Biotechnology Careers & DNA Structure (PDF)
Document Details
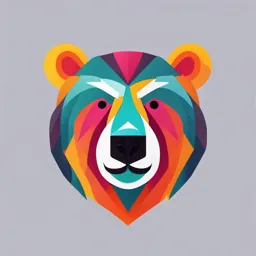
Uploaded by DurableEllipse
2021
Tags
Summary
This document introduces biotechnology careers and explains DNA structure and function. It details how DNA encodes proteins and the central dogma of biology. The document also discusses eukaryotic and prokaryotic chromosomal structure, bacterial culture methods, viruses, genetic engineering, and gel electrophoresis.
Full Transcript
Biotech Careers Photo courtesy of Paul Kaufman. Molecular Biologist/ Associate Professor Paul D. Kaufman, PhD...
Biotech Careers Photo courtesy of Paul Kaufman. Molecular Biologist/ Associate Professor Paul D. Kaufman, PhD University of Massachusetts Medical School Worcester, MA Dr. Kaufman heads a group that studies how eukaryotic cells assemble chromosomes and how the chromosomes function. They are especially interested in chromatin (DNA and pro- tein complexes in the nucleus). The group studies several dif- ferent classes of proteins used by yeast and human cells to deposit histone proteins onto DNA, as well as enzymes that chemically modify histone structure and function. Paul’s primary responsibility is scientific discovery. He conducts experiments of his own, trains graduate students, and oversees the research of postdoctoral fellows in his lab- oratory. His typical day involves reviewing recent experi- ments by members of his lab and suggesting improvements, writing papers and grants, reading scientific literature, and attending research seminars. For several weeks each year, Paul travels to scientific meetings to present research re- sults from his laboratory. chromatin (chro ma tin) nuclear DNA and proteins 112 0112-0147_Biotech2e_Ch04.indd 112 9/13/21 4:05 PM 4 Studying DNA Introduction to Learning Outcomes Describe the structure and function of DNA and explain the process by which it encodes for proteins Explain how molecular modeling is used to study the structure and function of DNA as well as other biomolecules. Differentiate between eukaryotic and prokaryotic chromosomal structure and explain how this difference impacts gene regulation in the two cell types Differentiate between bacterial cultures grown in liquid and solid media and explain how to prepare each media type using sterile technique Discuss the characteristics of viruses and their importance in genetic engineering Explain the fundamental process of genetic engineering and give examples of the following applications: recombinant DNA technology, site-specific mutagenesis, and gene therapy Describe the process of gel electrophoresis and discuss how the characteristics of molecules affect their migration through a gel 4.1 DNA Structure and Function In the late decades of the twentieth century, a “new” biotechnology industry grew out of innovative techniques to both transfer DNA between cells and to manipulate the cells to manufacture specific proteins. These techniques revolutionized science and industry, creating new kinds of companies and new kinds of science jobs. In this chapter, some of the methods by which DNA is isolated and studied are presented. Other biotechnologies are presented in later chapters, including genetic engineering, protein studies, and biomanufacturing. Together these have led many biotechnology companies to specialize in creating cellular-protein factories and modified organisms with new and more desirable characteristics. The manipulation of genetic information, specifically the deoxyribonucleic acid (DNA) and ribonucleic acid (RNA) codes, is at the center of most biotechnology research and development. The genetic 113 0112-0147_Biotech2e_Ch04.indd 113 9/13/21 4:05 PM information within cells is stored in DNA molecules (see Figure 4.1). Sections of the DNA sequence are then transcribed into RNA messages. The arrangement of nitrogen bases on a DNA strand determines the RNA sequence, and the RNA code determines the amino acids that are placed in the polypeptide chain of a protein (see Figure 4.2). Proteins do the work of cells, and they give cells and organisms their unique characteristics. The “Central Dogma of Biology” explains, in general, how genetic information is converted into structures and functions. It describes how small sections of DNA (genes) are transcribed into messenger RNA (mRNA) molecules, which Figure 4.1. Pure salmon DNA isolated from sperm cells. In are, in turn, translated at ribosomes into the proteins of the solution, DNA is clear, with bubbles trapped in long strands cell. Many biotechnology efforts modify DNA molecules with of DNA. During spooling, the solvent is squeezed out and the goal of affecting protein production. the DNA appears white. Photo by author. At a biotechnology company, the proteins produced in a cell may be the actual product desired, such as an insulin molecule manufactured in a pancreas cell. Or, the protein product may be a regulatory molecule, such as an enzyme needed to make another gene (gene) a section of DNA molecule, for example, cholesterol. on a chromosome that contains the Since so many proteins are necessary for the functions in an organism, the number genetic code of a protein of genes needed is enormous—about 20,000 to 25,000 genes in humans. A typical nitrogenous base (ni trog e nous base) a n cell synthesizes more than 2000 different kinds of protein. Hundreds or thousands important component of nucleic of copies of a particular protein may be needed at a given moment (remember that a acids (DNA and RNA), composed cell’s proteins may be structural or regulatory). Multiply that number of proteins by of one or two nitrogen-containing rings; forms the critical hydrogen the many hundreds of different cell types a multicellular organism contains, and the bonds between opposing strands of number of proteins produced by a single organism may reach over a million. Each a double helix protein has one or more genes coding for its production or regulation. The result is many thousands of genes coded for by several billion DNA bases in each species. The entire sum of DNA in a cell (the genome) is variable from organism to organism, but every cell within an organism, except sex cells, has the same genome. The sizes of the genomes from some important organisms are listed in Table 4.1. amino acids tRNA gene ribosome polypeptide mRNA protein = one or more folded polypeptide chains DNA Figure 4.2. The Central Dogma of Biology. Proteins are produced when genes on a DNA molecule are transcribed into mRNA, and mRNA is translated into the protein code. This is called “gene expression.” At any given moment, only a relatively small amount of DNA in a cell is being expressed. Chapter 4 114 0112-0147_Biotech2e_Ch04.indd 114 9/13/21 4:05 PM Table 4.1. Sizes of the Genomes from Some Model Organisms The abbreviation bp stands for base pair and refers to counting both nitrogenous bases on each side of the double helix at base pair (base pair) the a particular location on the strands. This is the accepted method of