Spectrochemical Analysis PDF
Document Details
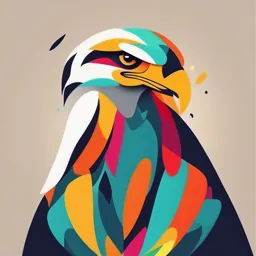
Uploaded by DauntlessMorningGlory773
Cyprus International University
Tags
Summary
This document details spectrochemical analysis, covering instrument components, types of spectroscopy, and optical materials. It discusses the principles and components of spectroscopic instruments used in the UV/visible and IR regions, alongside various types of spectroscopy, and materials used in optical instruments. The document also introduces spectroscopic sources, monochromators, and the concept of detecting and measuring radiant energy.
Full Transcript
Chapter 25 Spectrochemical Analysis 1 Instrument Components Most spectroscopic instruments in the UV/visible and IR regions are made up of five components: (1) a stable source of radiant energy (line or contiuum).; (2) a wavelength selector to isolate...
Chapter 25 Spectrochemical Analysis 1 Instrument Components Most spectroscopic instruments in the UV/visible and IR regions are made up of five components: (1) a stable source of radiant energy (line or contiuum).; (2) a wavelength selector to isolate a limited region of the spectrum for measurement (prism, grating, filter etc).; (3) one or more sample containers; transparent container used to hold the sample (eg. Cells, cuvettes, etc) (two for double-beam instruments) (4) a radiation detector, to convert radiant energy to a measurable electrical signal (photon or heat detectors) (5) a signal-processing and readout unit; amplifies or attenuates the transduced signal and sends it to a readout device consisting of electronic hardware and in modern instruments a computer. 2 https://www.youtube.com/watch?v=pxC6F7bK8CU 3 TYPE OF SPECTROSCOPY Spectroscopic measurements are made on three different kinds matter/radiation interactions. These are; (1) ABSORPTION (transmittance) of radiation (UV/visible and IR) by atoms, ions or molecules. (2) FLUORESCENCE of radiation by molecules. (3) EMISSION of radiation by atoms and ions. The basic arrangement of the main components of various instruments used in different types of «optical» spectroscopy are shown in figures a, b and c. 4 a) Absorption measurements: Selected wavelength is sent through the sample, and the transmitted radiation is measured by the detector /signal processing /readout unit. b) Fluorescence measurements: Two wavelength selectors needed; to select the excitation and the emission wavelengths. Excitation and emitted at right angles to avoid detecting the source radiation and to minimize scattering. c) Emission spectroscopy: A source of thermal energy, such as flame, produces an analyte vapor that emits radiation isolated by the wavelength selector and converted to an electrical signal by the detector. 5 «Optical» Materials Cells,windows,lenses,mirrors, and wavelength selecting elements in an optical spectroscopic instrument must transmit radiation in the wavelength region being employed. Ordinary silicate glass is OK for visible region and is cheap. Glass absorbs at wavelengths not shorter than 340 nm therefore fused silica or must be substituted. Also, glass, quartz, and fused silica all absorb in the IR region at wavelengths longer than about 2.5 µm. Hence, optical elements for IR spectrometry are typically made from halide salts. 6 1.Plastic cuvettes are often used in fast spectroscopic assays, where high speed is more important than high accuracy. Plastic cuvettes with a usable wavelength range of 380–780 nm (the visible spectrum). They are cheap to manufacture and purchase. 2.Glass cuvettes has an optimal wavelength range of 340–2500 nm. Glass cuvettes are typically for use in the wavelength range of visible light. 3.Quartz Cuvettes cells provide more durability than plastic or glass. Quartz excels at transmitting UV light, and can be used for wavelengths ranging from 190 to 2500 nm 7 Spectroscopic Sources To be suitable for spectroscopic studies, a source must generate a beam of radiation that is sufficiently powerful for easy detection and measurement. In addition, its output power should be stable for reasonable periods of time. Spectroscopic sources are of two types: continuum sources, which emit radiation that changes in intensity only slowly as a function of wavelength. line sources, which emit a limited number of spectral lines, each of which spans a very narrow wavelength range. 8 Spectra of two different spectral sources. The spectrum of a continuum source (a) is much broader than that of a line source (b). 9 Monochromators Entrance Slit: This is where the light enters the monochromator. It controls the amount of light that enters the system. Collimating Lens or Mirror: After passing through the entrance slit, the light encounters a collimating lens or mirror that makes the light rays parallel. This helps maintain the integrity of the spectrum. Dispersion Element: This could be a diffraction grating, prism, or sometimes a prism/grating combination. It disperses the incoming light into its various wavelengths. Focusing Lens or Mirror: Once the light is dispersed, it needs to be focused onto the exit slit. This component focuses the dispersed wavelengths onto the exit slit. Exit Slit: This selects the desired wavelength or range of wavelengths that will pass through for analysis or further use. 10 Monochromator generally employ a diffraction grating to disperse the radiation into its component wavelengths. Older instruments used prisms for this purpose. By rotating the grating, different wavelengths can be made to pass through an exit slit. The output wavelength of a monochromator is thus continuously variable over a considerable spectral range. The wavelength range passed by a monochromator, called the spectral bandpass or effective bandwidth, can be less than 1 nm for moderately expensive instruments to grater than 20 nm for inexpensive systems. 