Alkanes - Organic Chemistry
Document Details
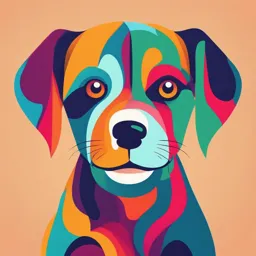
Uploaded by EuphoricAlliteration
Tags
Summary
This document provides an overview of alkanes, a fundamental class of organic compounds. It covers basic definitions, structural variations, naming conventions, and reactions. The study of alkanes is critical to understanding organic chemistry.
Full Transcript
Alkanes Alkanes are simplest family of molecules that contain the carbon– carbon single bond results from σ (head-on) overlap of carbon sp 3 hybrid orbitals. Alkanes are often described as saturated hydrocarbons: hydrocarbons because they contain only carbon and hydrogen; saturated because the...
Alkanes Alkanes are simplest family of molecules that contain the carbon– carbon single bond results from σ (head-on) overlap of carbon sp 3 hybrid orbitals. Alkanes are often described as saturated hydrocarbons: hydrocarbons because they contain only carbon and hydrogen; saturated because they have only C -C and C-H single bonds and thus contain the maximum possible number of hydrogens per carbon. They have the general formula CnH2n+2, where n is an integer. Alkanes are also occasionally called aliphatic compounds. 2 Think about the ways that carbon and hydrogen might combine to make alkanes. With one carbon and four hydrogens, only one structure is possible: methane, CH4. Similarly, there is only one combination of two carbons with six hydrogens (ethane, CH3CH3) and only one combination of three carbons with eight hydrogens (propane, CH3CH2CH3). When larger numbers of carbons and hydrogens combine, however, more than one structure is possible. For example, there are two substances with the formula C 4H10: the four carbons can all be in a row (butane), or they can branch (isobutane). Similarly, there are three C5H12 molecules, and so on for larger alkanes. Compounds like butane and pentane, whose carbons are all connected in a row, are called straight-chain alkanes, or normal alkanes. Compounds like 2-methylpropane (isobutane), 2-methylbutane, and 2,2-dimethylpropane, whose carbon chains branch, are called branched-chain alkanes. Compounds like the two C4H10 molecules and the three C 5 H 12 molecules, which have the same formula but different structures, are called isomers, from the Greek isos + meros, meaning "made of the same parts." Isomers are compounds that have the same numbers and kinds of atoms but differ in the way the atoms are arranged. Compounds like butane and isobutane, whose atoms are connected differently, are called constitutional isomers. As Table 3-2 shows, the number of possible alkane isomers increases dramatically with the number of carbon atom. Constitutional isomerism is not limited to alkanes it occurs widely throughout organic chemistry. Constitutional isomers may have different carbon skeletons (as in isobutane and butane), different functional groups (as in ethanol and dimethyl ether), or different locations of a functional group along the chain (as in isopropylamine and propylamine). Regardless of the reason for the isomerism, constitutional isomers are always different compounds with different properties but with the same formula. A given alkane can be drawn in many ways. For example, the straight chain, four-carbon alkane called butane can be represented by any of the structures shown in Figure 1. These structures don’t imply any particular three- dimensional geometry for butane; they indicate only the connections among atoms. In practice, chemists rarely draw all the bonds in a molecule and usually refer to butane by the condensed structure, CH3CH2CH2CH3 or CH3(CH2)2CH3. Still more simply, butane can be represented as n-C 4 H10, where n denotes normal (straight-chain) butane. Figure 1: Some representations of butane, C 4 H 10. The molecule is the same regardless of how it’s drawn. These structures imply only that butane has a continuous chain of four carbon atoms; they do not imply any specific geometry. Straight-chain alkanes are named according to the number of carbon atoms they contain, as shown in Table 3-3. With the exception of the first four compounds (methane, ethane, propane, and butane) whose names have historical roots, the alkanes are named based on Greek numbers. The suffix -ane is added to the end of each name to indicate that the molecule identified is an alkane. Thus, pentane is the five-carbon alkane; hexane is the six carbon alkane, and so on. We’ll soon see that these alkane names form the basis for naming all other organic compounds, so at least the first ten should be memorized. 10 Alkyl Groups If you imagine removing a hydrogen atom from an alkane, the partial structure that remains is called an alkyl group. Alkyl groups are not stable compounds themselves; they are simply parts of larger compounds. Alkyl groups are named by replacing the -ane ending of the parent alkane with an -yl ending. For example, removal of hydrogen from methane, CH4 , generates a methyl group, -CH3, and removal of hydrogen from ethane, CH3CH3, generates an ethyl group, -CH2CH3. Similarly, removal of a hydrogen atom from the end carbon of any straight-chain alkane gives the series of straight- chain alkyl groups shown in Table 3-4. Combining an alkyl group with any of the functional groups listed earlier makes it possible to generate and name many thousands of compounds. For example: Just as straight-chain alkyl groups are generated by removing hydrogen from an end carbon, branched alkyl groups are generated by removing a hydrogen atom from an internal carbon. Two 3-carbon alkyl groups and