contact were localized to RER-free phagosomes. Whether other with macrophages is not dependent on an intact Dot/Icm Legionella species replicate within RER-free phagosomes secretion system. The surface exposure of these two has still to be determined. proteins does not involve bacterial contact with the target Macrophages, peripheral blood monocytes, and alveolar cell, since bacteria incubated in medium that has been condi- epithelial cells support intracellular replication of L. pneu- tioned by bone marrow-derived macrophages for 24 h yield mophila [37,50]. Although alveolar epithelial cells, which almost identical results. In addition, DotH and DotO constitute more than 95% of the alveolar surface , have surface exposure on L. pneumophila requires intracellular been shown to allow intracellular replication of L. pneumo- growth of the bacteria in macrophages and is observed late in phila, their role in the pathogenesis has been largely over- the infection process, mostly when there are more than looked. 30 bacteria per phagosome. In fact, surface-exposed DotH and DotO disappear after uptake but reappear follow- 4.2. The dot/icm genes and their role in evasion of vesicle ing intracellular growth. The exposure of these two proteins traffıc increases L. pneumophila uptake into cells and may be necessary to promote bacterial escape from the phagosome The Dot/Icm type IV secretion system is the main L. and to facilitate the initiation of a new infection in macro- pneumophila virulence system. Because the Dot/Icm secre- phages. These data may also suggest that macrophage M. Molmeret et al. / Microbes and Infection 6 (2004) 129–139 133 components are able to induce changes in the L. pneumo- Using fluorescent markers specific for the ER, it has been phila envelope, and DotH/DotO export may occur as a re- shown that the L. pneumophila-containing vacuoles may sponse to the target macrophages just before uptake to allow resemble nascent autophagosomes. Autophagy is a cel- efficient initiation of intracellular growth. lular process for the degradation of unwanted organelles and Dot/Icm proteins such as IcmR, IcmQ, IcmX or IcmW do cellular components during which the autophagosome is not present sequence homology to other protein components formed from the smooth ER and fuses to lysosomes. The of type IV secretion system [9,10,51]. IcmX, a periplasmic hypothesis that L. pneumophila exploits the autophagy ma- protein, is required for biogenesis of the replicative phago- chinery in host cells and establishes an intracellular niche somes containing L. pneumophila. A truncated IcmX favorable for replication has been challenged recently product is secreted into culture supernatants by wild-type L. (see below) [44,64]. pneumophila growing in liquid media, but the transport of the Recent studies suggest that fusion or the exchange of protein into eukaryotic host cells has not been detected. lipid bilayer with ER vesicles on the L. pneumophila- IcmS and IcmW are small proteins required for the traf- containing phagosome occurs, allowing the phagosomal ficking of the Legionella-containing phagosome, and may membrane to become as thin as the ER membrane, with function as chaperones to help the secretion of proteins similar characteristics [44,64]. It has been shown that, within through the type IV secretion system [58,61]. It has also been 5 min of uptake, host vesicles come into contact with wild- shown that IcmS and IcmW interact, suggesting that they type Legionella-containing phagosomes and flatten along the may be components of a protein complex that is required for surface of the phagosome, and this process is completed modulating phagosome biogenesis. The phagosomes of within 15 min and is dot/icm dependent. This is the icmS and icmW mutants fuse to lysosomes. Interest- consistent with earlier studies that have shown that after 4 h ingly, phagosomes harboring icmS and icmW mutants still of infection, there are only a few vesicles associated with the recruit host vesicles including the RER. It is still to be phagosomal membrane, but there are ribosomes studding the confirmed whether recruitment of host vesicles does not phagosomal membrane. In contrast, autophagy takes prevent the phagosome from lysosomal fusion. place within 1 h, and ribosomes, mitochondria, and the The IcmR protein may also be a chaperone. An icmR nuclear membrane are not attached to the autophagosomal mutant can partially evade the endocytic pathway, but even- membrane; neither are the tiny hairs that connect ER and L. tually fuses with the lysosomes. These data suggest that pneumophila-containing phagosomes. Interestingly, the IcmR may be a co-factor for another protein effector in- thickness of the phagosomal membrane becomes similar to volved in evasion of lysosomal fusion. The icmR mutant that of the ER membrane within 15 min , which doesn’t that evades endocytic fusion does not recruit host vesicles, happen in the autophagy process. Thus, within 15 min of and the phagosome is not surrounded by the RER. Thus, infection, the phagosomal membrane resembles that of the effector molecules that recruit host cell vesicles may be ER. The ribosomes at 6 h stud the phagosomal membrane, different from the ones involved in evasion of the endocytic and L. pneumophila is thought to be located within the RER pathway.. However, these studies rely completely on the thickness Similarly to dotA and icmX mutants, an icmQ mutant is of the membranes of the ER and the phagosome membranes defective in all virulence activities. Furthermore, the to provide evidence that L. pneumophila is located within the icmQ gene, like the icmR and icmS genes, encodes soluble