11 Detecting and Measuring Radiant Energy To obtain spectroscopic information, the radiant power transmitted, fluoresced, or emitted must be detected in some manner and converted into a measurable quantity. A detector is such a device. In modern instruments, the information of interest is encoded and processed as an electrical signal. The term transducer is used to indicate the type of detector that converts quantities, such as light intensity, pH, mass, and temperature, into such electrical signals that can be subsequently amplified, manipulated, and finally converted into numbers proportional to the magnitude of the original quantity. 12 Single-Beam Instruments The simplest type of spectrometer employs a single source to supply radiation to the sample and then to the background in turn; for example, in some UV-visible absorbance measurements or in plasma emission spectrometry. 13 Advantages of Single Beam Systems Single beam instruments are less expensive High energy throughput due to non-splitting of source beam results in high sensitivity of detection Disadvantages Instability due to lack of compensation for disturbances like electronic circuit fluctuations, voltage fluctuations, mechanical component’s instability or drift in energy of light sources. Such drifts result in abnormal fluctuations in the results. 14 Double-Beam Instruments A double beam instrument takes the initial powers and splits the beam so that the light going through the sample has a constant reference beam that is not going through the sample. This allows for internal compensation for any change in light intensity In order to make rapid, accurate comparisons of a sample and a reference, double-beam instruments are frequently used. Since it is essential that the two beams are as similar as possible, a single source is used and the optics arranged to pass equal intensities of the beam through the sample area and through the reference area, and then to disperse and detect them alternately. 15 Advantages of Double Beam Systems Modern improvements in optics permit high level of automation and offer the same or even better level of detection as compared to earlier single beam systems. Instability factors due to lamp drift, stray light, voltage fluctuations do not affect the measurement in real-time. Little or no lamp warm up time is required. This not only improves throughput of results but also conserves lamp life 16 Ultraviolet and Visible Molecular Absorption Spectroscopy Several types of molecular species absorb ultraviolet and visible radiation. Molecular absorption by these species can be used for qualitative and quantitative analyses. Uv/visible absorption is also used to monitor titrations and to study the composition of complex ions. 17 Absorption by Organic Compounds Absorption of radiation by organic molecules in the wavelength region between 180 and 780 nm results from interactions between photons and electrons that either participate directly in bond formation or that are localized ( unbonding electrons) about such atoms as oxygen, sulfur, nitrogen, and the halogens. The wavelength of absorption of an organic molecule depends on how tightly its electrons are bound. The shared electrons in carbon- carbon or carbon-hydrogen single bonds are so firmly held that their excitation requires energies corresponding to wavelengths in the vacuum ultraviolet region below 180 nm. Single-bond spectra have not been widely exploited for analytical purposes because of the experimental difficulties of working in this region. 18 Absorption by Organic Compounds Electrons in double and triple bonds of organic molecules are not as strongly held and are therefore more easily excited by electromagnetic radiation. Thus, species with unsaturated bonds generally exhibit useful absorption bands. Unsaturated organic functional groups that absorb in the ultraviolet or visible regions are known as chromophores. Chromophores are unsaturated organic functional groups that absorb in the ultraviolet or visible region. 19 How to choose appropriate solvent for the analysis A solvent for ultraviolet/visible spectroscopy must be transparent in the region of the spectrum where the solute absorbs. The analyte must be sufficiently soluble in the solvent to give a well-defined analyte. In addition, we must consider possible interactions of the solvent with the absorbing species. For example, polar solvents, such as water, alcohols, esters, and ketones, tend to obliterate (corrode) vibrational fine structure and should thus be avoided to preserve spectral detail. Spectra in nonpolar solvents, such as cyclohexane, often more closely approach gas-phase spectra. In addition, solvent polarity often influences the position of absorption maxima. For qualitative analysis, analyte spectra should thus be compared to spectra of known compounds taken in the same solvent. 