Agr345 Chapter 9: Soil Fertility PDF
Document Details
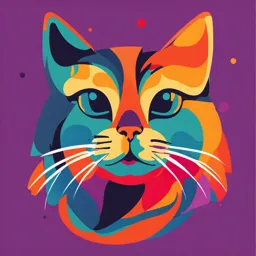
Uploaded by DetachableFeministArt6054
Murray State University
Tags
Summary
This document discusses soil fertility, focusing on efficient nutrient management practices for sustainable crop production.
Full Transcript
SoilFertility Chapter 9 Improving agricultural production per unit of farm area to fulfill future food and fiber demand causes an increase in soil nu...
SoilFertility Chapter 9 Improving agricultural production per unit of farm area to fulfill future food and fiber demand causes an increase in soil nutrient removal by plants, thus resulting in decreasing soil fertility. Consequently, eficient nutrient management practices an improvement estimation of plant-available nutrients are vital that involve for sustainable crop production. With over 50% of soils degraded with some regions approaching 70%, anthropogenic land degradation threatens the abilityof soil to provide important nutrients for crop growthand yield. Land degradation effects include both onsite (e.g., erosion) and offsite (sediment deposition) degrada tion. Onsite land degradation effects on agricultural productivity include physical (erusting. compaction, ero sion, desertification),chemical (acidification,leaching, salinization, fertility depletion), and biological (carbon oxidation/loss, microbial biodiversity) processes. Offsite effects are related to the eutrophication of surface water, groundwater contamination, and trace gas emissions (CO,, CH,, N,0, NO)to the atmosphere. Many of the same biological,chemical, and physical soil properties affected by onsite soil degrading processes also impact soil fertility and nutrient availability to plants. Understanding these processes and interactions is essential to optimizing plant nutrient availabilityand minimizing nutrient losses to the environment. As plants are removed from a field or soil sediments are transported offsite, nutrients in the soil are depleted. Native soil nutrient supply depends on the soil's ability to buffer nutrient loss through crop removal. Therefore, to optimize nutrient supply to crops and minimize the environmental risk of nutrient use, it is essential to understand nutrient reactions and processes in soils (soil fertility) and to efficiently manage inor ganic and organic nutrient inputs (nutrient management)to ensure adequate soil nutrient supply. Agricultural producers must take advantage of soil- and plant-management technologies that increase plant productivity and minimize soilproductivity loss through runoff, leaching, and nutrient depletion. In addition, with increas ing demand for fertilizer (>food demand), increasing the crop recovery of applied nutrients while reducing nutrient depletion due to offsitetransport is important. The purpose of this chapter is to explore the principles of soil fertility, how soil provides soil nutrients, how plant roots obtain soil nutrients, and how to improve and manage soil fertility levels to improve crop production. A.What is Soil Fertility? Soil fertility refers to the ability of soil to hold plant nutrients and make them available for plant growth. There are two types of soil fertility-native fertility and managed fertility. Native fertility is the natural capacity of soil to hold and supply nutrients because of its parent material and organic matter content. Managed fertility refers to plant nutrients and other amendments that are added to soil to make it fertile. 188 Chapter9 Soil Fertility Fertility is not similar to productivity. A fertile soil may not be productivedue to lack of water or proper crop management. An unfertile soil may be very fertileifirrigated and supplied with a timelyamount of plant nutrients. B. Plant Nutrients Throughout Earth's history, the natural cycling of nutrients has occurred from the soil to plants and ani mals,and then back to the soil, primarily through decompositionof biomass.This cycling helps to maintain the essential nutrients required for plant growth in the soil. Complex nutrient cycles incorporatea range of physical, chemical, andmost importantly-biologicalprocesses to trace the fate of specific plant nutri ents (e.g., N, P, C, S) in theenvironment. For a thorough analysis of these cycles, additional reference mate rials are available. A simplified version of nutrient cycling in natural and agricultural systems is described in Figure 9.1. Figure 9.1 https:/www.nature.com/scitablelcontent/ne0000/ne0000/ne0000/ne0000/84224407/ figure1_FINAL 1_2.jpg The thickness of the arrows correspondsto the relative amounts. The blue arrows represent basic nutrient pathways such as plant uptake, biomass decomposition,and nutrient return to soil. In the agroecosystem fer tilizer (e.g., manure, compost, chemical) is applied and nutrients are removed through plant harvest (red arrows).Greater potential leaching (eg, nitrate), erosion (e.g,soil, phosphate), and CO, emissions in the agroccosystemare indicated via thick black arrows. Out of the 15 essential elementsthat come from the soil, we deal with only the 12 that aregeneraly man aged by the growers.These 12 elementsare "mineral nutrients" and are obtained from the soil. We further divide mineral nutrients into three groups:primary,intermediate, and micronutrients. Our presentation will excludecobalt, chlorine, and nickel from our discussion on the management of essential mineral nutrients, though are included by many as essential nutrients. The primary are nitrogen, phosphorus, and potassium.You may be most familiar with these nutrients three nutrients because they are required in larger quantities than other nutrients. These three