RER. Immunocytochemical studies should be per- protein. The IcmR and IcmQ proteins interact as protein formed to confirm these observations. It is likely that the chaperone-substrate. The presence of IcmR that has chaper- recruitment of the ER may be involved in the biogenesis of one characteristics (it is acidic, small and predicted to have a the phagosome that is dependent on the type IV secretion hydrophobic alpha-helix in its C-terminal domain) affects system, since the dot/icm mutants are unable to recruit RER, the physical state of IcmQ directly. IcmR prevents IcmQ and their phagosomes fuse to the lysosomes. from participating in the formation of high-molecular-weight complexes by dissociating IcmQ homopolymers. Interestingly, a recent study showed that recruitment of RER to forming-phagosomes may be part of regular phago- 4.3. Recruitment of RER cytosis. Electron micrographs of latex beads and patho- gens such as Salmonella within macrophages have shown In 1982 Katz and Hashemi showed that the L. that ER membranes fuse with plasmalemma, underneath the pneumophila-containing phagosome resembles the ER phagocytic cup, and successive waves of ER are recruited to membrane. By electron microscopy, replicating L. pneumo- the phagosomes of latex beads and bacteria. In neutrophils, phila within macrophages was visualized located within or- the bacteria are quickly killed, and the ER is not involved in ganelles morphologically identical with the RER the turnover of membrane for phagocytosis. However, (Fig. 2). Later, several studies demonstrated that upon inter- since the dot/icm system is essential for RER recruitment nalization of L. pneumophila by the host cell, the Legionella- , it is likely that L. pneumophila utilizes a specific containing vacuole recruits organelles such as vesicles, mito- pathogen-regulated process to recruit vesicles, and this pro- chondria and ER (Fig. 2) [5,46,66,67]. cess is distinct from regular phagocytosis. 134 M. Molmeret et al. / Microbes and Infection 6 (2004) 129–139 4.4. Interception of early vesicle traffıc from the RER The protein ADP ribosylation factor-1 (ARF1), a highly conserved small GTP-binding protein, which acts as a key regulator of vesicle traffic from ER to Golgi, is found on about 30% of the phagosomes that contain wild-type L. pneumophila but not dot/icm mutants. These data sug- gest that a protein injected through the type IV secretion system may be required for ARF1 recruitment. Searching the L. pneumophila genome for proteins that have homology to ARF-specific guanine nucleotide exchange factors (GEFs), Nagai et al. have identified a protein, RalF, that has a sec7-homology domain, known to be sufficient to stimulate the exchange of GDP for GTP. It has been shown that RalF is injected through the phagosomal membrane by a process that requires the Dot/Icm system. However, phagosomes containing ralF mutants that do not recruit ARF1 evade fusion to lysosomes, and the bacteria replicate intracellularly Fig. 3. The rib mutants’ defect in cytolysis of the host cell is due to a defect in necrosis-mediated killing. Representative transmission electron micro- within macrophages and amoebae. Thus, RalF is not graphs of infected U937 macrophages at 24 and 48 h post-infection by the essential for transport of L. pneumophila to the ER [64,70]. wild-type strain AA100 and the GN229 mutant. The original magnifications It has been shown recently that the L. pneumophila- were 7000× and 5000× for the 24- and 48-h infections, respectively. Reprin- containing phagosome is a transitional ER (tER)-derived ted from. organelle. Its biogenesis involves intercepting early secretory vesicles exiting from tER. Vesicular transport We have identified five spontaneous mutants that are un- from ER exit sites is not necessary for the recruitment of ARF able to egress from the macrophages but are able to grow as to L. pneumophila-containing phagosomes. The vesicular well as the wild-type strains within these cells. These transport from ER exit sites is an ARF-dependent process mutants, designated rib (release of intracellular bacteria), are used for remodeling the L. pneumophila-containing phago- defective in the pore-forming toxin/activity as shown by the some. The final stage of the biogenesis of the mature ER- contact-dependent hemolysis assay and by permeability to derived vacuole is marked by the loss of ARF and the accu- propidium iodide upon infection of macrophages and epithe- mulation of calnexin. Moreover, ralF mutants also mature in lial cells. The rib mutants are also defective in acute calnexin-positive RER-derived vacuole, similarly to the cytotoxic lethality to A/J mice and fail to cause alveolar wild-type strain. The RalF protein is not essential in phago- inflammation [13,20] indicating a key role for the pore- some transport, replicative organelle biogenesis or intracel- forming toxin in the pulmonary pathology of the bacterium. lular replication. Subversion of the early secretory path- We have further documented that the Rib toxin is not re- way is an effective way of gaining access to the ER lumen quired for intracellular trafficking and replication [19–21]. and exploiting translocation machinery of this environment In addition to defects in evasion of lysosomal fusion,. dot/icm mutants are also defective in induction of apoptosis , enhancement of phagocytosis by human-derived mac- 4.5. The “traffıcking” pore and the “egress” pore rophages and induction of macropinocytic delayed up- take by A/J mouse macrophages. In contrast, rib mutants Kirby et al. have described the pore-forming ability are defective in pore-forming toxin but are not defective in of L. pneumophila in host cell