Amino Acid Metabolism PDF
Document Details
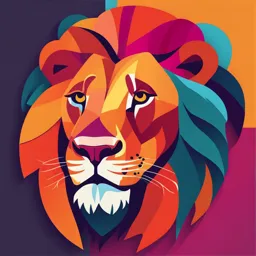
Uploaded by DistinguishedString
KNUST
Prince Adoba
Tags
Related
- BIOCHEMISTRY AMINO ACIDS: METABOLISM OF CARBON SKELETON (Part 2) PDF
- Amino Acids Metabolism PDF
- Harper's Biochemistry Chapter 30 - Conversion of Amino Acids to Specialized Products PDF
- Lippincott's Biochemistry Chapter 19 - Amino Acids (Nitrogen Disposal) PDF
- Lippincott's Biochemistry Chapter 20 - Amino Acids (Degradation and Synthesis) PDF
- MSBS 501 Biochemistry & Cell Biology 25 - Urea Cycle PDF
Summary
This document is a presentation on amino acid metabolism. It covers learning outcomes, different classifications of amino acids, and various reactions involved in amino acid metabolism. It details the process including sources of ammonia, toxicity, and transport.
Full Transcript
AMINO ACID METABOLISM Prince Adoba Department of Medical Diagnostics Medical Laboratory Science KNUST LEARNING OUTCOMES By the end of these series of lectures, you should be able to: Identify the common or standard amino acids;...
AMINO ACID METABOLISM Prince Adoba Department of Medical Diagnostics Medical Laboratory Science KNUST LEARNING OUTCOMES By the end of these series of lectures, you should be able to: Identify the common or standard amino acids; and the amino acid pool Understand the common reactions associated with amino acids Understand sources of ammonia; its toxicity, transport and disposal Describe the synthesis of urea and associated disorders Explain the fate of carbon skeleton of ‘certain’ amino acids and associated disorders – branched chain; aromatic; histidine & sulphur containing amino acids Amino Acid Metabolism Protein are nitrogen-containing The α carbon is linked to; macromolecules consisting of repeating an amino group (NH2), units called α-amino acids. a carboxyl group (COOH), a hydrogen atom (H), and Amino acids are the basic structural a distinctive R group. units of proteins. They are organic compounds R group is often referred to as the containing two functional groups: side chain. carboxyl group and amino groups An α-amino acid consists of a central carbon atom, called the alpha carbon (α carbon). Amino Acid Metabolism There are twenty (20) common amino acids found in proteins – standard amino acids Alanine Serine Valine Threonine Leucine Asparagine Isoleucine Glutamine Methionine Cysteine Proline Lysine Glycine Arginine Tyrosine Histidine Tryptophan Aspartate (Aspartic acid) Phenylalanine Glutamate (Glutamic acid) CLASSIFICATION OF AMINO ACIDS 1. Chemical classification: According to the chemistry or polarity of the side chains (R group) 2. Nutritional classification: A. Essential B. Non-essential 3. Metabolic classification: A. Glucogenic B. Ketogenic or C. Both Chemical Classification The amino acids differ from each other by the R group which varies in structure, size and electrical charge The R group also influences the solubility of the amino acid in water The common amino acids may be classified into FIVE (5) main classes based on the reactivities (polarity) or structure of their R groups as follows: 1. Non-Polar Aliphatic 2. Aromatic 3. Polar, uncharged 4. Polar, positively charged 5. Polar, negatively charged Chemical Classification (A). Non-Polar Aliphatic Glycine (Gly, G) Alanine (Ala, A) Proline (Pro, P) Valine (Val, V)* Leucine (Leu, L)* Isoleucine (Ile, I)* Methionine (Met, M) (B). Aromatic Phenylalanine (Phe, F) Tyrosine (Tyr, Y) Tryptophan (Trp, W) *NB: Branched chain AAs Chemical Classification (C). Polar, Uncharged Serine (Ser, S) Threonine (Thr, T) Cysteine (Cys, C) Asparagine (Asn, N) Glutamine (Gln, Q) Chemical Classification (D). Polar, Positively Charged Lysine (Lys, K) Arginine (Arg, R) Histidine (His, H) (E). Polar, Negatively Charged Aspartate (Asp, D) Glutamate (Glu, E) Nutritional Classification A. Essential Amino Acids: They cannot be synthesized by human body; must be obtained from diet. B. Non-essential Amino Acids: They can be synthesized in the body. Essential Non-essential Arginine* Alanine Valine Aspartate Histidine* Asparagine Essential AAs: Isoleucine Cysteine A.V. HILL, MP., T.T. Leucine Glutamate Lysine Glutamine Methionine Glycine Phenylalanine Proline Threonine Serine Tryptophan Tyrosine Metabolic Classification Amino acids may also be classified, depending on the metabolic intermediates they produce on catabolism (the fate of their carbon skeletons) into: A. Glucogenic ; B. Ketogenic; or C. Both Glucogenic amino acids are catabolised to pyruvate, α-ketoglutarate, succinyl CoA, fumarate or oxaloacetate – can form glucose. Ketogenic amino acids are catabolised to acetyl CoA or acetoacetate – can form fat or ketone bodies. Degradation of Amino Acids to One of Seven Common Metabolic Intermediates Glucogenic degradations in green Ketogenic degradations in red Metabolic Classification Glucogenic Both Glucogenic Ketogenic & Ketogenic Alanine Arginine Phenylalanine Leucine Asparagine Aspartate Isoleucine Lysine Cysteine Glutamate Tryptophan Glutamine Glycine Tyrosine Histidine Methionine Threonine Proline Serine Valine i.e. PITTT Uncommon Amino Acids Aside the 20 common AAs, quite a number of unusual amino acids are found in minor amounts in proteins and non-protein compounds. These unusual amino acids found in proteins result from modifications of the common amino acids – derived amino acids. In a few cases, these amino acids are found in polypeptide chains. E.g. 4-hydroxyproline & 5-hydroxylysine (collagen); 6-N-methyllysine (myosin) Uncommon Amino Acids Non-protein Amino Acids: There are some AAs found in the cells but not in proteins. E.g. Ornithine and citrulline (intermediates in the biosynthesis of arginine and in the urea cycle) OVERVIEW OF AMINO ACID POOL Bodyprotein 10-12-kg in adult Protein breakdown Protein synthesis (300-400 g/day) (300-400 g/day) Synthesis of non-protein Cpds(30 g/day;creatine, Dietary protein Porphyrins, phospholipids, (40-100 g/day) Body Amino acid purines, pyrimidines etc) Pool (100 g) Carbohydrates, fat (when excess dietaryprotein) Synthesis of non-essential Amino acids (variable) Protein loss from Energy (10-15% of Body (30-50 g/day) Body’s daily requirement) Sources Mostly asurea Utilization Urine OVERVIEW OF AMINO ACID METABOLISM Non-essential amino acids GENERAL REACTIONS OF AMINO ACIDS Amino acids can undergo reactions such as: 1. Decarboxylation reaction 2. Transamination reaction 3. Deamination reaction: a. Oxidative b. Non-oxidative GENERAL REACTIONS OF AMINO ACIDS: 1. Decarboxylation Reaction: Amino acids are decarboxylated to form amines. Some of the amines formed have very important physiological roles A. Histidine L- Histidine → Histamine + CO2 Histidine Histidine decarboxylase requires pyridoxal decarboxylase phosphate as a coenzyme Histamine: stimulates gastric secretion of acid, neurotransmitter, etc GENERAL REACTIONS OF AMINO ACIDS: 1. Decarboxylation Reactions (cont.) B. Dopamine Dopa → Dopamine + CO2 Dopa is an intermediate in the formation of adrenaline (epinephrine): hormone, neurotransmitter, etc (vasoconstriction) NB: BH4 - tetrahydrobiopterin GENERAL REACTIONS OF AMINO ACIDS: 1. Decarboxylation Reaction (cont.) C. Serotonin 5-hydroxyltryptophan → 5-hydroxytryptanine + CO2 Serotonin (5-hydroxytryptamine) is a vasoconstrictor, neurotransmitter, etc. D. Gamma aminobutyric acid (GABA) Glutamate → γ-aminobutyric acid + CO2 GABA is a neurotransmitter GENERAL REACTIONS OF AMINO ACIDS: 2. Transamination Reaction