elements form the basis of the N-P-K label on commercial fertilizer bags. As a result, the management of these nutrients is very important.However, the primary nutrients areno more importantthan the other essential elements since all essential elements are required for plant growth. Remember that the "Law of the Minimum" tells us that if deficient, any essential nutrient can become the controlling force in crop yield. The intermediate are sulfur, magnesium, and calcium. Together, primary and intermediate nutri nutrients ents are referred to as macronutrients. Macronutrients are expressed asa certain percentage (%)of the total plant uptake. Although sulfur, magnesium, and calcium are called intermediate, these elementsare not necessarily needed by plants in smallerquantities. In fact, phosphorus is required in the same amount as the intermediate nutrients, despite being a primary nutrient. Phosphorus is referred to as a primary nutrient because of the high frequencyof soils that are deficient in this nutrient, rather thanthe amount of phosphorus that plants actually use for growth. The remaining essential elements are the micronutrients and are required in very smallquantities. In com with parison macronutrients, the uptakeof mieronutrients is expressed in parts per million (ppm,where 10,000 ppm = 1.0%), rather than on a percentagebasis. Again, this does not infer that micronutrients are of lesser importance.Ifany micronutrient is deficient, thegrowth of the entire plant will not reach maxi mum yield (Law of the Minimum). Since the soil provides the most essential nutrients, it is crucial to understand the soil processes that determine the availability of cach essential nutrient for plant uptake. Chapter 9 Soil Fertility 189 C. The Essential Nutrients for Plants There are at least sixteen essential nutrients for plants, and there are additional nutrients, such as chromium (Cr)requiredforhuman nutrition.There arethreegroups under these nutrients:basic nutrients, macronutrients, and micronutrients. It is crucial to notice that just because plants take up a nutrient doesn't mean that they require it. Someplants in the genus Astragalus (loco weeds) accumulate arsenic (As),which causes them to be toxic to animals. Indian mustard (Brassicajuncea) takes up various heavy metals such as lead (Pb) and mercury (Hg), but these metals play no role in growth (although they do make Indian mustard useful in phytoremediation). Tobacco (Nicotinia tabacum) accumulates approximately 1.5 um/g cadmium (Cd), which contributes to tobacco's potential to cause increased lung cancer rates in smokers. Macronutrients Macronutrients (Table 9.1) are those nutrients required in the greatest amount by plants. The basic nutrients carbon (C),hydrogen (H), and oxygen (0)are the building blocks of plant structure in compounds such as carbohydrates (starch, cellulose), hydrocarbons (fattyacids), and lignin. Because these elements come from water and carbon dioxide (CO),so there is limited concern about their availability in soil.Water isa structural requirement (it a strict biochemical sense, it is also an important substrate for provides plant cell turgor). In metabolic reactions. But if a plant has enough water to grow, it has enough water for its nutritional and meta bolic needs. About 909% of plant dry weight is made up of these basic elements. The other plant nutrients are so-named because they are needed in the greatest amounts by growing plants. Nitrogen (N), K,Ca, P, Mg. and S all have important roles in structural compounds such as protein, lipids,or nucleic acids, or they play an important role in plant metabolic compounds involved in enzymatic activity and energy transfer (Table 9.2). Micronutrients All of the beneficial nutrients listed in Table 9.l are micronutrients with the exception of Si and Na. Silicon may be required by some plants as an essential part of the plant structure. In some plants, it is taken up and deposited naturally, which makes the stems feel gritty.Diatoms, single-celled algae in soil and aquatic sys tems, absolutely require Si because Si makes up part of their cell wall. Diatomaceous carth is almost entirely Table 9.1 Essential nutrients. Source:Adapted from Coyne & Thompson, 2006. composed of these Si cell walls. Sodium is important in halophilic (salt-loving) plants. Sodium may play an essential role in survival in salt-stressed environments. Sometimes the beneficial nutrient is only an absolute requirement for plants undertaking certain func tions.For example, nitrogen-fixing plants can grow perfectly well without nitrogen fixation as long as they are supplicd by inorganic N. However, when these plants need to fix N, they have to have trace quantities of the micronutrient cobalt (Co).Cobalt isan essential metal cofactor forthe enzymecomplex nitrogenase, which is actually responsible for nitrogen fixation. Cobalt is an essential micronutrient required by nitrogen-fixing organisms (Table 9.2). Some beneficial nutrients can substitute for micronutrients. A good example of this is the substitution of vanadium (V)in molybdenum (Mo)-requiring protein. Table 9.3 describes the adsorbed form and deficiency symptoms of essential elements. D. Beneficial Nutrients or Non-essential Elements Non-essential elements fall into two classes: (1)elements that are beneficial for some, but not all plants, and (2) elements that are not needed forplant growth. Elements that stimulate the growth of some plants include silicon, sodium,and selenium. Silicon is beneficial for rice, sugarcane, and other grass species. It accumulates preferentially in thestem tissues and appears to have a role in maintaining the structural