four 4-carbon alkyl groups are possible (Figure 3-3). Figure 3-3: Alkyl groups generated from straight-chain alkanes. One further comment about naming alkyl groups: the prefixes sec- (for secondary) and tert- (for tertiary) used for the C4 alkyl groups in Figure 3-3 refer to the number of other carbon atoms attached to the branching carbon atom. There are four possibilities: primary (1°), secondary (2°), tertiary (3°), and quaternary (4°). The symbol R is used here and throughout organic chemistry to represent a generalized organic group. The R group can be methyl, ethyl, propyl, or any of a multitude of others. You might think of R as representing the Rest of the molecule, which isn’t specified. The terms primary, secondary, tertiary, and quaternary are routinely used in organic chemistry, and their meanings need to become second nature. For example, if we were to say , "Citric acid is a tertiary alcohol," we would mean that it has an alcohol functional group (-OH) bonded to a carbon atom that is itself bonded to three other carbons. In addition, we also speak of hydrogen atoms as being primary, secondary, or tertiary. Primary hydrogen atoms are attached to primary carbons (RCH3), secondary hydrogens are attached to secondary carbons (R 2 CH 2 ), and tertiary hydrogens are attached to tertiary carbons (R3CH). There is, of course, no such thing as quaternary hydrogen. Naming Alkanes A chemical name typically has four parts in the IUPAC system of nomenclature: prefix, parent, locant, and suffix. The prefix identifies the various substituent groups in the molecule, the parent selects a main part of the molecule and tells how many carbon atoms are in that part, the locants give the positions of the functional groups and substituents, and the suffix identifies the primary functional group. Step 1: Find the parent hydrocarbon. (a) Find the longest continuous chain of carbon atoms in the molecule, and use the name of that chain as the parent name. The longest chain may not always be apparent from the manner of writing; you may have to ”urn corners.“ (b) If two different chains of equal length are present, choose the one with the larger number of branch points as the parent. Step 2: Number the atoms in the longest chain. (a) Beginning at the end nearer the first branch point, number each carbon atom in the parent chain. The first branch occurs at C3 in the proper system of numbering, not at C4. (b) If there is branching an equal distance away from both ends of the parent chain, begin numbering at the end nearer the second branch point. Step 3: Identify and number the substituents. (a) Assign a number, or locant, to each substituent to locate its point of attachment to the parent chain. (b) If there are two substituents on the same carbon, give both the same number. There must be as many numbers in the name as there are substituents. 4-ethyl-2,4-dimethylhexane Step 4: Write the name as a single word. Use hyphens to separate the different prefixes, and use commas to separate numbers. If two or more different substituents are present, cite them in alphabetical order. If two or more identical substituents are present on the parent chain, use one of the multiplier prefixes di-, tri-, tetra-, and so forth, but don’t use these prefixes for alphabetizing. Full names for some of the examples we have been using are as follows: Step 5: Name a branched substituent as though it were itself a compound. In some particularly complex cases, a fifth step is necessary. It occasionally happens that a substituent on the main chain is itself branched. In the following case, for instance, the substituent at C6 is a three-carbon chain with a methyl group. To name the compound fully, the branched substituent must first be named. 6-isobutyl-2,3-dimethyldecane Number the branched substituent beginning at the point of its attachment to the main chain, and identify it—in this case, a 2- methylpropyl group. The substituent is treated as a whole and is alphabetized according to the first letter of its complete name, including any numerical prefix. It is set off in parentheses when naming the entire molecule. As a further example: For historical reasons, some of the simpler branched- chain alkyl groups also have nonsystematic, common names, as noted earlier. The common names of these simple alkyl groups are so well entrenched in the chemical literature that IUPAC rules make allowance for them. Thus, the following compound is properly named either 4-(1-methylethyl) heptane or 4- isopropylheptane. There’s no choice but to memorize these common names; fortunately, there are only a few of them. When writing an alkane name, the non-hyphenated prefix iso- is considered part of the alkyl-group name for alphabetizing purposes, but the hyphenated and italicized prefixes sec- and tert - are not. Thus, isopropyl and isobutyl are listed alphabetically under i, but sec-butyl and tert-butyl are listed Physical Properties of Alkanes Alkanes are sometimes referred to as paraffins, a word derived from the Latin parum affinis, meaning “little affinity.” This term aptly describes their behavior, for alkanes show little chemical affinity for other substances and are chemically inert to most laboratory reagents. They are also relatively inert biologically and are not often involved in the chemistry of living organisms. Alkanes are used primarily as fuels, solvents, and lubricants. Natural gas, gasoline, kerosene, heating oil, lubricating oil, and paraffin wax are all composed primarily of alkanes, with different physical properties resulting from different ranges of molecular weights. 