membranes. This was docu- evasion of lysosomal fusion, intracellular replication, or in- mented by lysis of macrophages and red blood cells, which is duction of apoptosis [18,20,21]. These observations may dependent on the Dot/Icm secretion system. Since suggest that there are at least two pores inserted into host dot/icm mutants are defective in evasion of lysosomal fusion, membranes through the type IV secretion system at different it has been proposed that the pore-forming activity is re- stages of the infection. The first pore is the “invasion and quired for export of effector molecules necessary for evasion trafficking pore”, which is inserted upon initial contact with of the lysosomal fusion. Upon initial contact with the the host cell to deliver effectors into the host cell cytoplasm host cell, L. pneumophila may insert a pore into the plasma and allow the establishment of the intracellular infection. The membrane to deliver bacterial effector molecules into the dot/icm genes necessary for the assembly of the secretion host cell [52,53]. This concept is supported by the fact that apparatus are constitutively expressed and are required for many dot/icm mutants are defective in both intracellular this step. The second pore is the “egress pore”, which is replication and pore formation-mediated cytotoxicity triggered during the late stages of intracellular replication [52,53]. Moreover, some dot/icm mutants are defective in and is essential for lysis of the host cell (Figs. 3 and 4). trafficking and intracellular replication but not in the pore- This is consistent with the fact that upon transition into the forming activity. post-exponential growth in vitro, L. pneumophila becomes M. Molmeret et al. / Microbes and Infection 6 (2004) 129–139 135 Fig. 4. A model of growth phase-dependent cytolysis of mammalian cells by L. pneumophila upon termination of intracellular bacterial replication to egress from the spent host cell. During early stages of formation of the mitochondria and RER-surrounded phagosome (A) and during exponential intracellular replication (B), expression of the pore-forming activity is turned off, but caspase-3-mediated apoptosis is triggered. Upon transition to the post-exponential phase of growth, expression of the pore-forming activity is triggered, which results in insertions of pores in the phagosomal membrane first (C), leading to its disruption (D). This is followed by insertions of pores in the plasma membrane (E), leading to osmotic lysis of the cell and release of the intracellular bacteria. Reprinted from. cytotoxic. Thus, the rib mutants retain the “invasion and Hashemi had observed on electron micrographs that trafficking pore” but are defective in the “cytolysin/egress when present in macrophages at numbers greater than 25 per pore”, since they replicate within but fail to egress from the cell, L. pneumophila was usually dispersed within the cyto- host cells (Figs. 3 and 4). plasm of the host cell. The phenotype of the rib mutants is attributable to a point deletion in the icmT gene that is predicted to result in a 5. Apoptotic or necrotic cell death truncated protein of 54 amino acids instead of the 86 amino acids of the native protein [13,21]. In contrast, an icmT null 5.1. Induction of apoptosis by L. pneumophila in mutant is defective in both intracellular replication and pore mammalian but not protozoan host cells formation [13,21,74]. It has been shown that icmT expression is induced at stationary phase compared with the exponential Apoptosis requires a cascade of activation of a family of phase. We have shown that IcmT is bifunctional and that cysteine proteases (caspases) that specifically cleave proteins the carboxy terminus is essential for the pore-forming after aspartate residues. Among them, caspase-3 plays a “cytolysin/egress pore” activity, and the amino terminus is central role, allowing caspase-activated DNase to enter the essential to export effectors involved in various pathogenic nucleus and degrade chromosomal DNA. Muller et al. traits [13,21]. have shown that L. pneumophila induces apoptosis in We hypothesized that upon transition to the post- HL-60 human-derived macrophages after 24–48 h, at a mul- exponential phase of growth, the Rib toxin activity is trig- tiplicity of infection (MOI) of 10–100. The induction of gered, resulting in insertions of pores in the phagosomal apoptosis in mammalian cells is mediated by activation of membrane, leading to its disruption and bacterial egress into caspase-3 that is dose dependent and is maximal at 3 h the cytoplasm (Figs. 3 and 4). To test this hypothesis, we post-infection at an MOI of 50 [16,17] (Fig. 4). In alveolar examined late stages of the intracellular infection of mac- epithelial cells and macrophages, the induction of apoptosis rophages and amoebae by electron microscopy. Our data is dose dependent but not largely growth-phase regulated and showed that disruption of the phagosomal membrane was can be induced by extracellular bacteria [16,17]. The dot/icm detectable 12 h post-infection of both Acanthamoeba mutants of L. pneumophila are defective in inducing polyphaga and macrophages (unpublished data). After caspase-3 activation and, thus, apoptosis [16,18]. Therefore, 12 and 18 h post-infection in both host cells, vesicles and the Dot/Icm type IV secretion system of L. pneumophila is organelles from the host cytoplasm entered into the essential for the induction of apoptosis in mammalian cells Legionella-containing phagosome. Between 18 and 24 h, [16,18]. The pore-forming toxin is not required for the induc- most of the bacteria present in this disrupted phagosomal tion of apoptosis, but upon entry