integrity of the vas cular system,which is required to transport water, nutrients, carbohydrates, and other substances throughout the plant. Higher silicon concentrations are associated with greater drought tolerance. Sodium fertilization can improvethe growth of some C4 plants, celery, spinach, sugar beet, and turnips. Selenium appears to have general benefits related to oxidative stress tolerance and greater resistance to tissue damagecaused by patho gens and herbivores. Chapter9 Soil Fertility 191 Some elements can be absorbed in quantities that are toxic to plants. In acidic soils with water-soluble AP 10-20mg kg' or more, plants can experience aluminum toxicity. The decline in plant root levels of damage of cell membranes,and less cell division and elongation at the grow functions is related to oxidative ing tips due to Al replacement of Ca'+. In sensitive plants,the presence of AP in leaf tissues may reduce stomatal conductance and chlorophyll content, which reduces the photosynthesis rate. Plants grown in acidic soils are also prone to manganesetoxicity when they absorb more Mn than required for metabolic func tions,resulting in oxidative stress and destruction of indole-3-aceticacid (auxin),an important plant growth hormone. Manganese toxicity symptoms are be more severe in anoxic soils because oxygen expected to limitation will reduce several low-solubility compounds, such as MnO,, Mn,O,,and Mn,O, into Mn. Liming acidic soils with CaCO, or another neutralizing substance that raises the soil pH will increase the quantity of oxides and hydroxides, which form precipitates with AP and Mn. Since precipitated alumi num oxides/hydroxides and manganese oxides are not water-soluble, the risk of plant toxicity diminishes with increasing soil pH. Installing drainage systems that increase the soil oxygen level should reduce Mn² toxicity in plants. In salt-affected an abundance of sodium (Na'), SO, or CI creates salt stress for plants. Due to soils, osmosis, root cells tend to lose water , when the Na, SO; and CI levels are greater outside the root than inside the root. This can lead to physiological disorders within roots and other plant tissues, such as ion toxic ity, altered metabolic processes, oxidative stress, membrane disruption,and less cell division and expansion. Consequently. plants require more water to grow in salt-affected soils. Transpiration is responsible for the movement of water and the accumulation of water-soluble salts near the root. Evaporation also causes salt movement into topsoil, particularly in semi-arid and arid climates where annual water loss is greater than annual precipitation. Soil management can be effective in removing excess Na and CI from the root zone. Sprinkler irrigation combined with improved drainage could leach these salts through the soil profile and away from plant roots. Salt-tolerant crops should be planted in salt-affected soils. E. The Forms of Nutrients in Soil There are five forms of plant nutrients in the soil. 1. Minerals 2. Inorganic cations and anions bound in soil colloids 3. Nutrient complexes 4. Soluble or soil solution ions 5. Organic matter Minerals are the building blocks of soil. Minerals such as feldspar contain elements like K. Cations Ca and and anions are bound to the cation and anion exchange sites of soil as described com in chapter 6. Nutrient plexes refer to nutrients that have precipitatedor formed chemical associationswith other compounds in soil. An example is the interaction of P anions with the surface of calcium carbonate (CaCO,).Soluble ions are the nutrientsin the solution that move with soil water and are most readily available for plant uptake. The complex molecules in organic matter have many immobilized nutrientsthat become availableassoil microbes decom pose this material. Nutrient availability differs greatly depending on the form in which the nutrients exist. Phosphorus is a good example form ofP such ashydroxyapatite [(Ca,, (PO),(OH),)] is extremely insoluble and unusable by plants. Soluble P is readily available at neutral pH but can become available very quickly if the pH changes to become either more acid or more basic. Nitrogen is usually present in moderately or readily available forms in soil (Table 9.1). Most of the Earth's nitrogen exists as gaseous N, which is 78% theatmo of sphere, but it is unavailable for plants except for those few (such as legumes) capable of bacterially mediated N, fixation. Soluble/adsorbed nutrients and the deficiency symptom from cach essential nutrient can be seen in Table 9.3. 192 Chapter 9 Soil Fertility F. Movement of Nutrients from Soil to Plant Root There are three basic methods by which nutrients can make contact with the root surface for plant uptake. They are root interception, mass flow, and diffusion. Root interception: Root interception occurs when a nutrient comes into physical contact with the root surface. As a general rule, the occurrence ofroot interception increases as the root surface area andmass increase, thus enabling the plant to explore a greater amountofsoil. Root interception may be enhanced by myconhi zal fungi, which colonize roots and increase root exploration into the soil. Root interception is responsible for an appreciable amount of calcium uptake, andsome amountsofmagnesium,zine and manganese. Mass flow: Mass flow occurs when nutrients are transported ofroots by the movement of to the surface water in the soil (i.e., percolation, transpiration,or evaporation). The ofwater flow govems the rate amount of nutrients that are transported to the root surface. Therefore, mass flow decreases as soil water decreases. Most of the