sizing DNA. two nitrogenous bases that are Size of the Genome connected by a hydrogen bond; for example, an adenosine bonded to Organism Number of Genes (bp) a thymine or a guanine bonded to Haemophilus influenzae: childhood ear infection 1,749 1,830,137 a cytosine Mycoplasma genitalium: free-living bacterium 470 580,070 phosphodiester bond (phos pho di es ter bond) Caenorhabditis elegans: free-living roundworm 19,899 97,000,000 a bond that is responsible for the Homo sapiens: humans 20,000–25,000 3,000,000,000 polymerization of nucleic acids by linking sugars and phosphates of adjacent nucleotides Although there are different quantities of DNA in cells from different organisms, hydrogen bond (hy dro gen all DNA from all organisms share many characteristics. In fact, DNA molecules from bond) a type of weak bond that different organisms are virtually identical, except in the order of nitrogenous bases involves the “sandwiching” of a hydrogen atom between two (containing nitrogen). The structure of DNA was first introduced and discussed in fluorine, nitrogen, or oxygen Chapter 2. A discussion of the similarities and variations in DNA molecules among atoms; especially important in organisms is in the following pages. the structure of nucleic acids and proteins pyrimidine (py rim i dine) Similarities in DNA Molecules Among Organisms a nitrogenous base composed of a single carbon ring; a component 1. All DNA molecules are composed of four nucleotide monomers that contain four of DNA nucleotides nitrogenous bases (see Figure 4.3a–d and Figure 2.34). purine (pu rine) a nitrogenous adenosine deoxynucleotide (A) guanosine deoxynucleotide (G) base composed of a double carbon ring; a component of DNA cytosine deoxynucleotide (C) thymine deoxynucleotide (T) nucleotides 2. Virtually all DNA molecules form a double helix (two sides) of repeating nucleotides, several million bp in length. Nucleotides connect to other 5'→3' 3'→5' strand strand nucleotides in the strand through strong phosphodiester bonds P between sugars and phosphates of adjacent nucleotides. Hydrogen bonds A T S 5-C (H-bonds) between nitrogen bases on each complementary strand hold the S sugar two sides of the DNA molecule together. Base pairing in DNA only occurs P P between adenine and thymine molecules (A-T), or between guanine and G C S phosphate cytosine molecules (G-C). Figure 4.4 a-d uses different molecular models to S group represent the structural features of a section of DNA. P P T A H H O S S N hydrogen H3C C H bonds P C C N P N A T C N S H C C C S C C H N O N N P H P H thymine C G H adenine S S P H H O P N G C S N C H S H C C N C N H C P C C nitrogen base N H C C N N Figure 4.4a. DNA Structure. This H N O H structural diagram of a section of a DNA guanine H H cytosine molecule shows how the nucleotides in one chain of the helix face one direction, Figure 4.3a-d. Nitrogenous Bases in DNA. while those in the other strand face the The four nitrogenous bases (adenine, thymine, other direction. Each nucleotide contains Figure 4.4b. Space-filling Model cytosine, and guanine) are found in the four a sugar molecule, a phosphate group, and of a Section of DNA In this space DNA nucleotides. Notice how two of them a nitrogenous base. Nitrogenous bases from filling model, the phosphate groups are composed of a single carbon ring (called each strand bond to each other in the center in the backbone strands of each side a pyrimidine), and two of them are composed through H-bonds. The H-bonds are rather of the double helix are visible (orange of a double carbon ring (called a purine). weak; therefore, the two strands of DNA phosphorus with red oxygen atoms). The entire nucleotide, including the sugar separate easily at high temperatures. Notice the (blue) nitrogenous bases in and phosphate group, is shown in Chapter 2, the center of the double helix making Figure 2.34. up the rungs in the DNA ladder. Introduction to Studying DNA 115 0112-0147_Biotech2e_Ch04.indd 115 9/13/21 4:05 PM 3. Because an adenosine deoxynucleotide (A) of one strand always bonds to a thymine deoxynucleotide (T) in the other strand, the amount of adenine in every double-stranded DNA molecule always equals the amount of thymine. Likewise, the amount of guanosine deoxynucleotide (G) in one strand of every double-stranded DNA molecule always equals the amount of cytosine (C) (See Figure 4.4d). Since adenine and guanine are purines, and thymine and cytosine are pyrimidines, the width of an A-T base pair is the same as the width of a G-C base pair. 4. The nucleotides in each of the two strands of DNA are oriented in the opposite direction of the other strand. On one side, the nucleotides are oriented upward, and on the other side, they are oriented downward. We say that the strands are antiparallel. This arrangement gives “directionality” to a DNA strand, so that the DNA strand is “read” in one direction only. All DNA molecules are antiparallel. Figure 4.4c. Ball and Stick (See Figures 4.4a–d) Figure 4.4d. Wire Model of Model of a Section of DNA In 5. The nitrogenous bases (A, C, G, and T) are stacked a Section of DNA The same this model, the same color coding 0.34 nanometer (nm) apart with 10 nitrogen bases DNA strand as in Figure 4.4c is seen as in Figure 4.4b. Tracing per complete turn of the helix. This stacking of represented in a wire model. the backbone strands down, the The nucleotides are color-coded pentagonal deoxyribose sugar bases ensures that the shape of the DNA molecule is (yellow=G, blue=C, green=A, molecules can be seen between constant throughout its length. The uniform shape red=T). Do you see the G-C, A-T phosphate groups. Notice on one ensures that enzymes and regulatory molecules can base pairing? The anti-parallel strand the sugars are facing up recognize the DNA molecule, and allows for coiling configuration of the strands is and one the other strand they are and packaging of the DNA (see Figure 4.5). seen in this model. facing down (the strands are anti- parallel. 