into the post-exponential membrane were surrounded by cytoplasmic organelles, and growth phase, it enhances the ability of the bacteria to induce not by distinct phagosomal membrane (unpublished data). apoptosis. These data show that the phagosomal membrane is disrupted Interestingly, L. pneumophila induces apoptosis in human first, rather than undergoing lysis simultaneously with the phagocytes but not in protozoan host cells such as A. castel- plasma membrane. Whether this disruption is the result of a lanii [19,79]. Moreover, although A. polyphaga is capable of mechanical process or linked to the pore-forming activity of undergoing apoptosis, including DNA fragmentation, upon the type IV secretion system [21,53,73], is not known. Our stimulation by actinomycin D, L. pneumophila does not data are consistent with a previous study in which Katz and induce apoptosis in A. polyphaga [17,19]. 136 M. Molmeret et al. / Microbes and Infection 6 (2004) 129–139 5.2. Induction of necrosis by L. pneumophila in disruption of organelles and the plasma membrane occurs, mammalian and protozoan cells culminating in lysis of the host cells and bacterial egress. The dot/icm-regulated Rib pore-forming toxin is essential 5.4. The neuronal apoptosis inhibitory protein (Naip) for L. pneumophila induction of necrosis, killing, and exiting and susceptibility of mice to L. pneumophila the host cells [19–21]. At high MOI, mutants defective in the Rib pore-forming toxin replicate like the wild-type strains L. pneumophila is unable to replicate in macrophages within the protozoan and mammalian cells but are trapped derived from most inbred mouse strains, with the exception within these cells and cannot be released (Figs. 3 and 4) of the A/J strain. The natural resistance of these mouse [19,20]. The expression of the pore-forming activity by L. strains to infection with L. pneumophila is controlled by the pneumophila grown in vitro and within macrophages is com- expression of a single dominant locus mapped on mouse pletely repressed during exponential growth but is tempo- chromosome 13, designated lgn1 [41,43,81]. The intracellu- rarily activated upon entry into post-exponential phase lar growth of species other than L. pneumophila are not under (Fig. 4). Therefore, the Rib pore-forming activity is not lgn’s control. required for phagosomal trafficking and intracellular multi- The mouse lgn1 region includes six copies of the neuronal plication of L. pneumophila within the cells, but is essential apoptosis inhibitory protein (naip) gene. The Naip proteins for induction of cytolysis of the infected macrophages have been shown to be direct inhibitors of caspases 3 and 7 [19,20]. Thus, the C-terminus of IcmT is essential for pore. Interestingly, the Naip proteins are less expressed in formation-mediated cytolysis [20,21]. macrophages derived from permissive A/J mice than from Experiments performed with an IcmT null mutant which the non-permissive inbred strains, but their expression is is defective in intracellular trafficking, and multiplication in increased after the phagocytic events in both strains of mice both protozoan and mammalian cells, suggest that IcmT is. This increase does not require intracellular replication, bifunctional [13,21]. The C-terminus of IcmT is essential for since the avirulent dotA mutant and the inert latex particles pore formation-mediated cytolysis in mammalian cells, and also cause an increase in naip expression. This reduced the N-terminus is required for intracellular trafficking. It naip expression in A/J macrophages may result in enhanced is unlikely that IcmT is an effector or a common regulator, ability of L. pneumophila to induce apoptosis through acti- but it is possible that this protein is a co-factor involved in the vation of caspase-3 and thus, increased permissiveness to export of different substrates with roles in pore-forming infection. Recently, naip5, also known as birc1e (bacu- toxicity and intracellular trafficking. Effectors of this loviral inhibitory apoptosis protein repeat-containing 1), was type IV secretion system remain to be identified. shown to be the only gene responsible for susceptibility to Legionella [85,86]. In contrast to other Naip expression lev- 5.3. Egress of L. pneumophila els, Naip5 expression levels have shown large differences between A/J macrophages and non-permissive macrophages. The ability to lyse host cells and to egress from them is a. A bacterial artificial chromosome (BAC) transgenic line fundamental step in the pathogenic cycle of intracellular expressing a non-permissive allele of naip5 has exhibited a bacteria and determines the overall consequences of the reduction in permissiveness to Legionella [85,86]. An anti- infection of an organism. Apoptosis and necrosis are the two sense inhibitor of the translation of the mRNA of Naip5 has commonly observed types of cell death. Necrosis is charac- caused an increase in the permissiveness of permissive and terized by physical damage causing cell death. Apoptosis is a non-permissive macrophages to Legionella. These re- regulated suicide program of the cell, manifesting morpho- sults indicate a role for birc1e/naip5 in macrophage resis- logical and biochemical features distinct from those of necro- tance to L. pneumophila infection. The mechanism of action sis. Killing of mammalian cells by L. pneumophila has is not yet known. been proposed to occur in two phases [16,17] (Fig. 4). In the Although Naip differs from the classical IAPs , it was first phase, L. pneumophila induces apoptosis in macro- thought to belong to the inhibitor of apoptosis (IAP) family phages, monocytes and alveolar epithelial cells during