Transamination is the transfer of an amino (-NH2) group from an amino acid to an α- keto acid to form the corresponding amino acid. Thus, the α-keto acid that gains the amino group becomes an amino acid, and the amino acid that loses its amino group becomes an α-keto acid. The reaction is catalyzed by amino transferases which require pyridoxal phosphate, a derivative of vitamin B6 as a prosthetic group. GENERAL REACTIONS OF AMINO ACIDS: 2. Transamination Reaction (cont.) Pyridoxal phosphate functions as the intermediate carrier of amino groups at the active site of the aminotransferase. PLP undergoes reversible transformation between the aldehyde form and the aminated form, pyridoxamine phosphate. Transamination reactions are classic examples of bimolecular Ping-Pong reactions (proposed by Snell and Braustein). 2. Transamination Reaction (cont.) Examples of aminotransferases are AST and ALT. In mammals, all α-amino acids except lysine and threonine can be transaminated Proline, which has a secondary amino group (imino group – NH), cannot participate in PLP dependent reactions including transamination and decarboxylation. GENERAL REACTIONS OF AMINO ACIDS: 3. Deamination Deamination is the removal of amino group from the amino acids as NH3 (for urea synthesis) The carbon skeleton is simultaneously converted to keto acids Deamination could be either: A. Oxidative Deamination B. Non-Oxidative Deamination GENERAL REACTIONS OF AMINO ACIDS: A. Oxidative Deamination Oxidative deamination: liberation of free ammonia from the amino group of AAs coupled with oxidation (mostly in liver & kidney) Purpose: To provide ammonia (for urea synthesis) & keto acids (for various reactions) The oxidative deamination of glutamate (which serves as a ‘collection centre’ for amino groups in the biological systems) occurs in the mitochondria of the liver yielding ammonium ions L-Glutamate + H2O + NAD(P)+ α-KG + NAD(P)H + NH4+ GENERAL REACTIONS OF AMINO ACIDS: A. Oxidative Deamination (cont.) This reaction (reversible) is catalyzed by glutamate dehydrogenase (GDH) (present in the mitochondria) which is a complex allosteric enzyme; can use both NAD+ or NADP+ as co-enzyme. Its allosteric activators are GDP and ADP and the allosteric inhibitors are GTP and ATP. Thus, whenever the hepatocytes require fuel/energy from the TCA cycle, glutamate dehydrogenase activity increases to produce α-ketoglutarate to feed TCA cycle. On the other hand when ATP and GTP accumulate, oxidative deamination of glutamate is inhibited. GENERAL REACTIONS OF AMINO ACIDS: A. Oxidative Deamination (cont.) Important reversible reaction: it links up glutamate metabolism with TCA cycle through α-ketoglutarate NB: GDH involves in both anabolic and catabolic reactions The combined action of the aminotransferases and glutamate dehydrogenase is referred to as transdeamination The coupling of oxidative deamination of L-glutamate with transamination creates an avenue for deaminating almost all amino acids GENERAL REACTIONS OF AMINO ACIDS: A. Oxidative Deamination (cont.) Other Oxidative Deamination Reaction: Oxidative deamination by amino acid oxidases producing NH3 and keto acids L-amino acid oxidase (less common; FMN) D-amino acid oxidase (common in liver and kidney; FAD): Helps to convert unnatural D-amino acids (present in plants & microbes) to L- amino acids GENERAL REACTIONS OF AMINO ACIDS: B. Non-Oxidative Deamination Some amino acids can be deaminated to liberate NH3 without undergoing oxidation. Examples: a. Amino acid dehydrases Serine, threonine → Respective keto acids + NH3 b. Amino acid desulfhydrases Cysteine → Pyruvate + NH3 + H2S c. Histidase Histidine → Urocanate + NH3 Sources of Ammonia Production of NH3 occurs from: – Amino acids – Biogenic