6. DNA undergoes semiconservative 3' 5' replication, making two daughter strands from a single parent strand. During semiconservative antiparallel (an ti par al lel) replication, a strand unzips. Each original strand thymine a reference to the observation that acts as a template, or model, for building a new strands on DNA double helix have adenine their nucleotides oriented in the side. By the time replication is completed, two guanine opposite direction to one another identical DNA strands have been produced. One of cytosine semiconservative replication each copy goes into a newly forming daughter cell (sem i con ser va tive during cell division (see Figure 4.6). rep lic a tion) a form of replication in which each original strand of DNA acts as a template, or model, for building a new side; Variations in DNA Molecules 3' in this model one of each new copy Although DNA molecules are all remarkably similar 5' goes into a newly forming daughter cell during cell division from one organism to another, there are some variations, which may include the following: the number of DNA strands in the cells of an organism (ie, the number of chromosomes) the length in base pairs of the DNA strands 5' the number and type of genes (nucleotide sequences that code for protein production) and noncoding regions the shape of the DNA strands (circular or 3' 5' 3' 5' Figure 4.5. Circular DNA linear chromosomes) visualized by TEM (transmission Some of the unique characteristics of Figure 4.6. DNA Replication. DNA electron microscope) using replicates in a semiconservative fashion in cytochrome C and metal DNA from different sources are discussed in which one strand unzips and each side is shadowing techniques. a single, later sections. copied. It is considered semiconservative since circular DNA molecule is found one copy of each parent strand is conserved in in bacteria cells. ~40,000X the next generation of DNA molecules. © Corbis. Chapter 4 116 0112-0147_Biotech2e_Ch04.indd 116 9/13/21 4:06 PM Section 4.1 Review Questions 1. Describe the relationship between genes, mRNA, and proteins. 2. Name the four nitrogen-containing bases found in DNA molecules and identify how they create a base pair. 3. The strands on a DNA molecule are said to be “antiparallel.” What does antiparallel mean? 4. During cell division, DNA molecules are replicated in a semiconservative manner. What happens to the original DNA molecule during semiconservative replication? 4.2 Sources of DNA In nature, DNA is made in cells. For sources of DNA, scientists can find cells in nature or they can grow cultures of cells in the laboratory. Scientists have learned how to grow many different cells on or in a medium (source of nutrients) prepared in the medium (me di um) laboratory. Cells from cell cultures can be collected and broken open, a process called a suspension or gel that provides the nutrients (salts, sugars, growth lysis. Lysed cells release their DNA molecules in a mixture of other cellular molecules. factors, etc) and the environment Through separation techniques, the DNA molecules can be isolated from the other needed for cells to survive; plural cell molecules. is media The basic DNA molecule and the genetic code are the same from organism to lysis (ly sis) the breakdown or rupture of cells organism, but the packaging of the DNA and its location within the cell vary among R plasmid (R plas mid) a type groups of organisms. Knowing about the differences in DNA packaging in a cell will of plasmid that contains a gene for help a technician isolate DNA from different cells. antibiotic resistance Prokaryotic DNA Bacteria cells, such as E. coli, are prokaryotic, meaning they do not contain a nucleus or other membrane-bound organelles (see Figure 4.7). In a bacterium, the DNA is floating in the cytoplasm but is usually attached at one spot to the cell membrane. A bacterium typically contains only one long, circular DNA molecule (see Figure 4.5). It is usually supercoiled, folding over on itself like a twisted rubber band. Microscopically it appears as a dense area called the nucleoid. The DNA molecule is rather small and contains only several thousand genes. The well known bacterium, E. coli, has a single DNA strand containing approximately 4,400 genes with about 4.6 million bp. The entire E. coli genome codes for RNA and proteins. There is very little spacer (unused) DNA. The genes on the single DNA strand are, for the most part, necessary for survival. Some bacteria contain extra small rings of DNA floating in the cytoplasm (see Figure 4.8). These are called plasmids. A plasmid contains only a few genes (5 to 10), and these genes usually code for proteins that offer some additional characteristic that may be needed only under certain conditions but not others. Some of the most familiar plasmids are the R plasmids. These contain antibiotic resistance genes. Bacteria with these resistance genes can survive exposure to antibiotics that would normally kill or stunt them. Bacteria can transfer plasmids and, thus, genetic Figure 4.7. Prokaryotic cells, such as E. coli, do not contain information between themselves. There are several membrane-bound organelles such as mitochondria and ramifications of this practice. Genes, such as those for lysosomes. ~30,000X © Lester V. Bergman/Corbis/Getty Images. Introduction to Studying DNA 117 0112-0147_Biotech2e_Ch04.indd 117 9/13/21 4:06 PM flagella cytoplasm plasmid cell wall single circular DNA molecule in an an area called the nucleoid Figure 4.9. Beige (nontransformed) and blue-black Figure 4.8. Structure of a Bacterium. Although prokaryotic cells are (transformed) colonies of bacteria. The cells in the dark rather simple in structure, some may contain one or more plasmids. Plasmids colonies have taken in plasmids carrying a new gene that are tiny rings of “extra” DNA. So far, scientists have only found plasmids in codes for the production of a blue product. prokaryotes and yeast. © Ted Horowitz/Corbis/Getty Images. antibiotic resistance, can be transferred between bacteria and lead to deadly antibiotic- transformed (trans formed) resistant forms of disease-causing bacteria. Transferring plasmids may give bacteria the cells that have taken up foreign DNA and started expressing the a way of “evolving” by gaining new and better characteristics for survival. Scientists genes on the newly acquired DNA have learned how to use plasmids to transfer “genes of interest” into cells. When vector (vec tor) a piece of DNA these cells take up foreign DNA and start expressing the genes, they are considered that carries one or more genes into transformed (see Figure 4.9). a cell; usually circular as in plasmid vectors Different bacteria have different plasmids. Some bacteria have more than one kind operon (op er on) a section of of plasmid, and some bacteria contain no plasmids. Because plasmids are small and prokaryotic DNA consisting of one easy to extract from cells, they are often used as recombinant DNA (rDNA) vectors or more genes and their controlling to carry new genes into cells for transformation. Foreign DNA fragments (genes) elements can be cut and pasted into a plasmid vector. The recombinant plasmid may then be introduced into a cell. The cell will read the DNA code on the recombinant plasmid and start synthesizing the proteins coded for on the “foreign” gene(s). This is how Genentech, Inc. first manipulated E. coli cells to make human insulin. The scientists tricked the E. coli cells into reading the human genes that had been inserted into them on recombinant vector plasmids. Another difference between prokaryotic DNA and DNA from eukaryotic cells is that the gene expression (how genes are turned ON or OFF) in prokaryotes is rather simple with only a few controls. Figure 4.10 is a simplified model of an operon (two or more genes and their controlling elements) on a piece of prokaryotic geomic DNA. Remember that on a real bacterial chromosome there would be several hundred operons, one directly after another. In the middle of the operon