the of anti-apoptotic proteins, which were first identified in bacu- early stages of the infection [17,79], apoptosis which is loviruses. A recent study has shown that Naip proteins mediated through the activation of caspase-3. Induction are part of the nucleotide-binding oligomerization domain of apoptosis is largely independent of the bacterial growth (NOD) proteins. NOD proteins are a large family of proteins phase. The second phase is mediated through rapid involved in apoptosis and innate immunity [89–91]. Several induction of necrosis by L. pneumophila upon entry into the NOD proteins have already been shown to be implicated in post-exponential phase of growth, when the bacteria become the activation of caspases such as APAF1, which is involved cytotoxic (Fig. 4). Our working model of bacterial egress can in activation of caspase 9. NOD1 and NOD2, also part of be presented in three steps (Fig. 4). First, upon exiting the the NOD family, have been shown to mediate the recognition exponential phase of intracellular growth, an “egress pore” is of specific Gram-negative bacterial components such as li- inserted into the phagosomal membrane, leading to its dis- popolysaccharides (LPS) and peptidoglycan, once they are ruption. Second, the bacteria egress into cytoplasm. Third, processed in the cytosol [90,93,94]. Most of the NOD family M. Molmeret et al. / Microbes and Infection 6 (2004) 129–139 137 members contain three distinct functional domains. The first protein is not essential in phagosome transport or replicative domain is the amino-terminal effector-binding domain organelle biogenesis. Interestingly, L. pneumophila avoids (EBD), which is variable among NOD family members. phagosome–lysosome fusion within the first few minutes of The EBD amino-terminal domain of the Naip proteins con- the intracellular infection, and the phagosomes are converted tains an N-terminal domain with 1–3 repeats of an approxi- into endoplasmic reticulum-derived organelles that support mately 65-amino-acid motif, termed the baculovirus IAP intracellular replication. This process involves intercept- repeat (BIR) [88,90]. The role of BIRs in Naip remains to be ing early secretory vesicles exiting from the transitional ER determined, but it is thought to bind and inhibit caspase-3. After 4 h, L. pneumophila starts to replicate within this. The second domain is a central domain, designated replicative organelle. Between 8 and 18 h, the phagosomal NOD, which mediates self-oligomerization, required for the membrane is gradually disrupted, and the bacteria become activation of downstream effector molecules. The third do- cytoplasmic and are dispersed among cytoplasmic organelles main is a carboxy-terminal ligand-recognition domain such as mitochondria and lysosomes prior to lysis of the host (LRD), generally called LRR domain (leucine-rich repeats) cell (Molmeret et al., submitted). Further investigations [89,91]. Mammalian toll-like-receptors (TLRs) have mul- should be focused on determination of whether the disruption tiple LRRs in the ectodomain. of the phagosomal membrane is due to the action of a toxin. The LRD domain of Nod1 and Nod2 have been shown to Understanding this unique intracellular fate at the molecular be essential for LPS-mediated NF-jB activation, indepen- and the cellular level will unravel fascinating aspects of the dently of TLR4, MyD88 and TRAF6, which all together, intricate balance in the evolution of this host–parasite inter- mediate activation of NF-jB [93,94]. In fact, Nod1 and Nod2 action. seem to be the cytosolic counterparts of TLRs. Activa- tion of the TLRs initiates intracellular signaling cascades that culminate in the activation of NF-jB. NF-jB has been re- References ferred to as a central mediator of the immune response, since C.B. Fliermans, Ecology of Legionella: from data to knowledge with its activation controls the expression of many inflammatory a little wisdom, Microb. Ecol. 32 (1996) 203–228. cytokines, chemokines, immune receptors, and cell surface B.S. Fields, The molecular ecology of legionellae, Trends Microbiol. adhesion molecules. It is also a central regulator of stress 4 (1996) 286–290. responses. However, it is not known whether NAIP, M.A. Horwitz, S.C. Silverstein, Legionnaires’ disease bacterium which renders macrophages permissive to replication by L. (Legionella pneumophila) multiples intracellularly in human mono- cytes, J. Clin. Invest. 66 (1980) 441–450. pneumophila, or TLRs known to bind components of the M.A. Horwitz, The Legionnaires’ disease bacterium (Legionella bacterial surface (TLR4 in particular, for the LPS of the pneumophila) inhibits phagosome–lysosome fusion in human mono- Gram-negative bacteria) on the surface of the host cell are cytes, J. Exp. Med. 158 (1983) 2108–2126. involved in the regulation of innate immunity to L. pneumo- M.A. Horwitz, Formation of a novel phagosome by the Legionnaires’ phila via the activation of NF-jB. Naip has been shown to be disease bacterium (Legionella pneumophila) in human monocytes, J. Exp. Med. 158 (1983) 1319–1331. less expressed in A/J macrophages than in non-permissive M.A. Horwitz, Phagocytosis of the Legionnaires’ disease bacterium ones and is a direct inhibitor of caspase-3. If Naip (Legionella pneumophila) occurs by a novel mechanism: engulfment proteins mediate the activation of NF-jB, a lower concentra- within a pseudopod coil, Cell 36 (1984) 27–33. tion of Naip in the permissive macrophages may result in a M.A. Horwitz, F.R. Maxfield, Legionella pneumophila inhibits acidi- decreased ability to activate NF-jB and in enhanced ability fication of its phagosome in human monocytes, J. Cell Biol. 99 (1984) 1936–1943. of L. pneumophila to induce apoptosis. G. Segal, M. Purcell, H.A. Shuman, Host cell killing and bacterial Therefore, Naip5 can potentially mediate resistance to L. conjugation require overlapping sets of genes within a 22-kb region of pneumophila through two different pathways. First, it may the Legionella pneumophila chromosome, Proc. Natl. Acad. Sci. USA function as an IAP-like protein to block caspase-3 activation, 95 (1998) 1669–1674. which is required for intracellular replication (Molmeret et G. Segal, H.A. Shuman, Intracellular multiplication and human mac- rophage killing by Legionella pneumophila are inhibited by conjugal al., 2003 unpublished). Second, Naip5 may function as a components of IncQ plasmid RSF1010, Mol. Microbiol. 