amines – Amino group of purines and pyrimidines – Action of intestinal bacteria (urease) on urea Functions of Ammonia It is involved in the synthesis of many substances e.g. Non-essential AAs, purines, pyrimidines, amino sugars etc. Ammonium ion is important in the maintenance of acid-base balance Ammonia Toxicity Ammonia (marginal elevation – Acquired or Genetic) is toxic to mammalian cells esp. the CNS (nerve cells) for the following reasons: 1. Accumulated ammonia reverses the glutamate dehydrogenase reaction thus depleting the cell (neuron) of α-ketoglutarate (TCA cycle) – This will lead to depressed synthesis of ATP which will thus affect active transport systems – Impaired active transport will lead to loss of electrochemical gradient which is paramount to the transfer of impulses. Ammonia Toxicity (cont.) 2. It results in the increased synthesis of glutamine (an osmotically active solute in brain astrocytes causing swelling) which may lead to encephalopathy (cerebral edema). 4. It may also result in acid-base disturbances. Transport of Ammonia Ammonia (at physiological pH exists as ammonium – NH4+ ion) is quite toxic to mammalian tissue especially brain. Despite constant production, NH3 concentration in circulation is low – efficient NH3 transport mechanism & immediate utilization for urea synthesis. In peripheral tissues, excess ammonia is converted to non-toxic compounds (glutamine & alanine) before transport to the liver and kidney. In many peripheral tissues (e.g. brain), ammonia is converted to glutamine (storehouse of NH3) in a reaction catalyzed by glutamine synthetase (mitochondrial enzyme). Transport of Ammonia (cont.) Glutamine is then transported in the blood to the liver where it is converted to glutamate by glutaminase in the mitochondria releasing NH3. Alanine-glucose Cycle Ammonia Disposal- (Nitrogen Excretion) Based on mode of disposal of ammonia, animals may be classified into three: A. Ammonotelic: Amino nitrogen may be excreted as ammonia in most aquatic species such as the bony fish. B. Uricotelic: It may also be excreted as uric acid by birds and reptiles. C. Ureotelic: The mammals including man excrete ammonia as urea. UREA SYNTHESIS (UREA CYCLE) Urea (synthesized from ammonia) is Urea has two amino (-NH2) groups, one the end product of AA metabolism. derived from NH3 and the other from aspartate; & the carbon atom is The main site of ureogenesis is the supplied by CO2 liver. The synthesis of urea/urea cycle consists of five enzymatic steps; elucidated by Hans Krebs and Kurt Henseleit (1932), hence it is also known as Krebs- Henseleit cycle The first two enzymes are found in the mitochondria; the rest are localized in Urea the cytosol Liver cell or hepatocyte UreaCycle CARBAMOYL PHOSPHATE SYNTHETASE 1 Mitochondrion Cytosol ARGININOSUCCINICACID SYNTHETASE UREA SYNTHESIS (UREA CYCLE) Step 1: Synthesis of carbamoyl phosphate Carbamoyl phosphate is synthesized from condensation of NH4+ with CO2 by carbamoyl phosphate synthetase I (CPS1) This irreversible reaction occurs in the mitochondria and consumes two ATP (the rate-limiting step) CPS1 requires allosteric activator, N-acetyl glutamate N-acetyl glutamate (NAG) is synthesized from acetyl CoA and glutamate by NAG synthase when protein intake is high. NAG synthase activity is also activated by [arginine] UREA SYNTHESIS (UREA CYCLE) Step II: Formation of citrulline Carbamoyl phosphate reacts with L-ornithine to form L-citrulline in a reaction catalysed by ornithine transcarbamoylase (OTC). This reaction occurs in the mitochondria. L-citrulline is transported to cytosol by a transporter system. UREA SYNTHESIS (UREA CYCLE) Step III: Synthesis of argininosuccinate Citrulline then condenses with aspartate to