1. Solubilities and Densities of Alkanes: Alkanes are nonpolar, so they dissolve in nonpolar or weakly polar organic solvents. Alkanes are said to be hydrophobic (water hating) because they do not dissolve in water. Alkanes are good lubricants and preservatives for metals because they keep water from reaching the metal surface and causing corrosion. Alkanes have densities around 0.7 g/mL, compared with a density of 1.0 g/mL for water. Because alkanes are less dense than water and insoluble in water, a mixture of an alkane (such as gasoline or oil) and water quickly separates into two phases, with the alkane on top. 2. Boiling point and melting point Alkanes show regular increases in both boiling point and melting point as molecular weight increases (Figure 3-4), an effect due to the presence of weak dispersion forces between molecules (Section 2 -12). Only when sufficient energy is applied to overcome these forces does the solid melt or liquid boil. As you might expect, dispersion forces increase as molecule size increases, accounting for the higher melting and boiling points of larger alkanes. Figure 3-4 A plot of melting and boiling points versus number of carbon atoms for the C1–C14 straight-chain alkanes. There is a regular increase with molecular size. Another effect seen in alkanes is that increased branching lowers an alkane’s boiling point. Thus, pentane has no branches and boils at 36.1 °C, isopentane (2- methylbutane) has one branch and boils at 27.85 °C, and neopentane (2,2-dimethylpropane) has two branches and boils at 9.5 °C. Similarly, octane boils at 125.7 °C, whereas isooctane (2,2,4 trimethylpentane) boils at 99.3 °C. Branched-chain alkanes are lower boiling because they are more nearly spherical than straight-chain alkanes, have smaller surface areas, and consequently have smaller dispersion forces. 35 Reactions of Alkanes Alkanes do, however, react with oxygen, halogens, and a few other substances under appropriate conditions. Reaction with oxygen occurs during combustion in an engine or furnace when an alkane is used as a fuel. Carbon dioxide and water are formed as products, and a large amount of heat is released. For example, methane (natural gas) reacts with oxygen according to the equation: 1. Combustion: is a rapid oxidation that takes place at high temperatures, converting alkanes to carbon dioxide and water. Little control over the reaction is possible, except for moderating the temperature and controlling the fuel/air ratio to achieve efficient burning. 2. Cracking and Hydrocracking catalytic cracking of large hydrocarbons at high temperatures produces smaller hydrocarbons. The cracking process usually operates under conditions that give the maximum yields of gasoline. In hydrocracking, hydrogen is added to give saturated hydrocarbons; cracking without hydrogen gives mixtures of alkanes and alkenes. 3. Halogenation Alkanes can react with halogens (F2, Cl2, Br2, I2) to form alkyl halides. For example, methane reacts with chlorine to form chloromethane (methyl chloride), dichloromethane (methylene chloride), trichloromethane (chloroform), and tetrachloromethane (carbon tetrachloride). The reaction of an alkane with Cl 2 occurs when a mixture of the two is irradiated with ultraviolet light (denoted hy, where y is the Greek letter nu). Depending on the time allowed and the relative amounts of the two reactants, a sequential substitution of the alkane hydrogen atoms by chlorine occurs, leading to a mixture of chlorinated products. Methane, for instance, reacts with Cl2 to yield a mixture of CH3Cl, CH2Cl2, CHCl3, and CCl4. A radical is highly reactive because it contains an atom with an odd number of electrons (usually seven) in its valence shell, rather than a stable, noble gas octet. A radical can achieve a valence- shell octet in several ways. For example, the radical might abstract an atom and one bonding electron from another reactant, leaving behind a new radical. The net result is a radical substitution reaction. An example of an industrially useful radical reaction is the chlorination of methane to yield chloromethane. This substitution reaction is the first step in the preparation of the solvents dichloromethane (CH2Cl2) and chloroform (CHCl3). Like many radical reactions in the laboratory, methane chlorination requires three kinds of steps: initiation, propagation, and termination. Initiation Irradiation with ultraviolet light begins the reaction by breaking the relatively weak Cl-Cl bond of a small number of Cl2 molecules to give a few reactive chlorine radicals. Propagation Once produced, a reactive chlorine radical collides with a methane molecule in a propagation step, abstracting a hydrogen atom to give HCl and a methyl radical (·CH3). This methyl radical reacts further with Cl2 in a second propagation step to give the product chloromethane plus a new chlorine radical, which cycles back and repeats the first propagation step. Thus, once the sequence has been initiated, it becomes a self- sustaining cycle of repeating steps (a) and (b), making the overall process a chain reaction. Termination Occasionally, two radicals might collide and combine to form a stable product. When that happens, the reaction cycle is broken and the chain is ended. Such termination steps occur infrequently, however, because the concentration of radicals in the reaction at any given moment is very small. Thus, the likelihood that two radicals will collide is also small. References: Text Books of John McMurry "Organic Chemistry" 9th Edition Cengage Learning, USA