is one or more structural genes. A structural gene is a section that actually codes for one or more mRNA molecules, which will later promoter region terminator 5' 3' A T A T A A T T A T T T T A T A T T structural genes A A T A A A 3' 5' operator RNA region polymerase Figure 4.10. Bacterial Operon. An operon contains the controlling elements that turn genetic expression ON and OFF. Chapter 4 118 0112-0147_Biotech2e_Ch04.indd 118 9/13/21 4:06 PM be translated into one or more proteins. For the structural gene(s) to be read or “expressed” as a functional protein, other accessory areas are required. For gene expression to occur in prokaryotes, the enzyme that synthesizes a mRNA molecule, RNA polymerase, must attach to a segment of DNA at a promoter RNA polymerase (RNA region of the operon. This essentially “turns on” the prokaryotic gene. The RNA po ly mer ase) a n enzyme that catalyzes the synthesis of polymerase works its way down the DNA strand to a structural gene, where it builds a complementary RNA strands from mRNA molecule from free-floating nucleotides, using the DNA strand as a template. a given DNA strand A synthesized mRNA is decoded into a peptide at a ribosome. A region called the promoter (pro mo ter) the operator, located just prior to the structural gene(s), can “turn off” the operon. If a region at the beginning of a gene where RNA polymerase binds; regulatory molecule attaches at the operator, the operon is turned off because the RNA the promoter “promotes” the polymerase is blocked from continuing down the strand to the gene(s). In this case, recruitment of RNA polymerase no protein is produced. Blocking or unblocking the operator is the way bacterial cells and other factors required for transcription make only certain proteins at certain times. It would be a waste, for example, for a cell operator (op er a tor) a region to make beta-galactosidase, which breaks lactose down to monosaccharides, if no on an operon that can either turn lactose was around (see Figure 4.11). on or off expression of a set of Genetic engineers utilize the promoter and operator regions to turn on and off the genes depending on the binding of a regulatory molecule production of certain genes. When the insulin gene from humans was genetically beta-galactosidase engineered into E. coli cells, a bacterial promoter region had to be attached to the (be ta ga lac to si dase) human insulin gene. Without it, the E. coli cells would not have recognized the new an enzyme that catalyzes the gene, and it would not have been transcribed and translated into protein. There would conversion of lactose into monosaccharides have been no gene expression. broth (broth) a liquid media used for growing cells agar (a gar) a solid media used RNA repressor for growing bacteria, fungi, plant, regulatory polymerase beta-galactosidase or other cells gene breaks down lactose promoter operator lactose gene B-gal gene gene The regulatory gene The repressor/ When lactose is present, the lactose molecule makes a repressor lactose pair falls binds to the repressor molecule and pulls it off molecule that blocks off the operator, the operator. With the operator cleared, the the operator and turns turning on the RNA polymerase moves down the DNA strand the operon off when structural and will read the beta-galactosidase gene. Beta- lactose is not present. gene(s). galactosidase is made and lactose is broken down for energy. Figure 4.11. Lac Operon. Cell Culture and Sterile Technique To study or manipulate the DNA of bacteria, bacteria cells are needed in large numbers. To grow bacteria cells in the laboratory, a scientist must provide an environment, or medium, that the cells “like.” Some bacteria grow well in a liquid medium (broth). Some bacteria prefer a solid medium, called agar. Some will grow well on or in either. Agar medium is a mixture of water and protein molecules. To prepare it, researchers mix powdered agar in water and heat the mixture until the agar is completely suspended. The agar is sterilized at a high temperature (121°C or higher) at a high pressure of 15 pounds per square inch (psi) or higher for a minimum of 15 minutes (see Figure 4.12). The agar is allowed to cool to about 65°C and is poured under sterile conditions into sterile Petri dishes. The agar cools and solidifies within 15 to 20 minutes, and the poured plates may be used after about 24 hours. Liquid or broth (water and protein molecules) cultures grow as suspensions of millions of floating cells. Starting with sterile broth, the researcher introduces a colony of cells under sterile conditions into the broth. The cells grow and divide and spread themselves throughout the liquid. Broth culture cells reproduce quickly since they have better access to nutrients than do colonies growing on the surface of solid media. Introduction to Studying DNA 119 0112-0147_Biotech2e_Ch04.indd 119 9/13/21 4:06 PM Figure 4.13a. Pouring plates and transferring media in a sterile laminar flow hood (LFH) decreases the chances Figure 4.12. Media, glassware, tubes, and pipet tips can be of contamination. The LFH has a HEPA filter with pores so sterilized in an autoclave (center). An autoclave creates high small that it removes microorganisms from the air before temperature and high pressure to burst all bacteria or fungus the air enters the working area in the hood chamber. cells. Autoclaved tips and tubes that need to dry before use can Everything brought into the hood chamber must be free be placed in a drying oven at 42°C for 24 hours (right). of microorganisms. Photo by author. Photo by author. Oxygen and food diffuse into these cells easily. Under ideal conditions, broth culture media preparation (me di a cells might replicate as often as every 20 minutes. prep ar a tion) the process A technician needs to learn media preparation and sterile technique to be able of combining and sterilizing ingredients (salts, sugars, growth to grow cells in culture. Many kinds of powdered media base can be purchased factors, pH indicators, etc) of and prepared following recipes on the container. An important part of all media a particular medium preparation is sterilizing the medium. The medium, in bottles or plates, must be free autoclave (aut o clave) a n of any unwanted bacteria or fungi before it is used. An autoclave, or pressure cooker/ instrument that creates high temperature and high pressure to sterilizer, is used to heat the medium to over 121°C for a minimum of 15 minutes to sterilize equipment and media destroy any cells or spores. If the medium is to be used in other flasks, bottles, or plates, it must be transferred under sterile conditions to the new sterile vessel (see Figure 4.13a–b). Sterile technique is the process of doing something without contamination by unwanted microorganisms or their spores. Of course, sterile technique is used in surgery. Doctors “scrub up” to get rid of “germs” before they enter the operating room. They also use disinfectants and antiseptic soaps to kill bacteria and