Infrared Spectroscopy (IRS) Past Paper PDF
Document Details
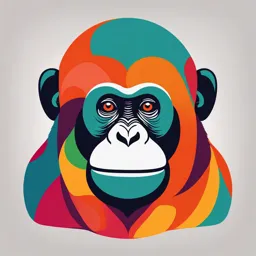
Uploaded by InvaluableViolet5195
University of Pretoria
SCACA3-44
Tags
Related
Summary
This document covers the principle of infrared spectroscopy (IRS), including its interaction with bonds and a table of IR absorption values. It also details instrumentation and applications. It is a likely part of a larger analytical chemistry course.
Full Transcript
Analytical Chemistry SCACA3-44 WEEK 4: INFARED SPECTRAS PRINCIPLE Principle: Infrared spectroscopy (IRS): measures how molecules absorb infrared light, causing specific vibrations in bonds between atoms. This absorption is direct...
Analytical Chemistry SCACA3-44 WEEK 4: INFARED SPECTRAS PRINCIPLE Principle: Infrared spectroscopy (IRS): measures how molecules absorb infrared light, causing specific vibrations in bonds between atoms. This absorption is directly related to the bond type and the atoms involved. Infrared radiation in the range of 400 cm⁻¹ to 4000 cm⁻¹ is used. Bonds in molecules absorb radiation at specific wavelengths, causing them to stretch, bend, or vibrate. Interaction: Bonds with a dipole moment (unequal distribution of charge) absorb IR radiation, while symmetrical bonds like O₂ and N₂ do not. Asymmetric stretching, bending, and wagging motions are common types of molecular vibrations. IR ABSORPTION TABLE IR Absorption Table: This table categorizes the wavelengths (or wavenumbers) where diOerent bonds within functional groups absorb IR radiation. For instance: o O-H stretching: Absorbs around 3200-3600 cm⁻¹ (broad peak for alcohols, acids). o C=O stretching: Absorbs around 1700 cm⁻¹ (sharp peak for carbonyl groups). o C-H stretching: Absorbs around 2800-3000 cm⁻¹. Usage: By analyzing an IR spectrum and referring to this table, specific functional groups (e.g., alcohols, carbonyls, amines) within a molecule can be identified based on their characteristic absorption bands. Analytical Chemistry SCACA3-44 Principle: IR spectroscopy is based on the absorption of specific frequencies of light by bonds within a molecule. These frequencies correspond to the bond's characteristic structure and cause bending, stretching, or wagging motions. Energy Absorption: Bonds absorb energy like springs when excited. This only occurs for bonds with a dipole moment (between atoms of diOerent electronegativities). Symmetrical Bonds: Do not absorb IR radiation (e.g., O₂, N₂), while most organic molecules with asymmetrical bonds do. Key Points: The intensity of absorption depends on the dipole moment, which is influenced by the diOerence in electronegativity between atoms in the bond. Greater electronegativity diOerences result in stronger absorption. Spectra Interpretation: Functional Groups: Specific functional groups absorb at characteristic wavenumbers. A reference table for these absorption ranges is typically provided in exams. Plot: IR spectra plot transmittance against wavenumber, where peaks represent diOerent bond types and molecular functions. Applications: Molecular Fingerprinting: Each molecule has a unique IR spectrum, acting as a "fingerprint." This allows unknown substances to be identified by comparing their spectra to a database of known compounds. Analytical Chemistry SCACA3-44 Visual Resources: The spectrum of hexanoic acid is an example, showing characteristic peaks for O-H and C=O groups. INSTRUMENTATION - IR SPECTROMETER Schematic Overview: The instrument setup includes several critical components: o Radiation source: Provides IR light. o Sample compartment: Holds the sample, either solid, liquid, or gas, prepared using various techniques. o Monochromator: Filters the IR light into specific wavelengths. o Detector: Measures how much light is transmitted or absorbed by the sample. o Computer/Display: Converts detector data into an IR spectrum. Analytical Chemistry SCACA3-44 FUNCTION AND SPECIFICATIONS OF INSTRUMENT COMPONENTS Radiation source: Typically an inert solid material like a ceramic or Nernst glower that heats up and emits infrared radiation. The radiation covers the infrared region (usually 400 cm⁻¹ to 4000 cm⁻¹) needed to excite molecular bonds. Sample cells and sample preparation: Sample cells vary for diOerent states of matter: o Solids: Typically ground with KBr and pressed into pellets or run as thin films. o Liquids: Often placed between NaCl or KBr discs or dissolved in non-polar solvents like CCl₄. o Gases: Contained in gas cells with windows made of IR-transparent materials like NaCl. o Sample preparation is critical to ensure the correct interaction with IR light and avoid artifacts. Monochromator: A device (usually a diOraction grating) that selects specific wavelengths from the source radiation and directs it at the sample. It enables scanning across the entire IR range to generate a full spectrum. Detector: DiOerent detectors capture how much of the IR light is absorbed by the sample. Common types include thermocouples or pyroelectric detectors, which are sensitive to changes in temperature or light intensity. The detector records the intensity of the transmitted light and generates a signal. Spectra generated by detector: The IR spectrum is a graph plotting % transmittance (how much light passes through the sample) against wavenumber (cm⁻¹). Peaks represent the absorption of energy by bonds at specific wavenumbers, corresponding to functional groups in the molecule. HOW IT WORKS AND APPLICATIONS HOW IT WORKS Infrared light is passed through a sample. The sample absorbs specific frequencies of the light, corresponding to the vibrational energies of the bonds within the molecule. These vibrations may involve stretching, bending, or twisting motions depending on the bond type. The light that passes through the sample (i.e., is not absorbed) is measured by a detector, and an absorption spectrum is created, showing peaks at the wavelengths where absorption occurred. Each peak correlates to a specific bond or functional group. APPLICATIONS Organic