nitrogen, caleium, magnesium, sulfur, copper, boron, manganese,and molyb denum move to the root by mass flow. Diffusion: Diffusion is the movement ofa particular nutrientalong a concentration gradient. When there is a difference in the concentration of a particular nutrient within the soil solution, the nutrient will move froman area ofhigher concentration to an area oflower concentration. You may have observed the phe nomenon ofdiffusion when adding sugar to water. As the sugar dissolves, it moves through parts ofthe water with lower sugar concentration until it is evenly distributed,or unifornly concentrated. Diffusion delivers appreciable amountsof phosphorus,potassium, zine, and iron to the root surface. Diffusion is a relatively slow process compared to the mass flow of nutrients with water movement toward the root. Nutrient Uptake into the Root and Plant Cells Before both water and nutrients are incorporated into plants,both must first be absorbed by plant roots. Uptake of Water and Nutrients by Roots Root hairs, along with the rest ofthe root surface are the major sites ofwater and nutrient uptake. Water moves into the root through osmosis and capillary action. Soil water contains dissolved particles, such as plant nutrients. Thesedissolved particles within soil water are referred to assolution, Osmosisis the movement of soil water from areas of low solution concentra tion to areas of high solution concentration. Osmosis is essentially the diffusion of soil water. Capillary action results from water's adhesive (attraction to solid surfaces) and cohesion (attraction to other water molecules). Capillary action enables water to move upwards, against the force ofgravity,into the plant water from the surrounding soil. Nutrient ions move into the plant root by diffusion and cation exchange. Diffusion is the movenment of ions along a high-to-low concentration gradient. Cation ion exchange occurs when nutrient cations are attractedto the charged surface ofcells within the root, called cortex cells. When cation exchangeoccurs, the plant root releases a hydrogen ion. Thus,cat ion exchange in the root causes the pH of the immediately surrounding soil to deerease. Once water and nutrient ions enter the plant root, they move though spaces that exist within the root tissue between neighboring cells. Waterand nutrientsare then transported into the xylem, which conducts water and nutrientsto all parts of the plant. Once water and nutrients enter the xylem, both can be transportcd to other parts in the plant where the water and nutrients are needed. The basic outline of how nutrient ions are absorbed by plant cells follows. Chapter 9 Soil Fertility 193 Absorption of Nutrients into Plant Cells Plant cells contain barriers (plasmamembrane and tonoplast) that selectivelyregulate the movement of water and nutrients into and out of the cell. These cell barriers are: Permeable to oxygen,carbon dioxide, as well as certain compounds Semi-permeable to water Selectively permeable to inorganic ions and organic compounds,such as amino acids and sugars Nutrient ions may move across these barriers actively or passively. Passive transport is the diffusion of an ion along a concentration gradient. When the interior of the cell has a lower concentration of a specific nutrient than the outside of the cell, the nutrient can diffuse into the cell. This type oftransport requires no energy. Active transport is the movement of a nutrient ion into the cell that occurs against a concentration gradi ent. Unlike passive transport, this type of movement requires energy. G. Nutrient Mobility Within Plant An important characteristic of some nutrients is the ability to move within the plant tissue. In general, when certain nutrients are deficient in the plant tissue, that nutrient is able to translocate from older leaves to younger leaves where that nutrient is needed for growth.Nutrients with this ability are said to be mobile nutri ents and phosphorus,potassium, magnesium, and molybdenum. In contrast, immobile include nitrogen, nutrients donot have the ability to translocate from old to new growth.Immobile nutrients include caleium, sulfur, boron, copper, iron, manganese, and zinc. Nutrient mobility, or immobility, provides us with special clues when diagnosing deficiency symptoms. If the deficiency symptom appears first in the old growth,we know that the deficient nutrient is mobile. On the other hand., if the symptom appears in new growth,the deficient nutrient is immobile. Within the Soil Mobility of a nutrient within the soil is closely related to the chemical properties of the soil, such asCEC and AEC, as well as the soil conditions, such asmoisture. When there is sufficient moisture in the soil for leaching to occur, the percolating water can carry dissolved nutrients which from the soil will be subsequently lost profile. The nutrients that are casily leached are usually those nutrients that are less strongly held by soil par ticles. For instance, in soil with a high CEC and low AEC, nitrate (an anion) will leach much more readily than calcium (a cation).Additionally, in such soil, potassium (a monovalent cation) will leach more readily than calcium (divalent cation) since calcium is more strongly held to the soil particles than potassium. H. Nutrient Deficiency Symptoms Macronutrients Tables 9.2 and 9.3 describe the essential nutrient functions in plant development and metabolism as well as the deficiency symptoms for each nutrient.The mobility of cach nutrient within the plant determines the location of the plant where the first symptoms thatspecific nutrientdeficiency will appear (Tables 9.3 and 9.4). For exam of ple,nitrogen deficiency appears on older