fungi on their hands, on the patient’s body, and on working surfaces. Doctors and nurses wear masks and sterile gloves to prevent transmission of microorganisms. All instruments must be sterilized by steam, heat, or radiation so that no disease-causing organisms enter a patient’s body. Technicians must practice sterile technique during cell culture. They must be certain that they are growing only the cells they want to grow (See Figure 4.13a). A single unwanted cell could ruin an experiment or a multimillion-dollar production run. Using a sterile laminar flow hood, disinfectants, sterile tools, sterile media, and attention to detail, technicians can be fairly confident they have not transferred unwanted cells into a culture. Once cells are transferred to a sterile medium, they will start to grow and reproduce. Under ideal conditions, E. coli cells will divide Figure 4.13b. Cell Culture Archivist A Research Associate every 20 minutes. On plate cultures, colonies can be seen within uses sterile technique to collect cells from a plate culture and a day. A broth culture will appear cloudy as more and more cells fill transfer them to long-term glycerol stock storage. Roy Kaltschmidt, Lawerence Berkeley National Lab, it up. Bacterial cell culture concentrations may be checked using a The Regents of the University of California, 2010 Chapter 4 120 0112-0147_Biotech2e_Ch04.indd 120 9/13/21 4:06 PM spectrophotometer (or an instrument that contains a spectrophotometer) or by counting cells on a microscope slide (see Figure 4.14). More information on growing and monitoring cell culture and fermentation (bacterial or fungal cell culture) is presented in Chapters 8 and 9. Eukaryotic DNA Eukaryotic DNA from protist, fungi, plant, and animal cells is similar in many ways to DNA from prokaryotes. Eukaryotic DNA uses the same nucleotide code of A, C, G, and T. Like prokaryotic DNA, eukaryotic DNA has the same double helix structure of repeating nucleotides, with each antiparallel strand bound to the other by H-bonds. DNA from higher organisms, however, is packaged into chromosomes, regulated, and expressed differently from that of bacteria. Most notably, eukaryotes generally have several chromosomes per cell, and each chromosome is a single, linear, very long molecule of DNA coiled around proteins (histones). Each single DNA chromosome may contain several million or more nucleotides and up to many thousands of genes (see Figure 4.15). The genome of eukaryotes is substantially larger than that of prokaryotes (see Figure 4.16). Whereas bacterial cells have only Figure 4.14. In addition to spectrophotometry, one genomic DNA strand per cell, eukaryotic cells contain multiple bacteria cultures can be checked using numbers of chromosomes. Every cell in a multicellular organism’s wet mount slides on high-powered light body has the same number of chromosomes, but depending on the microscopes. Here, a scientist prepares species, the number of chromosomes per cell could be as few as 4 to take a sample of a bacteria colony for microscopic observation. and as many as 100 or more. Humans have 46 chromosomes per cell, iStock.com/tinydevil. with about 3 billion bp making up about 20,000 genes. Fruit flies have 8 chromosomes, and some ferns have more than 1000. However, the total amount of DNA per cell is not directly related to an organism’s complexity. Figure 4.16. The human genome, the total DNA content of a human cell, is 46 chromosomes in 23 Figure 4.15. Arabidopsis plants contain 10 chromosomes pairs. Chromosomes are taken from dividing cells. The in each of their cells. The genetic information stored in the chromosome pairs are lined up from large (Chromosome genes on each of the chromosomes is similar from plant Pair No. 1) to small (Chromosome Pair No. 22). The sex to plant. Changes in the genetic code can lead to mutant chromosomes are the 23rd pair. The chromosome profile organisms, as shown in the plant on the left, which has shown is from a male, since an X chromosome (long) a mutant gene for shortness. and a Y chromosome (short) are shown. Photo by author. iStock.com/Serpil_Borlu. Introduction to Studying DNA 121 0112-0147_Biotech2e_Ch04.indd 121 9/13/21 4:06 PM BIOTECH ONLINE Know Your Genome Go to biotech.myemcp.com/humangenomeproject. Read the article. Write a TO summary of the Human Genome Project (HGP) that addresses the following topics: DO goals of the HGP origin of the HGP and the people involved in launching it When was the HGP completed and how has its completion impacted research? What is in store for us in the future now that we understand the sequence of the human genome? Humans are more complex organisms than ferns, yet we have only a fraction of the amount of DNA per cell. This is because much of eukaryotic DNA is noncoding, meaning it does not transcribe directly into protein. Much of the DNA in higher organisms is spacer DNA within and between genes. There appears to be an evolutionary advantage to widely spaced genes. Genes that are far apart are often involved in recombination, which shuffles forms of a gene from one chromosome to another. This leads to new combinations of genes being sent to sex cells. The end result of variant sex cells is increased diversity in the next generation. Determining the function of spacer DNA is an area of intense interest for researchers. What is its purpose? Why is it there? Why is there so much spacer DNA? Is it just “junk” DNA or does it have some value to the organism or the species and its function is just not yet determined? Another difference between eukaryotic and prokaryotic DNA is the lack of operators in eukaryotes. Like prokaryotic genes, a eukaryotic gene contains a “promoter region,” where an RNA polymerase molecule binds (see Figure 4.17). The RNA polymerase moves along the DNA molecule until it finds the structural gene(s). At the structural gene, the RNA polymerase builds a complementary mRNA transcript from one side of the DNA strand. The enzyme transcribes the entire gene until it reaches a termination sequence. However, eukaryotic genes do not contain operators, so their gene expression is controlled differently than for prokaryotes. Eukaryotic genes are usually “on” and expressed at a very low level. Expression is increased or decreased when molecules interact with enhancer or silencer regions on the eukaryotic DNA strand. The enhancer or silencer regions may be within a gene or somewhere else on the enhancer (en han cer) chromosome. The molecules that bind at enhancer or silencer regions are called a section of DNA that increases the transcription factors (TF). The study of transcription factors in gene regulation and expression of a gene how to control them is a growing area in biotechnology research and development. silencer (si lenc er) a section of DNA that decreases the expression In prokaryotic cells, the mRNA transcript is immediately translated into a of a gene polypeptide at a ribosome. In eukaryotes, though, mRNA transcripts are often transcription factors