20 Quantitative applications Ultraviolet and visible molecular absorption spectroscopy is one of the most useful tools available for quantitative analysis. The important characteristics of spectrophotometric and photometric methods are Wide applicability. A majority of inorganic, organic, and biochemical species absorb ultraviolet or visible radiation and are thus amenable (responsible) to direct quantitative determination. Of the determinations performed in clinical laboratories, a large majority is based on ultraviolet and visible absorption spectroscopy. High sensitivity. Typical detection limits for absorption spectroscopy range from 10-4 to 10-5 M. This range can often be extended to 10-6 or even 10-7 M with procedural modifications. 21 Moderate to high selectivity. Often a wavelength can be found at which the analyte alone absorbs. Furthermore, where overlapping absorption bands do occur, corrections based on additional measurements at other wavelengths sometimes eliminate the need for a separation step. Good accuracy. The relative errors in concentration encountered with a typical spectrophotometric or photometric procedure lie in the range from 1% to 5%. Such errors can often be decreased to a few tenths of a percent with special precautions. Ease and convenience. Spectrophotometric and photometric measurements are easily and rapidly performed with modern instruments. In addition, the methods lend themselves to automation quite nicely. 22 Calibration curve method A general method for determining the concentration of a substance in an unknown sample is the comparing the unknown to a set of standard sample of known concentration. 23 How to measure the concentration of unknown? https://www.youtube.com/watch?v=8efZRBCFpRg Practically, you have measure the absorbance of your unknown. Once you know the absorbance value, you can just read the corresponding concentration from the graph. 24 Drawing a Calibration Curve ABSORBANCE ∝ CONCENTRATION and should be linear relationship Prepare 3-5 standards of the analyte to be quantified at known concentrations and measure absorbance at a specified wavelength. Prepare calibration curve. Concentration of analyte in sample can be obtained from the calibration curve. Molar absorptivity can be obtained from the slope of the calibration curve for a given wavelength (𝞴) 25 At high concentrations the calibration curve may deviate from linearity – Always ensure your concentration of the sample falls within the linear range – if necessary dilute sample. Absorbance not to exceed 1. CHOOSE CORRECT WAVELENGTH An analyte may give more than one absorbance maxima (ʎmax) value. Note: Many compounds absorb at 220-230 nm hence do not use A. Need to choose wavelength more specific to compound (SELECTIVITY) and if more than one, select one with highest absorbance as this gives less error. Therefore, we should use C. 26 27 Infrared Spectroscopy 28 Infrared Absorption Spectroscopy Infrared spectroscopy is a powerful tool for identifying pure organic and inorganic compounds because, with the exception of a few homonuclear molecules such as O2, N2, and Cl2, all molecular species absorb infrared radiation. In addition, with the exception of chiral molecules in the crystalline state, every molecular compound has a unique infrared absorption spectrum. Therefore, an exact match between the spectrum of a compound of known structure and the spectrum of an analyte unambiguously identifies the analyte. Infrared spectroscopy is a less satisfactory tool for quantitative analyses than its ultraviolet and visible counterparts because of lower sensitivity and frequent deviations from Beer’s law. Additionally, infrared absorbance measurements are considerably less precise. 29 Infrared Absorption Spectra The energy of infrared radiation can excite vibrational and rotational transitions, but it is insufficient to excite electronic transitions. As shown in figure, infrared spectra exhibit narrow, closely spaced absorption peaks resulting from transitions among the various vibrational quantum levels. 30 Determination of structural formula from molecular formula 1- calculate double bond equivalent no of C atom - ½ x ( no of H+ halogen atom) + ½ x no of N atom + 1 Ex: C6H5Cl Double bond equivalent: 6 – ½ x (5 + 1) + 1 = 4 If Double bond equivalent 4, this mean that , your molecule contain aromatic benzene ring. 2- Determine Functional group on IR spectra using IR functional group table. 31 32 Example-1 33 34 Determination of functional group in spectrum of n-Butanal 35 The number of ways a molecule can vibrate is related to the number of atoms, and thus the number of bonds, it contains. For even a simple molecule, the number of possible vibrations is large. For example, n-butanal (CH3CH2CH2CHO) has 33 vibrational modes, most differing from each other in energy. Not all of these vibrations produce infrared peaks, but, as shown in previous figure, the spectrum for n-butanal is relatively complex. 36 C3H6O Homework Which of the following is/ are correct according to given IR spectrum above ? ( molecular formula of the compound is C3H6O) I- Double bond equivalence of this spectrum is 1. ıı- The peak around 3050 cm-1 is represent N-H stretching vibrations III- the peak around 1720 cm-1 is represent carbonyl functional group 37