leaves first because it is mobile, and the plant defense system protects young leaves. Insandysoils, potassium can be easily leached, thus aswith nitrogen, the timing offertilizer appli cations is important to meet crop demand.It is important to consider how nutrients are retained and how they can move in soils. Soil texture includes clay type, and clay content will also influence the deficiency symptoms. Source:Adapted from https://edis.itas.ufl.edu'publication/AG462. Soil pH an important chemical characteristic that determines the availability of soil nutrients for plant is roots to absorb. The ideal soil pH for the availability of most nutrients is 6.5. However, with different pH ranges in the soils, one strategy to manage nutrients may not be useful for the entire state. Therefore, when possible. producers need to maintain their soil pH in the ideal range to get the maximum benefit of their applied fertilizers. Figure 9.2 explains the availability of diferent nutrients across different pH ranges. For example,soil P is most available for plant growth in the soil pH rangeof 6.0-7.0 (Figure 9.2). In general, soil pH is one important indicator in detecting soil fertility. Figure 9.2 https://www.no-tilfarmer.com/extresources/images/2019/CTG-Apri/Soil-Ph-Chart.jpeg It is Each nutrient has specific deficiency very important to diagnose nutrient deficiency in crop plants. symptoms, and visual analysis could help growers learn a lot about plant health. It is recommended that once the plant shows any deficiency symptoms, a representative tissue sample should be collected for lab analysis to confirm a deficiency. Figures 9.3 and 9.4 represent the nutrient deficiency of major and minor essential nutrients, respectively. Figure 9.3 https:/edis.ifas.ufl.eduimagelnqt1 kcy6v/screen Figure 9.4 https:/edis.ifas.ufl.edulimage/lkrqp5k7jb/screen Micronutrients Micronutrient deficiencies most commonly limit crop growth under the following conditions: Highly leached, acidic sandy soils: These soils are common in Florida, with a pH range of 4.5-6.5. The organic matter content in such soils rangesfrom 0.5% to 2%.These soils are not suited for crop produc tion without fertilizers, amendments, and proper drainage. Muck soils: These soils are distinctly black in color, with 20-80% organic matter content. Soils with high pH: These soils are common in southwestFlorida near the Immokalee area. The pH range in these soils is 7-8.5 becauseof excess application of lime and naturally occuring CaC0, below the soil surface. Soils that have been intensively cropped and heavily fertilized with macronutrients. I. Sources of Plant Nutrients In the soil, plants obtain mineral nutrients through root uptakefrom the soil solution. Sources of these soluble nutrients in soil include: 1. Decomposition of plant residues, animal remains, and soil microorganisms 2. Weathering of soil minerals 3. Fertilizer applications 4. Manures, composts, biosolids (sewage sludge), kelp (seaweed),and other organicamendments such as food processing byproducts 5. N-fixation by legumes 6. Ground rock productsincluding lime, rock phosphate,and greensand 7. Inorganic industrial byproductssuch as wood ash orcoal ash 8.Atmospheric deposition, such as N and S from acid rain or N-fixation by lightning discharges 9. Depositionof nutrient-rich sediment from erosion and flooding. Sources of Native Soil Fertility Parent material and SOM are two importantsourcesof native plant nutrients. Parent Materials Most of the plant nutrients come from the parent materials from which the soil is formed. Severaltypes of mineralshave naturally high levels of certain nutrients and can even be mined commercially based on their nutrient content (Table 9.5). Most of the micronutrients occur as trace clements in primary and secondary mineralssuch asoxides,sulfides, silicates, and carbonates. Soil Organic Matter Carbon, H, and O are supplied by air and water. Most of the N that is found in soil originally came from either N, fixation or from the precipitation of inorganic N from the atmosphere.Lightning discharge in the atmo sphere, for example, will produce a small amount of NO, More recently, fossil fuel burning and internal combustion engines have produced considerable amounts of NOx (nitrogen oxides) that are also ultimately Sources: https:/www.researchgate. netfigure Minerals- discussed-in-the-paperand- their-formulas_ tbl2 267699051 transformed to NO, and fall to earth. The N is rapidly taken up by plants and soil organismsand recycled in the readily available component of soil organic matter. Some of the N also becomes stably incorporated into humic and fulvic substances in soil, which have greatly diminished availability. On of the impacts of storing C and N in organic matter is that this pool fluctuates according to the decompo sition processes that occur in soil. The amount increases when the environment is wet and cool, and organic matter accumulates. The amount is as temperatures increase and organic matter decomposition increases. Min reduced eral soils with relatively hgh organic matter such as Mollisols, therefore, have considerably amounts of nutrients in storage. The deeper the A horizons of Mollisols, the greaterthe potential oforganic matter or nutrient storage. J. Factors Controling the Loss of Plant Nutrients Nutrients can also be lost from the soil system and become unavailable for plant uptake. Nutrient losses are not just costly and wasteful, they can be a source of environmental contamination when they reach lakes, rivers, and groundwater. Nutrient losses occur through: 1. Runoff loss of dissolved nutrients in water moving across the soil surface 2. Erosion loss