modified before translation. In eukaryotic DNA, structural genes are composed of (tran scrip tion fac tors) intron and exon sections (refer to Figure 4.17). Exons are the DNA sections that molecules that regulate gene expression by binding onto actually contain the protein code. They are “expressed,” which is why they are called enhancer or silencer regions of exons. A functional mRNA molecule has the complementary code of a gene’s exons. DNA and causing an increase or The introns within the structural gene are sometimes spacer DNA but often are the decrease in transcription of RNA exons for some other protein. A polymerase molecule attaches at the promoter and intron (in tron) the region on a gene that is transcribed into an moves down an entire structural gene, including the intron sections, to produce a long mRNA molecule but not expressed mRNA molecule. Upon completion of the mRNA molecule, the sections on the mRNA in a protein that correspond to introns (noncoding regions) are removed so that only the coding, exon (ex on) the region of exon regions remain on the mRNA molecule. When reassembled, the mRNA molecule a gene that directly codes for a protein; it is the region of the gene is decoded into a protein at a ribosome. Sometimes similar proteins are coded for at that is expressed the same structural gene. The processing of introns differently, removing some and leaving others, can result in different forms of a protein. Chapter 4 122 0112-0147_Biotech2e_Ch04.indd 122 9/13/21 4:06 PM transcription factor enhancer region 3’ 5’ T A T A 3’ promoter 5’ RNA polymerase terminator 3’ 3’ structural genes T T A T T T A A T A A A 5’ 5’ mRNA transcript exon intron Figure 4.17. Eukaryotic Gene. Eukaryotic genes have a promoter to which RNA polymerase binds, but they do not have an operator region. Transcription factors may bind at enhancer regions and increase gene expression. Eukaryotic genes are also regulated by the way the chromosomes are coiled. Chromosomes in higher organisms are highly coiled around structural proteins called histones (his tones) the histones. The histone-DNA complex coils on itself again and again, which conceals nuclear proteins that bind to genes (see Figure 4.18). When genes are buried this way, RNA polymerase cannot chromosomal DNA and condense it get to them to transcribe them into mRNA. The gene has, essentially, been turned into highly packed coils off. DNA has to uncoil all the way to expose the DNA helix to be transcribed and translated to protein. Mammalian Cell Culture Growing mammalian cells in culture is significantly more challenging than growing bacterial cells. This is mainly because under normal circumstances, mammalian cells grow within a multicellular organism. As a part of the whole organism, mammalian cells depend on other cells for several products and stimuli. A biotechnologist growing mammalian cells in culture needs to provide an environment that is an adequate substitute for the normal environment. On a small scale, mammalian cells are typically grown in broth culture in special tubes and bottles with a bottom surface to which the cells can stick (see Figure 4.19). In production facilities, large-scale mammalian cell cultures Figure 4.18. The DNA strand is highly coiled around histone proteins. Extremely coiled, double chromosomes, such as are grown in suspension broth cultures in fermenters. these, are seen during cell division. 500X The media are specifically designed to have all the special © Lester V. Bergman/Corbis/Getty Images. Introduction to Studying DNA 123 0112-0147_Biotech2e_Ch04.indd 123 9/13/21 4:06 PM Figure 4.20. A lab technician checks mammalian cells Figure 4.19. Mammalian cells are viewed on inverted light growing in broth culture in a carbon dioxide incubator. Most microscopes. These have the objectives mounted under mammalian cell cultures are grown at 37°C and 5% CO2. The the stage, closer to the mammalian cells on the bottom of cultures need to be reseeded (restarted) in fresh, sterile media the culture flask. The rate of cell growth and the health of every few days to give them more food and nutrients, and the cells can be checked. a red indicator solution is used to to prevent the buildup of toxic waste products. A phenol red monitor the pH. If the pH of the growth medium falls outside indicator, added to the media, changes color as the media’s a narrow pH range, the indicator will change colors. pH changes. Photo by author. Photo by author. nutrients that each cell type may require. Special indicators may be added to monitor the culture. Phenol red is one such indicator. It changes from red to gold as the solution becomes acidic from cell overcrowding (see Figure 4.20). Viral DNA Although scientists do not consider them to be living things, viruses infect organisms nonpathogenic and are often the target of biotechnology therapies. In addition, nonpathogenic (non path o gen ic) not viruses or virus particles are often used in biotechnology research as vectors to carry known to cause disease DNA between cells. For these reasons, understanding the structure of viruses and bacteriophages (bac ter i o phag es) the their genetic information is important. viruses that infect bacteria Viruses do not have cellular structure. They are collections of protein and nucleic acid molecules that become active once they are within a suitable cell. Viruses are very tiny, measuring from 25 to 250 nm (a nanometer = 1 millionth of a millimeter). Viruses are classified into the following three main categories, based on the type of cell they attack: bacterial (bacteriophages) plant animal Figure 4.21 shows a herpes infection, which is caused by a type of animal virus. Viruses are classified further based on the specific type of cell infected and on other characteristics, such as their genetic material and shape (see Table 4.2). No matter the type, all virus particles have a thick protein coat surrounding a nucleic acid core Figure 4.21. An animal virus causes the sores in oral of either DNA or RNA. herpes infection. © Dr. Milton Reisch/Corbis/Getty Images. Chapter 4 124 0112-0147_Biotech2e_Ch04.indd 124 9/13/21 4:06 PM Table 4.2. Examples of Viruses and Their Characteristics Virus Example of Host Cells Infected Shape Type of Nucleic Acid Herpes Simplex I human nerve and epithelial cells spherical double-stranded DNA Parvovirus human bone, blood, and cardiac cells spherical single-stranded DNA Reovirus mouse heart cells icosahedral double-stranded RNA Tobacco Mosaic tobacco and tomato, etc rod-shaped single-stranded RNA HIV human immune cells spherical single-stranded RNA Within a cell, the nucleic acid of a virus is released. The viral genes are read by the host cell’s enzymes, decoded into viral mRNA, and translated into viral proteins. New gene therapy (gene ther a py) virus particles are assembled and released, and may infect other cells. Some viruses, the process of treating a disease or disorder by replacing for example, the lysogenic viruses, incorporate