30 (1998) Nod-like protein that activates NF-jB to generate certain 197–208. responses involved in apoptosis and/or innate immunity. J.P. Vogel, H.L. Andrews, S.K. Wong, R.R. Isberg, Conjugative trans- fer by the virulence system of Legionella pneumophila, Science 279 (1998) 873–876. G. Segal, H.A. Shuman, Legionella pneumophila utilizes the same 6. Conclusion genes to multiply within Acanthamoeba castellanii and human mac- rophages, Infect. Immun. 67 (1999) 2117–2124. The type IV secretion system is a key virulence system of M.S. Swanson, R.R. Isberg, Association of Legionella pneumophila L. pneumophila, allowing it to invade the host cells, replicate, with the macrophage endoplasmic reticulum, Infect. Immun. 63 evade the endocytic pathway, induce apoptosis, and egress (1995) 3609–3620. M. Molmeret, O.A. Alli, M. Radulic, M. Susa, M. Doric, Y. Abu from the host cells [13–21]. Not all the functions of the Kwaik, The C-terminus of IcmT is essential for pore formation and for Dot/Icm proteins have been understood, and no effectors intracellular trafficking of Legionella pneumophila within Acan- other than RalF have yet been identified. However, the RalF thamoeba polyphaga, Mol. Microbiol. 43 (2002) 1139–1150. 138 M. Molmeret et al. / Microbes and Infection 6 (2004) 129–139 M. Watarai, I. Derre, J. Kirby, J.D. Growney, W.F. Dietrich, D.L. Weinbaum, R.R. Benner, J.N. Dowling, A. Alpern, A.W. Pas- R.R. Isberg, Legionella pneumophila is internalized by a macropi- culle, G.R. Donowitz, Interaction of Legionella micdadei with human nocytotic uptake pathway controlled by the Dot/Icm system and the monocytes, Infect. Immun. 46 (1984) 68–73. mouse Lgn1 locus, J. Exp. Med. 194 (2001) 1081–1096. J.N. Dowling, A.K. Saha, R.H. Glew, Virulence factors of the family H. Hilbi, G. Segal, H.A. Shuman, Icm/dot-dependent upregulation of Legionellaceae, Microbiol. Rev. 56 (1992) 32–60. phagocytosis by Legionella pneumophila, Mol. Microbiol. 42 (2001) T.W. Nash, D.M. Libby, M.A. Horwitz, Interaction between the 603–617. legionnaires’ disease bacterium (Legionella pneumophila) and human L.-Y. Gao, Y. Abu Kwaik, Activation of caspase-3 in Legionella alveolar macrophages. Influence of antibody, lymphokines, and pneumophila-induced apoptosis in macrophages, Infect. Immun. 67 hydrocortisone, J. Clin. Invest. 74 (1984) 771–782. (1999) 4886–4894. M.A. Horwitz, S.C. Silverstein, Interaction of the legionnaires’ disease L.-Y. Gao, Y. Abu Kwaik, Apoptosis in macrophages and alveolar bacterium (Legionella pneumophila) with human phagocytes II. Anti- epithelial cells during early stages of infection by Legionella pneumo- body promotes binding of L. pneumophila to monocytes but does not phila and its role in cytopathogenicity, Infect. Immun. 67 (1999) inhibit intracellular multiplication, J. Exp. Med. 153 (1981) 398–406. 862–870. S.L. Cirillo, J. Lum, J.D. Cirillo, Identification of novel loci involved S.D. Zink, L. Pedersen, N.P. Cianciotto, Y. Abu Kwaik, The Dot/Icm in entry by Legionella pneumophila, Microbiology 146 (2000) 1345– type IV secretion system of Legionella pneumophila is essential for 1359. the induction of apoptosis in human macrophages, Infect. Immun. 70 Y. Yamamoto, T.W. Klein, H. Friedman, Genetic control of macroph- (2002) 1657–1663. age susceptibility to infection by Legionella pneumophila, FEMS L.-Y. Gao, Y. Abu Kwaik, The mechanism of killing and exiting the Microbiol. Immunol. 89 (1992) 137–146. protozoan host Acanthamoeba polyphaga by Legionella pneumo- M.C. Beckers, S. Yoshida, K. Morgan, E. Skamene, P. Gros, Natural phila, Environ. Microbiol. 2 (2000) 79–90. resistance to infection with Legionella pneumophila: chromosomal O.A.T. Alli, L.-Y. Gao, L.L. Pedersen, S. Zink, M. Radulic, M. Doric, localization of the Lgn1 susceptibility gene, Mamm. Genome 6 (1995) Y. Abu Kwaik, Temporal pore formation-mediated egress from mac- 540–545. rophages and alveolar epithelial cells by Legionella pneumophila, M.C. Beckers, E. Ernst, E. Diez, C. Morissette, F. Gervais, K. Hunter, Infect. Immun. 68 (2000) 6431–6440. D. Housman, S. Yoshida, E. Skamene, P. Gros, High-resolution link- M. Molmeret, O.A. Alli, S. Zink, A. Flieger, N.P. Cianciotto, Y. Abu age map of mouse chromosome 13 in the vicinity of the host resis- Kwaik, icmT is essential for pore formation-mediated egress of tance locus Lgn1, Genomics 39 (1997) 254–263. Legionella pneumophila from mammalian and protozoan cells, W.F. Dietrich, D.M. Damron, R.R. Isberg, E.S. Lander, M.S. Swan- Infect. Immun. 70 (2002) 69–78. son, Lgn1, a gene that determines susceptibility to Legionella pneu- T.J. Rowbotham, Preliminary report on the pathogenicity of mophila, maps to mouse chromosome 13, Genomics 26 (1995) 443– Legionella pneumophila for freshwater and soil amoebae, J. Clin. 450. Pathol. 