Anabolism and Catabolism PDF
Document Details
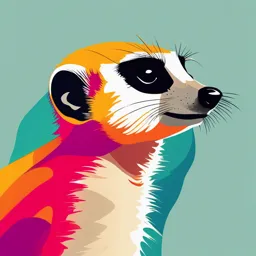
Uploaded by PanoramicCornet
University of Texas at El Paso
Tags
Summary
This document provides an overview of anabolism and catabolism. It discusses cellular respiration, including glycolysis, the citric acid cycle, and oxidative phosphorylation. The document also touches on concepts like phosphorylation, outlining how energy is stored and released.
Full Transcript
Anabolism and Catabolism Anabolism: reactions that build larger molecules or structures from smaller ones; E.g., synthesis of proteins from amino acids Catabolism: reactions that break down more complex structures to simpler ones E.g., hydrolysis of proteins into amino acids Thr...
Anabolism and Catabolism Anabolism: reactions that build larger molecules or structures from smaller ones; E.g., synthesis of proteins from amino acids Catabolism: reactions that break down more complex structures to simpler ones E.g., hydrolysis of proteins into amino acids Three major stages involved in processing energy-containing nutrients: Stage 1: digestion and absorption in gastrointestinal tract Stage 2: in cytoplasm, newly delivered nutrients either: ▪ Built into lipids, proteins, and glycogen by anabolic pathways ▪ Broken down by catabolic pathways to smaller fragments (like pyruvate) Stage 3: in mitochondria, complete breakdown of stage 2 products (most will first be converted into acetyl C o A) ▪ Uses oxygen ▪ Produces carbon dioxide, water, and large amounts of ATP Anabolism and Catabolism Cellular respiration: group of catabolic reactions (glycolysis, the citric acid cycle, and oxidative phosphorylation) that convert some of the chemical energy of nutrients (like glucose) into a form of chemical energy (ATP) cells can use to do work Phosphorylation: transfer of high-energy phosphate group from ATP to another molecule Primes molecule; changing it in a way that increases its activity, produces motion, or does work Body stores energy as glycogen and triglycerides, then breaks them down later to produce ATP for cellular use Three Stages of Metabolism of Energy- Containing Nutrients Figure 24.3 Three stages of metabolism of energy-containing nutrients Oxidation-Reduction Reactions and the Role of Coenzymes Many reactions in cells are oxidation reactions Oxidation is the gain of oxygen or the loss of hydrogen atoms (with their electrons) ▪ Oxidized substance always loses (or nearly loses) electrons as they move to (or toward) a substance that more strongly attracts them ▪ E.g., oxidation of glucose involves removal of pairs of hydrogen atoms (with their electrons) untilremains CO only 2 O2 is final electron acceptor, joining with H atoms to removed HO 2 form Oxidation-Reduction Reactions and the Role of Coenzymes Oxidation-reduction (redox) reactions Whenever one substance loses electrons (oxidized), another gains them (reduced) ▪ Oxidized substances lose and reduced ones gain energy (as energy-rich electrons transferred from one substance to next) Redox reaction enzymes: ▪ Dehydrogenases catalyze removal of hydrogen atoms ▪ Oxidases catalyze transfer of oxygen Most redox enzymes require a B vitamin coenzyme, which can accept the hydrogen and its electron, becoming reduced when a substrate is oxidized ▪ Two important coenzymes of the oxidative pathway: Nicotinamide adenine (NAD ) dinucleotide Flavin adenine dinucleotide (F ▪ E.g.,AFD) AD reduced FADH as succinate is oxidized to 2 to fumarate Succinate to Fumarate ATP Synthesis Two mechanisms capture (as ATP) some of the energy released via cellular respiration Substrate-level phosphorylation Direct transfer of high-energy phosphate group from substrate to ADP Occurs twice in glycolysis (enzymes in cytosol) and once in Krebs cycle (enzymes in mitochondria) Oxidative phosphorylation more complex, but produces most of the ATP Carried out by inner mitochondrial membrane proteins in two steps: ▪ Electron transport ~ 50% of energy released via nutrient chain: oxidation H across inner membrane, creating [H ] gradien used to pump ▪ Chemiosmosis: steep t coupling H (diffusion down gradient) movement of selectively permeable across reaction—the membrane to a chemical synthesis of ATP Diffusion H across inner membrane through protein ATP of provides energysynthase to attach phosphate groups to ADP, making ATP Mechanisms of Phosphorylation Figure 24.4 Mechanisms of phosphorylation 24.4 Carbohydrate Metabolism Is the Central Player in ATP Production Food carbohydrates are converted to glucose, which enters cells via glucose transporters Process enhanced by insulin Glucose enters cell and is immediately phosphorylated to glucose-6-phosphate Most cells lack enzymes to reverse reaction, so glucose trapped in cell ▪ Only cells in intestine, kidney, liver can reverse reaction and release glucose Keeps intracellular glucose (a different molecule from glucose-6-phosphate) concentration low, ensuring continued glucose entry (via facilitated diffusion) Oxidation of Glucose Glucose is catabolized via following reaction: C6H12O6 6O2 6H2O 6CO2 32 ATP heat glucose oxygen