their DNA into the host chromosome, a dysfunctional gene with and some, for example, the lytic viruses, do not. a functional one The structure of the virus particle is important in trying to control viruses. Many therapies utilize the fact that the human immune system can recognize virus surface proteins. Viral vaccine molecules recognize specific viral surface proteins and target them for attack. Addtionally, some biotechnology companies are developing protease inhibitors as an additional way to fight viral infection. Protease inhibitors destroy proteases made by viruses in their attempts to take over host cells. Blocking or destroying viral DNA, RNA, or protein molecules is a broadening area of disease therapy (see Figure 4.22). Viral DNA or RNA molecules are relatively short and easy to manipulate, since viruses do not produce very many proteins compared with cells. Like plasmid DNA, viral DNA molecules are often used as vectors. They may be cut open to insert genes of interest. When sealed, they become recombinant molecules that can be inserted into new cells. If the recombinant molecules are inserted back into virus-protein coats, the viruses themselves can insert the rDNA into an appropriate host cell (see Figure 4.23). Recombinant virus technology is one technique used in a process called gene therapy. Viruses can insert corrective genes (the “therapy”) into cells that contain defective genes. Several companies are exploring the use of gene therapy to treat diabetes by replacing defective insulin genes in the pancreas. Gene therapy is also a possible treatment for cystic fibrosis and other genetic disorders. Although many biotechnology companies are developing gene therapies (over 400 as of 2015) to treat an assortment of disorders, to date only a few gene therapies are actually on the market. Gene therapy is discussed in more detail in later sections. Figure 4.22. Coronaviruses are a group of viruses that have a halo or crown-like (corona) appearance due to spike-like projections. In 2005, new evidence showed that a new coronavirus is the cause of Figure 4.23. In France, scientists at The Institut Gustave severe acute respiratory syndrome (SARS). The protein spikes could Roussy transform mice with viral vectors carrying genes for be a target for potential therapy. ~100,000X tumor suppression or other anti-cancer proteins. © Mediscan/Corbis. © Philippe Eranian/Corbis/Getty Images. Introduction to Studying DNA 125 0112-0147_Biotech2e_Ch04.indd 125 9/13/21 4:06 PM BIOTECH ONLINE Using Viruses to Do Good The word “virus” often initiates a justifiable negative response including worries of serious illness. Many viruses are responsible for diseases that cause significant sickness and even death. However, biotechnologists are learning how to manipulate some viruses to do no harm and actually to do some good. Specifically, a team has taken the poliovirus that until the 1950s, caused widespread paralysis and even death, and engineered it to fight brain cancer. 1. Go to biotech.myemcp.com/viralzone and pick a human virus to learn more about. Pick one TO that is spread between other animals and humans. Click the link and read and record three DO things about the virus’ characteristics or behavior. 2. Learn more about the poliovirus using information from the website, biotech.myemcp.com/ poliovirus. Describe how the poliovirus infects cells 3. Go to biotech.myemcp.com/poliobraincancer and read the article Polio Virus May Cure Brain Cancer Thanks To Genetic Re-Engineering. Describe how and why genetically-engineered poliovirus may be able to kill brain cancer cells. Section 4.2 Review Questions 1. Plasmids are very important pieces of DNA. How do they differ from chromosomal DNA molecules? 2. Bacteria cell DNA is divided into operons. Describe an operon using the terms promoter, operator, and structural gene. 3. Describe the human genome by discussing the number and types of chromosomes, genes, and nucleotides. 4. What is gene therapy? Cite an example of how it can be used. 4.3 Isolating and Manipulating DNA Modifications of DNA can be as simple as changing a single nitrogen base (A, C, G, or T) in a gene sequence, or as complicated as cutting out entire genes or gene sections and inserting new ones (genetic engineering). Changing DNA sequences may alter the production of proteins in a cell or an organism. New proteins may be created, or the production of some proteins may be arrested. The characteristics of cells or whole organisms may be affected by the change in the protein population. The term “genetic engineering” was first used to describe the production of rDNA molecules (pieces of DNA cut and pasted together) and their insertion into cells. The cells were considered genetically engineered because their genetic code and, thus, their protein production, had been manipulated. Now, the phrase “genetic engineering” is used to describe virtually all directed modifications of the DNA code of an organism. Keep in mind that when scientists attempt to alter the genetic code the goal is to alter protein production. The process of genetic engineering, by any method, requires the following steps: 1. Identification of the molecule(s), produced by living things, which could be produced more easily or economically through genetic engineering; for example, insulin for diabetic patients. 2. Finding and isolating the DNA and gene sequence for the target product molecule; for example, finding the human insulin gene in DNA isolated from human cells. Chapter 4 126 0112-0147_Biotech2e_Ch04.indd 126 9/13/21 4:06 PM 3. Manipulation of the DNA instructions by either changing them inside the cell/ organism or by putting those instructions into another organism/cell that can produce the molecule more easily, in larger amounts, or less expensively. For example, the human insulin gene is pasted into a plasmid and inserted into E. coil cells. 4. Harvesting of the molecule or product, testing it, and marketing it to the public (for commercial products). For example, recombinant human insulin is recovered from the fermentation tanks growing huge volumes of transformed E. coli cells. The insulin product is tested and formulated for distribution as a therapeutic drug, such as Humalog® by Eli Lilly and Company. Recombinant DNA Technology Recombinant DNA technologies are the methods used to create new DNA molecules by piecing together different DNA molecules. When cells accept rDNA and start expressing the new genes by making new proteins, they are considered genetically engineered. When written by scientists, the names of proteins or DNA produced in this way are written with a “r” in front of them. For example, rInsulin is recombinant insulin, and rhInsulin stands for recombinant human insulin. Many items currently on the market are produced through rDNA technology. These products are all versions of naturally occurring molecules or organisms improved through genetic engineering. Some well known examples of items produced through rDNA technology