ofnutrients in or attached to soil particles that are removed from fields by wind or water movement 3. Leaching loss of dissolved nutrients in water that moves down through the soil to groundwater or out of the field through drain lines 4. Gaseous losses to the atmosphere primarily losses of different N foms through volatilization and denitrification 5. Crop removal plant uptake and removal of nutrients from the field in harvested products 198 Chapter 9 Soil Fertility K. Soil Nutrient Cycles Agricultural crops absorb nutrient ions from the soil pore water to produce cdible biomass (eg.grain, oilseed, forage, fruit, and tubers) and non-edible biomass, some of which is harvested for animal bedding and other purposes (ie,second-generation biofuels are made from non-edible stems and leaves). Fertilizer addition will replenish the plant-available nutrients, but the nutrient ions from fertilizer will not necessarily remain in the soil pore water because they are subject to the biological,chemical, and physical reactions of soil nutrient cycles,described below. Nitrogen Cycling Nitrogen cycling contributes to plant-availablenitrogen. The soil nitrogen cycle (Figure 9.5) is well studied, due to the central importance ofnitrogen for chlorophyll production, Calvin cycle reactions that take place in chloroplasts during photosynthesis, and protein synthesis in plants. NO,N,O NH Organic residue Soil matter organic H H,NC +NH, NO, NO, NO,N,0, N, NH, OF Soil pore water Animal manure NH. Clay Organo-mineral NO, NH NH, NH, NH, complex NO. N fertilizer granule NH. Leaching Figure 9.5 Simplified version of the soil nitrogen cycle. Source: O Joann Whalen is licensed under a CC BY (Atribution) license. One way that agriculturalcrops can obtain nitrogen is through a symbiotic relationship with N,-fixing prokaryotes. The best known of these are soil bacteria in the Rhizobium genera, but there are many others (Table9.6). The N,-fixing prokaryotes possess the nitrogenase enzyme,which can transform atmospheric N, into ammonia (NH,).The nitrogenase complex reduces N, to NH, and it also reduces H to H,. The energy (ATP) for this reaction comes from the breakdown, via glycolysis,of photosynthates that are transferred from the host plant to the symbiont. The NH, (g) product diffuses out of the bacteriod's protoplasm into the root cortex, where it is protonated with 2 H ions. The NH, then binds to glutamate to produce glutamine or is transformed into ureides such as allantoin, allantoic acid, or citrulline, before it is translocated from the root to other parts of the plant. Source: Adapted from Rascio and La Rocca (2013). Legumesin the Fabaceae form a symbiosis with N,-fixing bacteria, which inhabit nodules in their family roots. The symbiosis is initiated when roots secrete flavonoids, which are chemo-attractants for the nodulat ing bacteria (rhizobia)to attach to the root hairs. The flavonoid signal activates the nodulation (nod) genes in the rhizobia,which synthesizes Nod factors that bind to surface protein receptors at the subapical root tip. This causes the root hair to curl and entrap the rhizobia, and the plasma membrane invaginates to form the infection thread, a tubular structure that extends from the root hair tip to the lower cells of the root cortex. Rhizobia moves through the infection thread and multiplies, and root cortex cells proliferate into the nodule primordia, which will eventually become a fully-formed nodule enveloped in a membrane that prevents oxy gen diffusion. This protective membrane prevents nitrogenase from being inactivated by oxygen. Atthis stage, the rhizobial bacteria become bacteroids capable of biological N, fixation. Although N, is abundant (78% of the Earth's atmosphere), the biological N, fixation reaction is energeti cally demanding. In theory, 16 ATP are required to reduce one molecule of N, to NH, but the actual ener getic requirement is generally higher due to inefficiencies in the process. Therefore, biological N, fixation is a strictly controlled proces. All other available forns of nitrogen, such as NH,", NO, ,and amino acids, will inactivate the nitrogenase complex and inhibit the expression of the nif genes that encode for the nitrogenase components. In addition, the reaction products NH, and H, also inhibit nitrogenase activity. Consequently, most legumes acquire 40-759% of their nitrogen requirement from biological N, fixation, and the rest is absorbed as water-soluble NH," and NO, from the soil pore water. Associative N,-fixing bacteria live in the rhizosphere (in the soil pores and on soil particles close to plant roots) or as endophytes (within the plant of grasses such as sugareane, rice, wheat, sorghum,maize, and tissues) others. Somecommon genera are Azospirillum and Herbaspirillum, and thereare many others (Table 9.6). These bacteria do not form nodules. Instead,they live on the root surface or within the intercelular space and xylem vessels of stems and leaves. They obtain energy from plant photosynthates and transfer a portion ofthe NH," gen erated from N,fixation to the plant. There is considerable variability in their contribution tocrop nitrogen require ments. Insugarcane, Gluconacetobacter diazotrophicus can supply 0-60% of the ntrogen requirement under field conditions. Cereals and oilsceds may obtain I-25 kg N ha y' from their associative N,-fixing bacteria. The rest ofthe nitrogen needed by these crops must be absorbed from the soil pore water as NH, and NO, ions. 200 Chapter 9 Soil Fertility The variation in nitrogen mineralization potentialcan vary substantially as a result of soil moisture, tem perature, soil type, cropping system, or soil management. Sometimes, the