33 (1980) 1179–1183. L.G. Tilney, O.S. Harb, P.S. Connelly, C.G. Robinson, C.R. Roy, How T.J. Rowbotham, Current views on the relationships between amoe- the parasitic bacterium Legionella pneumophila modifies its phago- bae, legionellae and man, Isr. J. Med. Sci. 22 (1986) 678–689. some and transforms it into rough ER: implications for conversion of B. Byrne, M.S. Swanson, Expression of Legionella pneumophila plasma membrane to the ER membrane, J. Cell Sci. 114 (2001) virulence traits in response to growth conditions, Infect. Immun. 66 4637–4650. (1998) 3029–3034. M.S. Swanson, R.R. Isberg, Formation of the Legionella pneumo- B.K. Hammer, M.S. Swanson, Co-ordination of Legionella pneumo- phila replicative phagosome, Infect. Agents Dis. 2 (1995) 269–271. phila virulence with entry into stationary phase by ppGpp, Mol. J.A. Bozue, W. Johnson, Interaction of Legionella pneumophila with Microbiol. 33 (1999) 721–731. Acanthamoeba catellanii: uptake by coiling phagocytosis and inhibi- M.A. Bachman, M.S. Swanson, RpoS co-operates with other factors tion of phagosome–lysosome fusion, Infect. Immun. 64 (1996) 668– to induce Legionella pneumophila virulence in the stationary phase, 673. Mol. Microbiol. 40 (2001) 1201–1214. S. Sturgill-Koszycki, M.S. Swanson, Legionella pneumophila repli- L.M. Hales, H.A. Shuman, The Legionella pneumophila rpoS gene is cation vacuoles mature into acidic, endocytic organelles, J. Exp. Med. required for growth within Acanthamoeba castellanii, J. Bacteriol. 192 (2000) 1261–1272. 181 (1999) 4879–4889. Y. Abu Kwaik, Induced expression of the Legionella pneumophila M.M. Samrakandi, S.L. Cirillo, D.A. Ridenour, L.E. Bermudez, gene encoding a 20-kilodalton protein during intracellular infection, J.D. Cirillo, Genetic and phenotypic differences between Legionella Infect. Immun. 66 (1998) 203–212. pneumophila strains, J. Clin. Microbiol. 40 (2002) 1352–1362. L.-Y. Gao, M. Susa, B. Ticac, Y. Abu Kwaik, Heterogeneity in intrac- T. Zusman, O. Gal-Mor, G. Segal, Characterization of a Legionella ellular replication and cytopathogenicity of Legionella pneumophila pneumophila relA insertion mutant and toles of RelA and RpoS in and Legionella micdadei in mammalian and protozoan cells, Microb. virulence gene expression, J. Bacteriol. 184 (2002) 67–75. Pathog. 27 (1999) 273–287. Y. Abu Kwaik, Fatal attraction of mammalian cells to Legionella L.-Y. Gao, B.J. Stone, J.K. Brieland, Y. Abu Kwaik, Different fates of pneumophila, Mol. Microbiol. 30 (1998) 689–696. Legionella pneumophila pmi and mil mutants within human-derived N.R. Payne, M.A. Horwitz, Phagocytosis of Legionella pneumophila macrophages and alveolar epithelial cells, Microb. Pathog. 25 (1998) is mediated by human monocyte complement receptors, J. Exp. Med. 291–306. 166 (1987) 1377–1389. P.J. Christie, J.P. Vogel, Bacterial type IV secretion: conjugation J.A. Elliott, W.C. Winn Jr, Treatment of alveolar macrophages with systems adapted to deliver effector molecules to host cells, Trends cytochalasin D inhibits uptake and subsequent growth of Legionella Microbiol. 8 (2000) 354–360. pneumophila, Infect. Immun. 51 (1986) 31–36. J.E. Kirby, R.R. Isberg, Legionnaires’ disease: the pore macrophage F.G. Rodgers, F.C. Gibson III, Opsonin-independent adherence and and the legion of terror within, Trends Microbiol. 6 (1998) 256–258. intracellular development of Legionella pneumophila within U-937 J.E. Kirby, J.P. Vogel, H.L. Andrews, R.R. Isberg, Evidence for pore- cells, Can. J. Microbiol. 39 (1993) 718–722. forming ability by Legionella pneumophila, Mol. Microbiol. 27 C. Rechnitzer, J. Blom, Engulfment of the Philadelphia strain of (1998) 323–336. Legionella pneumophila within pseudopod coils in human phago- J. Coers, C. Monahan, C.R. Roy, Modulation of phagosome biogen- cytes. Comparison with the other Legionella strains and species, Acta esis by Legionella pneumophila creates an organelle permissive for Pathol. Microbiol. Immunol. Scand. (B) 97 (1989) 105–114. intracellular growth, Nature Cell Biol. 1 (1999) 451–453. M. Molmeret et al. / Microbes and Infection 6 (2004) 129–139 139 K.H. Berger, J. Merriam, R.R. Isberg, Altered intracellular targeting O. Gal-Mor, T. Zusman, G. Segal, Analysis of DNA regulatory ele- properties associated with mutations in the Legionella pneumophila ments required for expression of the Legionella pneumophila icm and dotA gene, Mol. Microbiol. 14 (1994) 809–822. dot virulence genes, J. Bacteriol. 184 (2002) 3823–3833. C.R. Roy, R.R. Isberg, Topology of Legionella pneumophila DotA: an P. Anderson, Kinase cascades regulating entry into apoptosis, Micro- inner membrane protein required for replication in macrophages, biol. Mol. Biol. Rev. 61 (1997) 33–46. Infect. Immun. 65 (1997) 571–578. M. Enari, H. Sakahira, H. Yokoyama, K. Okawa, A. Iwamatsu, K.H. Berger, R.R. Isberg, Two distinct defects in intracellular growth S. Nagata, A caspase-activated DNase that degrades DNA during complemented by a single genetic locus in Legionella pneumophila, apoptosis, and its inhibitor ICAD, Nature 391 (1998) 43–50. Mol. Microbiol. 7 (1993) 7–19. A. Muller, J. Hacker, B. Brand, Evidence for apoptosis of human macrophage-like HL-60 cells by Legionella pneumophila infection, J. Coers, J.C. Kagan, M. Matthews, H. Nagai, D.M. Zuckman, Infect. Immun. 64 (1996) 4900–4906. C.R. Roy, Identification of icm protein complexes that play distinct S. Hagele, J. Hacker, B.C. Brand, Legionella pneumophila kills roles in the biogenesis of an organelle permissive for Legionella pneu- human phagocytes but not protozoan host cells by inducing apoptotic mophila intracellular growth, Mol. Microbiol. 38 (2000) 719–736. cell death, FEMS Microbiol. Lett. 169 (1998) 51–58. G. Segal, J.J. Russo, H.A. Shuman, Relationships between a new type J.J. Cohen, Overview: mechanisms of apoptosis, Immunol. Today 14 IV secretion system and the icm/dot virulence system of Legionella (1993) 126–130. pneumophila, Mol. Microbiol. 