include the following: rhInsulin (Humalog®, marketed by Eli Lilly and Company), recombinant human growth hormone (Nutropin®, marketed by Genentech, Inc.), recombinant gamma interferon (marketed by Genentech, Inc.), recombinant HER2 antibody Figure 4.24. Blood clots can occur and block blood vessels (produced by Genentech, Inc.), and recombinant rennin during some heart attacks and strokes. To make the blockages (chymosin or ChyMax®, manufactured by Pfizer, Inc.). visible, doctors inject dye into the blood vessels in a process Each of these recombinant DNA products is either called angiography. If a heart attack occurs, the enzyme, Activase® (recombinant t-PA), may be injected to help synthesized in too small a quantity in the organism, “dissolve” the blockage. synthesized at the “wrong” time, or lacks an important © Lester Lefkowitz/Corbis. characteristic. For example, tissue plasminogen activator (t-PA) is a naturally occurring protein that decreases the time it takes to dissolve blood clots. The human body makes t-PA only in very small amounts. Scientists quickly realized that if t-PA could be made on a large enough scale, it could be used to treat blood clots that occur during some heart attacks and strokes. The product is now marketed under the trade name Activase®, by Genentech, Inc. (see Figure 4.24). Constructing rDNA requires the isolation of DNA molecules. For the production of t-PA, two kinds of DNA molecules had to be isolated: human genomic DNA containing the t-PA gene and a bacterial plasmid DNA for use as a vector. First, the human t-PA gene is identified. The t-PA gene has several introns. The mRNA exon sequence is used to produce a piece of complementary DNA (cDNA). Next, the human t-PA cDNA and the vector plasmid are each cut with a restriction enzyme and then pasted together using DNA Figure 4.25. A lab technician monitors the cells growing ligase. The bacterial plasmid carrying the human t-PA genetic in broth culture. The mammalian cells in the fermentation information is inserted into appropriate cells, in this case, flask on the left are making human proteins for therapeutic Chinese hamster ovary (CHO) cells growing in broth culture purposes. When enough protein is produced, the protein is (see Figure 4.25). The CHO cells then transcribe and translate separated from the cells and all other contaminants. Photo by author. the human t-PA gene and produce the human protein in the CHO cells. The t-PA molecules are harvested from the CHO broth culture and formulated for sale. Introduction to Studying DNA 127 0112-0147_Biotech2e_Ch04.indd 127 9/13/21 4:06 PM BIOTECH ONLINE Recombinant Pharmaceuticals – Designed to Take Your Breath Away? Do you have asthma or do you know someone who does? Chances are the answer is yes. According to the American College of Chest Physicians, 10% of North Americans have asthma. Several biotechnology companies are developing products to interrupt the cascade of events that result in asthmatic symptoms. Some of these asthma therapies use recombinant protein products produced through genetic engineering. Learn more about asthma and a genetically engineered product used to treat it. TO Use the Internet to answer the following questions. In your notebook, record your DO answers and the websites you used. 1. Define/describe the causes and symptoms of asthma. 2. Learn about Xolair® (omalizumab) by Genentech USA, Inc. and Novartis Pharmaceuticals Inc. a genetically engineered human antibody that is used as an asthma medication. Naturally produced by humans, the omalizumab protein is manufactured by Chinese hamster ovary cells grown in culture. Do a search on the Internet to find our how it works and some of the pros and cons of its use. Site-Specific Mutagenesis The phrase “site-specific mutagenesis” or “site-directed mutagenesis” refers to site-specific mutagenesis (site- the process of inducing changes (mutagenesis) in certain sections (site-specific) of a spe ci fic mu ta gen e sis) particular DNA code. The changes in the DNA code are usually accomplished through a technique that involves changing the use of chemicals, radiation, or viruses. Bacteria, fungi, plant, or cell cultures may the genetic code of an organism (mutagenesis) in certain sections be treated with one or more mutagens. Exposure of bacteria colonies to different (site-specific) of the genome amounts of ultraviolet (UV) light radiation, for example, can cause increased cell growth, new pigment production, or even cell death, to name just a few outcomes. Mutagenic agents have varied effects, depending on the type and amount of exposure. They may cause substitutions of one base for another, for example, an “A” for a “G.” A substitution may cause a positive or negative change in a protein’s structure or function. Some mutagens cause additions, or deletions, to large sections of the genetic code, which may alter or prevent a protein’s production. Sometimes site-specific mutagenesis is “directed,” meaning a scientist is trying to make certain changes in a protein’s structure that will translate into an improved function. Such is the case with the protein, subtilisin, marketed by Genencor International. Subtilisin is an enzyme that degrades other proteins (it is a type of protease). It is added to laundry detergent to remove proteinaceous stains, such as blood or gravy, from soiled clothing (see Figure 4.26). To improve the activity of subtilisin, fungi were treated with chemicals that caused changes in their subtilisin DNA code. A random change in DNA code led to a change in protein structure such that the protein would work more effectively in the alkaline (high pH) detergent solution. Since mutagenic agents act rather randomly, the outcome often is unexpected. The mutagenesis may stunt or inhibit product production. Sometimes genetic engineers get new or improved products. At Genencor International, Inc. and other companies with a similar focus, scientists screen large Figure 4.26. Tide® laundry detergent by Procter & Gamble libraries of mutated fungi and bacteria while looking for new contains rSubtilisin, a recombinant protease that helps remove or improved ways to produce a product. blood, gravy, and other protein-based stains from clothing. Photo by author. Chapter 4 128 0112-0147_Biotech2e_Ch04.indd 128 9/13/21 4:06 PM Gene Therapy Gene therapy is the process of correcting or modifying DNA codes that cause genetic diseases and disorders. There are two common ways to introduce new genes into defective cells. The first is to use a virus to carry a new or normal gene into target cells. A second way is to package a “good” gene in a synthetic lipid envelope (liposome) and use the envelope to bring the gene into a cell. By either method, the hope is that the gene inserts into a chromosome, is transcribed into mRNA and produces the needed functional proteins. One of the first attempts at human gene therapy was with cystic fibrosis (CF). CF causes a buildup of thick mucus that clogs the respiratory and digestive syst