greatest variation occurred from field to ficld meaning the value of site-specific soil testing to quantify N mineralization potential. Nitrogen mineralization, is the main source of NH, in unfertilized soil. This naturally-occurring process releases water-soluble NH, from decaying plants, animals, and microbial residues, which were recently added to soilor are part of the soil organic matter. Nitrogen mineralization occurs more quickly with increas ing soil temperature and is optimal when soil moisture reaches 60% water-filled pore space. Soil conditions that favor bacterial activity (pH from 6 to 7.5, low salinity) will stimulate mineralization since bacteria have a faster generation time and greater capacity to produce protein-degrading enzymes than fungi. Nitrogen mineralization is also affected by the C:N ratio in organic residues. The C:N ratio is the mass of the total organic carbon divided by the mass of total nitrogen in an organic substance. Organic residues with a largerproportion of organic carbon provide substrates forheterotrophic microorganisms, which use carbon for metabolic processes and biomass production, and respire CO,. growth becomes limited by a lack of If their nitrogen in the organic residue, heterotrophic microorganisms will immobilize NH, and NO, from the soil pore water. Organic residues with a C:N ratio > 20 such as woodchips, straw, and grass-based residues tend to be rich in organic carbon and relatively impoverished in total nitrogen, which causes immobilization. Organic residues with a C:N ratio < 20such as food-based compost and decomposed animal manure are relatively enriched in total nitrogen, so they will mineralize and increase the NH, concentration in soil pore water. Many fertilizers are a source of NH, in soil. Granular fertilizer such as ammonium sulfate, (NH,),So,. is water-soluble and dissolves to release NH,. Urea fertilizer is hydrolyzed by the urease enzyme, which is produced by naturally-0ccurring soil microorganisms and plants, to release NH,. A similar reaction occurs in animal manure, which contains urea from animal urine. Chicken manure collected from laying facilitieswithout bedding is enriched with urea-like molecules, due to the fact that 80% of the nitrogen excreted is in the form of uric acid. It takes several days to a week to transform uric acid to NH, in warm,moist soil. Pig manure that is collected by spray-washing the barns and stored in a liquid form also has a high pro portion ofNH,, due to urea hydrolysis and protein degradation that occurs in the manure storage. For exam ple, liquid pig slurry contains >90% water, has a C:N ratio of 3, and the NH, form represents 75% of the total N content. The high proportion ofreadily-degradable uric acid in chicken manure, and the large amount of water-soluble NH, in liquid pig slurry, makes these manures "fast-release" nitrogen fertilizer, compared to animal manure mixed with bedding, which has a lower water content. Ammonia oxidizers and nitrifiers are responsible for transforming the NH," to NO,.The ammoniaoxi dizers are a diverse group of prokaryotes, including bacteria and archaea, which possess the amo and hao genes that encode for the enzymes to catalyze the following two-step reaction: ammonia Chapter9 Soil Fertility 201 Nitrifiers are prokaryotes and eukaryotes that possess the nxr gene and are capable of converting nitrite (NO, ) into NO,", as: nitrite oxidoreductase NO, + 5H* + 4e NO, + 2H*+ 2e Ammonia oxidation and nitrification reactions occur quickly in ficld soils during the growing season. Consequently, NH, released from nitrogen mineralization or added in water-soluble animal fertilizer or manure will be transformed into NO, days to weeks. This understanding becomes important when within deciding when to apply fertilizer becausewe would like crops to absorb most of the water-soluble NH, and NO, from fertilizer as quickly as possible after the fertilizer application. Otherwise, reactions in the nitrogen cycle will remove these nutrient ions from the soil pore water, thereby reducing the amountof plant-available nitrogen forcrops and increasing the risk of environmental pollution. What Do Cause Loss of Plant-available Nitrogen? The NH," and NO, ions in the soil pore waterareremoved through biological, chemical, and physical pro cesses. Nitrogen uptake by plants and immobilization by microorganismsis driven by nitrogen requirements for protein synthesis and formation of genctic material. In plants, nitrogen is a major component ofproteins of the Calvin cycle (eg., about 20-309% of the leaf nitrogen is found in the RuBisCO enzyme that initiates car bohydrate production), the thylakoids and the chlorophyll molecule and thus essential for CO, fixation associ ated with photosynthesis. NO, and NO, asclectron acceptors are common. These reactions per Soil biological reactions that use mit soil and fungito acquire oxygen when the soil environmentis anoxic. High soil moisture content bacteria can create anoxic conditions, as can intense biological activity that consumes oxygen and produces carbon dioxide, such as in the rhizosphere or in the detritusphere (i.., around decomposing organic matter). Nitrifier-denitrification is one such reaction and is expected when the soil moisture content is from 50% to 80% of water-filled pore space. nitrite nitric oxide reductase reductase NO, (aq) + NO (B) N,O (e) Denitrification is another biological reduction reaction that consumes NO,,and it is expected when the soil moisture content exceeds 80% water-filled pore space. nitrate nitrite nitric oxide nitrous oxide reductase reductase reductase reductase NO, (aq) NO, (aq) + NO (B) N,O (e) N, (e) Animal