34 (1999) 799–809. Y. Yamamoto, T.W. Klein, H. Friedman, Legionella pneumophila T. Komano, T.Yoshida, K. Narahara, N. Furuya, The transfer region of growth in macrophages from susceptible mice is genetically con- IncI1 plasmid R64: similarities between R64 tra and Legionella trolled, Proc. Soc. Exp. Biol. Med. 196 (1991) 405–409. icm/dot genes, Mol. Microbiol. 35 (2000) 1348–1359. H. Miyamoto, K. Maruta, M. Ogawa, M.C. Beckers, P. Gros, H. Nagai, C.R. Roy, The DotA protein from Legionella pneumophila S. Yoshida, Spectrum of Legionella species whose intracellular mul- is secreted by a novel process that requires the Dot/Icm transporter, tiplication in murine macrophages is genetically controlled by Lgn1, EMBO J. 20 (2001) 5962–5970. Infect. Immun. 64 (1996) 1842–1845. M. Watarai, H.L. Andrews, R.R. Isberg, Formation of a fibrous struc- J.K. Maier, Z. Lahoua, N.H. Gendron, R. Fetni, A. Johnston, ture on the surface of Legionella pneumophila associated with expo- J. Davoodi, D. Rasper, S. Roy, R.S. Slack, D.W. Nicholson, sure of DotH and DotO proteins after intracellular growth, Mol. A.E. MacKenzie, The neuronal apoptosis inhibitory protein is a direct Microbiol. 39 (2001) 313–330. inhibitor of caspases 3 and 7, J. Neurosci. 22 (2002) 2035–2043. M. Matthews, C.R. Roy, Identification and subcellular localization of E. Diez, Z. Yaraghi, A. MacKenzie, P. Gros, The neuronal apoptosis the Legionella pneumophila IcmX protein: a factor essential for inhibitory protein (Naip) is expressed in macrophages and is modu- lated after phagocytosis and during intracellular infection with establishment of a replicative organelle in eukaryotic host cells (in Legionella pneumophila, J. Immunol. 164 (2000) 1470–1477. process citation), Infect. Immun. 68 (2000) 3971–3982. E.K. Wright, S.A. Goodart, J.D. Growney, V. Hadinoto, M.G. Endrizzi, C.R. Roy, L.G. Tilney, The road less traveled: transport of Legionella E.M. Long, K. Sadigh, A.L. Abney, I. Bernstein-Hanley, W.F. Dietrich, to the endoplasmic reticulum, J. Cell Biol. 158 (2002) 415–419. Naip5 affects host susceptibility to the intracellular pathogen G. Dumenil, R.R. Isberg, The Legionella pneumophila IcmR protein Legionella pneumophila, Curr. Biol. 13 (2003) 27–36. exhibits chaperone activity for IcmQ by preventing its participation in E. Diez, S.H. Lee, S. Gauthier, Z. Yaraghi, M. Tremblay, S. Vidal, high-molecular-weight complexes, Mol. Microbiol. 40 (2001) 1113– P. Gros, Birc1e is the gene within the Lgn1 locus associated with 1127. resistance to Legionella pneumophila, Nat. Genet. 33 (2003) 55–60. S.M. Katz, S. Hashemi, Electron microscopic examination of the N. Roy, Q.L. Deveraux, R. Takahashi, G.S. Salvesen, J.C. Reed, The inflammatory response to Legionella pneumophila in guinea pigs, c-IAP-1 and c-IAP-2 proteins are direct inhibitors of specific Lab. Invest. 46 (1982) 24–32. caspases, EMBO J. 16 (1997) 6914–6925. Y. Abu Kwaik, The phagosome containing Legionella pneumophila P. Liston, N. Roy, K. Tamai, C. Lefebvre, S. Baird, G. Cherton-Horvat, within the protozoan Hartmanella vermiformis is surrounded by the R. Farahani, M. McLean, J.E. Ikeda, A. MacKenzie, R.G. Korneluk, rough endoplasmic reticulum, Appl. Environ. Microbiol. 62 (1996) Suppression of apoptosis in mammalian cells by NAIP and a related 2022–2028. family of IAP genes, Nature 379 (1996) 349–353. B.R. Dorn, W.A. Dunn Jr, A. Progulske-Fox, Bacterial interactions N. Inohara, G. Nunez, The NOD: a signaling module that regulates with the autophagic pathway, Cell Microbiol. 4 (2002) 1–10. apoptosis and host defense against pathogens, Oncogene 20 (2001) E. Gagnon, S. Duclos, C. Rondeau, E. Chevet, P.H. Cameron, 6473–6481. O. Steele-Mortimer, J. Paiement, J.J. Bergeron, M. Desjardins, Endo- N. Inohara, G. Nunez, NODs: intracellular proteins involved in plasmic reticulum-mediated phagocytosis is a mechanism of entry inflammation and apoptosis, Nat. Rev. Immunol. 3 (2003) 371–382. into macrophages, Cell 110 (2002) 119–131. N. Inohara, Y. Ogura, G. Nunez, Nods: a family of cytosolic proteins that regulate the host response to pathogens, Curr. Opin. Microbiol. 5 H. Nagai, J.C. Kagan, X. Zhu, R.A. Kahn, C.R. Roy, A bacterial (2002) 76–80. guanine nucleotide exchange factor activates ARF on Legionella Y. Hu, L. Ding, D.M. Spencer, G. Nunez, WD-40 repeat region phagosomes, Science 295 (2002) 679–682. regulates Apaf-1 self-association and procaspase-9 activation, J. Biol. J.C. Kagan, C.R. Roy, Legionella phagosomes intercept vesicular Chem. 273 (1998) 33489–33494. traffic from endoplasmic reticulum exit sites, Nat. Cell Biol. 4 (2002) S.E. Girardin, I.G. Boneca, L.A. Carneiro, A. Antignac, M. Jehanno, 945–954. J. Viala, K. Tedin, M.K. Taha, A. Labigne, U. Zathringer, A.J. Coyle, D.M. Zuckman, J.B. Hung, C.R. Roy, Pore-forming activity is not P.S. DiStefano, J. Bertin, P.J. Sansonetti, D.J. Philpott, Nod1 detects a sufficient for Legionella pneumophila phagosome trafficking and unique muropeptide from Gram-negative bacterial peptidoglycan, intracellular replication, Mol. Microbiol. 32 (1999) 990–1001. Science 300 (2003) 1584–1587. M. Molmeret, Y. Abu Kwaik, How does Legionella pneumophila exit N. Inohara, Y. Ogura, F.F. Chen, A. Muto, G. Nunez, Human Nod1 the host cell? Trends Microbiol. 10 (2002) 258–260. confers responsiveness to bacterial lipopolysaccharides, J. Biol. G. Segal, H.A. Shuman, Characterization of a new region required for Chem. 276 (2001) 2551–2554. macrophage killing by Legionella pneumophila, Infect. Immun. 65 X. Li, G. R. Stark, NFkappaB-dependent signaling pathways, Exp. (1997) 5057–5066. Hematol. 30 (2002) 285–296.