form argininosuccinate in the cytosol This reaction is catalysed by argininosuccinate synthetase and consumes one ATP: the reaction is driven forward by the pyrophosphate cleavage The second amino group of urea is incorporated in this reaction from aspartate UREA SYNTHESIS (UREA CYCLE) Step IV: Cleavage of argininosuccinate Step V: Formation of urea (Cleavage of Arginine) Argininosuccinate is then cleaved into fumarate and arginine by Arginine is then cleaved by arginase argininosuccinate lyase (mostly found only in the liver) to (arginonosuccinase). yield urea and ornithine. Arginine is the immediate precursor Urea freely diffuses from the liver into for urea; and the fumarate liberated blood, then to kidneys for excretion in joins TCA cycle. urine; some also get into the GIT. Ornithine regenerated enters the mitochondria for reuse. UREA SYNTHESIS (UREA CYCLE) Overall reaction and energetics: The cycle is irreversible and consumes 4 ATP NH4+ + CO2 + Aspartate + 3ATP → Urea + Fumarate + 2ADP+ 2Pi + AMP + PPi LINK BETWEEN THE ORNITHINE/UREA AND CITRIC ACID CYCLES The two cycles are linked in three different ways: 1. Fumarate produced in the argininosuccinate lyase reaction is transported into the mitochondria. In the mitochondria, fumarate is ultimately converted into oxaloacetate by fumarase and malate dehydrogenase. Oxaloacetate has the following metabolic fates: – It may condensewith acetyl CoA to form citrate and become committed into the TCA cycle. – It may be converted to glucose (via gluconeogensis). – It may also be converted to aspartate (via transamination) which is an important nitrogen donor in the urea cycle. LINK BETWEEN THE ORNITHINE/UREA AND TCA CYCLES 2. TCA cycle liberates CO2 in the mitochondria; the liberated CO2 can be utilized in urea cycle. 3. ATP (12) are generated in the TCA cycle while ATP (4) are utilized for urea synthesis. INHERITED ENZYMATIC DEFECTS OF THE UREA CYCLE Defect in any one of the five enzymes involved in urea synthesis finally lead to hyperammonaemia As such can result in similar clinical signs and symptoms. The symptoms of hyperammonaemia include lethargy, stupor, convulsions and CNS impairment. In severe cases, coma and death may result. INHERITED ENZYMATIC DEFECTS OF THE UREA CYCLE Infants born with a complete deficiency of urea synthesizing enzymes die soon after their first protein meal. Those with partial deficiency of the enzymes may however survive to early adulthood or later if managed properly. Patients with inherited defects of urea synthesizing enzymes cannot tolerate protein-rich diets. INHERITED ENZYMATIC DEFECTS OF THE UREA CYCLE 1. Hyperammonemia Type 1: A consequence of carbamoyl phosphate synthase I deficiency. 2. Hyperammonemia Type 2: A deficiency of ornithine transcarbamoylase produces this X chromosome–linked deficiency. 3. Citrullinuria/Citrullinemia: Deficiency of argininosuccinate synthetase - Plasma and cerebrospinal fluid citrulline levels are elevated and 1–2 g of citrulline are excreted daily. 4. Argininosuccinic aciduria/acidemia: Deficiency of argininosuccinase - Characterized by elevated levels of argininosuccinate in blood, cerebrospinal fluid, and urine. 5. Hyperargininemia: Deficiency of arginase - Characterized by elevated blood and cerebrospinal fluid arginine levels. Defects of Urea Cycle ↑ orotic acid V III IV INHERITED ENZYMATIC DEFECTS OF THE UREA CYCLE (Cont.) The first approach to treatment of all of the inborn errors of urea synthesis is a relatively low protein intake (adequate for growth but avoiding a significant excess of amino acids to be deaminated after a meal). Avoidance of prolonged fasting. They may be provided with diets containing the α-keto analogues of the essential amino acids. THE END