water carbon dioxide Complete glucose catabolism requires three pathways, in sequence: 1. Glycolysis 2. Citric acid cycle 3. Oxidative phosphorylation During Cellular Respiration, A TP Is Formed in the Cytosol and in the Mitochondria Figure 24.5 During cellular respiration, A TP is formed in the cytosol and in the mitochondria Oxidation of Glucose Glycolysis (glycolytic pathway) Occurs in cytosol, involves ten chemical steps that convert each glucose to two pyruvate molecules Anaerobic process ▪ Glycolysis itself does not use oxygen and occurs whether or not oxygen is present Three major phases Phase 1: Sugar activation Phase 2: Sugar cleavage Phase 3: Sugar oxidation and ATP formation The Three Major Phases of Glycolysis Figure 24.6 The three major phases of glycolysis Oxidation of Glucose Glycolysis (cont.) Final products of glycolysis (each molecule of C6H12O6 ) : inued glucose is ▪ 2 molecules of (C3H4O3 ) ▪ pyruvate 2 NAD (NADH H ) ▪ reduced Net gain of 2 ATP To continue NAD must remain available to accept glycolysis, atoms more H ▪ When oxygen readily available, this is no NADH H problem, and delivers its H atoms to the electron transport chain ▪ When oxygen insufficient (e.g., during strenuous NADH H exercise), unloads its H atoms back onto pyruvate, reducing it to form lactate Oxidation of Glucose Glycolysis (cont.) inued Some of this lactate enters blood and is picked up by liver, which can convert it back to glucose-6- phosphate ▪ Then converted to glucose and released into blood or stored as glycogen With more oxygen available, more lactate can be oxidized back to pyruvate to enter aerobic pathways (citric acid cycle and oxidative phosphorylation) Glycolysis generates ATP rapidly, but yields only 2 A TP compared to 30–32 ATP when glucose is completely oxidized (via pyruvate entering mitochondria) Oxidation of Glucose Citric acid cycle (Krebs cycle) Next stage of glucose oxidation; named for its first substrate (citrate) Occurs in mitochondrial matrix, fueled mostly by pyruvate (via glycolysis) and fatty acids from fat breakdown Transitional phase converts pyruvate to acetyl CoA via three-step process: 1. Decarboxylation: 1 carbon from pyruvate is removed, CO2 producing gas, which diffuses into blood to be expelled by lungs 2. Oxidation: remaining 2-C fragment oxidized to acetate by removal of H atoms, which are picked up NAD by 3. Formation of acetyl CoA: acetate combines with coenzyme A to form acetyl coenzyme A (acetyl C oA) Acetyl CoA is now ready to enter the citric acid cycle and be broken down by mitochondrial enzymes Simplified Version of the Citric Acid (Krebs) Cycle Figure 24.7 Simplified version of the citric acid (Krebs) cycle Oxidation of Glucose Citric Acid Cycle Makes 2 molecules of CO2 4 molecules of reduced coenzymes 3 NADH and 1 FADH2 1 molecule of ATP (GTP) Oxidation of Glucose Oxidative phosphorylation Electron transport chain (ETC) only pathway to directly use oxygen ▪ Citric acid cycle provides substrates (reduced coenzymes) for ETC, so the coupled pathways are aerobic (require the presence of oxygen to continue) Overview ▪ NADH H and FADH (from glycolysis and Krebs cycle) shuttle H 2 atoms to ETC proteins, which combine O2 to form H2O with ▪ Chemiosmosis uses energy released to Pi to ADP, forming ATP ETC attach involves chain of carrier proteins (with bound metal atoms called cofactors) embedded in inner mitochondrial membrane ▪ Flavins: proteins derived from riboflavin (ETC I and II) ▪ complexes Cytochromes: proteins with iron-containing (III and IV) ▪ pigment Respiratory enzyme complexes: clusters of neighboring carriers that are alternately reduced and oxidized as they pass electrons down the line Focus Figure 24.1 Oxidative Phosphorylati on Focus Figure 24.1 Oxidative Phosphorylation Oxidation of Glucose Oxidative phosphorylation has two phases: Phase 1: ETC creates (H ) gradient across inner mitochondrial proton using high-energy membrane (e ) removed from food ▪ Complexes I and II accept H fuels electrons from NADH FADH2 NAD and FAand D can now return to glycolysis and Krebs cycle ▪ H atoms split protons (H ) electrons (e ) into Electrons passed down chain, releasing energy with each transfer Each complex is reduced e ) and then oxidized (picking up e to next (transferring At complex complex) IV, electron pairs combine with two H and half a molecule of oxygen to form water 2 H 2 e ½ O 2 H2O Energy Is Harvested at Each Step in the Electron Transport Chain Figure 24.8 Energy is harvested at each step in the electron transport chain Oxidation of Glucose Oxidative phosphorylation has two phases: (cont.)inued Phase 2: Chemiosmosis uses energy of proton gradient to synthesize ATP ▪ Proton gradient is both a pH (H higher in gradient intermembrane space than matrix) and a voltage gradient (negative on matrix side of membrane) ▪ As a H strongly attracted to matrix side of membrane, result, but can only cross through membrane protein ATP (complex V) synthase These synthases act as small rotary motors that drive a molecular mill that joins ADP P into ATP (like an ion i and working in pump reverse)