Chemistry: Identifying functional groups such as alcohols, esters, and ketones. Pharmaceuticals: Quality control and verification of drug structure. Material Science: Analyzing polymers, coatings, and other materials to confirm their structure. Food Industry: Monitoring product quality (e.g., fat content, water content). Environmental Science: Detecting gases like CO₂, CH₄ in atmospheric studies. Analytical Chemistry SCACA3-44 STRENGTHS AND LIMITATIONS STRENGTHS Non-destructive: Does not alter or destroy the sample, allowing for archival storage and reanalysis. Broad applicability: Can analyze solids, liquids, and gases with minimal sample preparation. Unique spectral fingerprint: Each molecule produces a unique IR spectrum, making the technique highly specific for compound identification. Rapid analysis: Relatively fast method with results generated in minutes. LIMITATIONS Qualitative, not quantitative: IR is mainly used to identify the presence of functional groups rather than to quantify them. Sample preparation: Some preparation techniques (e.g., pressing solids into KBr pellets) can introduce errors or artifacts. Weak for symmetrical molecules: Bonds with no dipole moment, like O₂ or N₂, do not absorb IR radiation and thus cannot be analyzed with this technique. Overlapping peaks: Complex molecules with many bonds can produce overlapping peaks, making interpretation challenging without additional techniques like NMR or MS for confirmation. Analytical Chemistry SCACA3-44 WEEK 4: MOLECULAR EMISSION SPECTROSCOPY (MES) Molecular Emission Spectroscopy (MES): also known as molecular fluorescence spectroscopy, involves the absorption of light by molecules, which excites electrons to a higher energy state. As these electrons return to their ground state, they emit light at a longer wavelength. The emitted light or fluorescence is measured, which is dependent on the structural properties of the molecules. Fluorescence is particularly useful for compounds with a rigid structure or chromophores, and it can provide highly sensitive detection for various biological and chemical processes. Molecular emission spectroscopy focuses on the ability of certain molecules (especially those with chromophores or rigid structures) to absorb light at shorter wavelengths and then emit light at longer wavelengths as their electrons return to their ground state. This emission is called fluorescence. o Fluorescent Molecules: Excited electrons emit radiation at a lower energy (longer wavelength) due to the gradual release of energy in the form of vibrational energy. o Non-Fluorescent Molecules: Energy absorption and emission occur at the same wavelength because the energy change remains the same. GRAPHICAL REPRESENTATION Stokes Shift: The diOerence between the absorption wavelength (shorter, higher energy) and the emission wavelength (longer, lower energy), typically between 50- 150 nm. Instrumentation: The emission spectra produce two peaks: o Absorption Peak: At a shorter wavelength, higher energy. o Emission Peak: At a longer wavelength, lower energy, and lower intensity. Analytical Chemistry SCACA3-44 HOW IT WORKS COMPARED TO FLAME EMISSION SPECTROSCOPY MES: Excited molecules emit radiation at longer wavelengths (fluorescence) after absorbing light. MES involves excitation via photons, and emission is observed as the electrons return to the ground state. The intensity of fluorescence is proportional to the concentration of the analyte. FES (Flame Emission Spectroscopy): In FES, atoms or ions are excited by thermal energy (flame) and emit radiation as they return to their ground state. FES measures atomic emission rather than molecular fluorescence. APPLICATIONS OF MES MES is widely used in: Drug discovery for identifying molecules with specific fluorescent properties. Protein and enzyme studies to detect changes in molecular structures. Fluorescence microscopy for visualizing biological samples. Material sciences for studying molecular properties. Forensic sciences for detecting substances like drugs or explosives. STRENGTHS AND LIMITATIONS STRENGTHS High sensitivity and specificity for detecting fluorescent molecules. Non-invasive and applicable to biological, chemical, and material sciences. Allows real-time monitoring of processes. LIMITATIONS Interference by environmental factors (e.g., concentration, heavy atoms, temperature). Not all molecules are naturally fluorescent, and some require chemical modifications. Less suited for non-fluorescent compounds compared to techniques like UV-Vis spectroscopy or FES. Analytical Chemistry SCACA3-44 INSTRUMENTATION AND FUNCTIONALITY OF COMPONENTS INSTRUMENT USED = FLUORIMETER 1. Light Source (Quartz Halogen Lamp): Provides a continuous light source for exciting the sample. In some cases, xenon arc lamps or lasers can be used for more intense or pulsed light. 2. Monochromators (Two): o Excitation Monochromator: Selects the specific wavelength of light to excite the molecules. o Emission Monochromator: Filters the emitted fluorescence to measure a specific wavelength. 3. Detector (Photomultiplier Tube/Photodiode): Measures the intensity of emitted fluorescence, which is used to quantify the analyte. INTERFERENCE FACTORS IN MES 1. Concentration: At high concentrations, the fluorescence intensity may decrease due to self-quenching or reabsorption of emitted light (inner filter eOect). 2. Heavy Atoms in Solution: Heavy atoms can promote intersystem crossing, reducing fluorescence and increasing phosphorescence. 3. Viscosity: Increased viscosity can reduce the rate of non-radiative decay, enhancing fluorescence by limiting molecular motion. 4. Temperature: Higher temperatures can enhance non-radiative decay, reducing fluorescence intensity. 5. pH of Solution: The pH can alter the ionization state of the molecule, which may aOect fluorescence properties. 6. Complex Formation: Binding with other molecules (e.g., metal ions) can either enhance or quench fluorescence, depending on the nature of the interaction.