manure can lose up to 50% of the total nitrogen excreted by the animal as NH, during manure storage and land application. Urea fertilizer is also susceptible to NH, loss, especially in alkaline soils that have an excess OH and HCO, in the soil pore water, since H associates with these counter-anions rather of than with NH,Volatilization of NH,is undesirable becausethe transfer of gaseousnitrogen from agricultural fields to theatmosphere reducesplant-available nitrogen forthe crop. Eventually, the NH, will return to the ground through wet and dry deposition, which results in the unintentional fertilization of non-agricultural land and aquatic systems. Ammonium fixation is a chemical reaction that removes NH, from the soil pore water. This occurs when NH, adsorbs to exchange sites on organo-mineralsurfaces. Ammonium fixation is greater in clayey soils, which have greater reactive surface area and more adsorption sites in the interlattices (2:1 clays only), than sandy soils. Due to its 1+ charge and small ionic radius, NH, is likely to bind to the interlattice, which reducesthe amount NH, in the soil pore water. This could account for a reserve of as much as 90-460 mg of NH, kg soil in fine-textured clays. In contrast, the N0, molecule is loosely retained in the soil matrix due to its size and negative chargeand is more likely to leach through the soil profile and below the plant root zone. Nitrate leaching is affected by soil porosity and the water infiltration rate after snowmelt, rainfall, and irriga tion events. Finally, both NH, and NO, are susceptible to loss from soil througherosion and surface runoff processes. Wind and water erode soil particles that contain adsorbed NH,, while runoffwater transports NO, from saturated soils through overland flow. Phosphorus Cycling Plant-available phosphorus is derived from biological and geochemical processes (Figure 9.6). The biological source is organic phosphorus,which is hydrolyzed by phosphataseenzymes from plant roots and soil micro organisms.Organic phosphorus compounds are abundant in plant residues and undigested plant materials. are major phosphate storage compounds that contribute to cell wall biosynthesis, cell-to-cell com Inositols munication,storage and transport of plant hormones and other stress-related molecules, and osmoregulation. Degradationof myoinositol releases water-soluble H,PO,, as follows: OH HO OH HQ HO OH HOP Phosphatase OH H,PO; + H* + OH OH HO HO HO HO. OH -OH myo inositol OH scyllo Inosose OH Primary minerals such as apatite undergo weatheringto release H,PO, into soil pore water. Other geo chemicalsourcesincludeH,PO, which is desorbed from clay surfaces or dissolved from secondary minerals, iron, aluminum, calcium,and magnesium phosphates. Weathering and dissolution processes require water. The dissolution of calcium phosphatesand magnesium phosphates is accelerated by the presenceof carbonic acid (H,C0, HCO, + H") and other acidic substances, however, iron phosphatesand aluminum phos phates become less soluble in acidic soils. Inorganicacids that contribute to the solubilization of H,PO, from calcium phosphatesand magnesium phosphatesinclude H,SO, and HCI. Organic acids produced by plant roots and soil microorganisms lowerthe pH in their surroundings,result ing in localized H,PO, solubilization; in addition, organic acidscan displace H,PO, that is adsorbedonto clayand organo-mineralsurfaces. Organic acids that are effective in this regard include acetic, lactic, malonic, and salicylic acids. Soil bacteria that produce organic acids, such malic, tartaric, oxalic, citric, p-hydrobenzoic, as those from the genera Pseudomonas, Bacillus, Rhizobium, and Burkholderiacan be isolated, cultivated, and re-inoculated into the soil to increase H,PO, released via dissolution but have not proven to be reliable at solubilizing HPO, under field conditions. Arbuscular mycorrhizal fungi also produce organic acids that increase water-soluble H,PO,, which they then absorb through their extra-radial hyphae. Since arbuscular mycorrhizal fungi are plant symbionts, someof the H,PO, acquired by the fungus can be transferred to the plant host through low- and high-affinity phosphorus transporters. What Do Cause Loss of Plant-available Phosphorus? The H,PO, ions in the soil pore water are removed through biological, chemical, and physical processes. Phosphorus uptake by plants and immobilization in microbial biomass is necessary for energy storage and transfer reactions,as well as the formation of nucleic acids and phospholipids. Phosphorusretention reactions reduce the H,PO, concentration in soil pore water because H,PO, adsorbs to organo-mineral surfaces and to the surface of iron and aluminum sesquioxides, namely iron oxide (Fe,03), iron hydroxide (Fe(OH),), and aluminum hydroxide (Al(OH),). These reactions are common in acidic soils but occur in all soils to some extent. In general, soils with a higher clay content will adsorb more H,PO, partic. ularly when they contain 2:1 expanding lattice clays such as smectite. The hydrated sesquioxides of iron and aluminum bind H,PO, tightly and render it unavailable for plant uptake. In addition, H,PO, precipitates with Fe, Al, and Ca present in the soil pore water. This produces precipitates such as Fe(OH),H,PO, and the variscite-like compound, AlOH),H,PO, in acidic soils. In neutral to alkaline soils, the H,PO, precipitates with Ca to form slightly soluble dicalcium phosphate dibydrate, CaHPO,.Further reaction with Ca(OH), and CaCO, in alkaline soils leads to the formation of insoluble hydroxylapatite (eg.(Ca,(PO)], CaOH,) or insoluble carbonated calcium apatite (eg.carbonato-apatite: (Ca,(POJ,], CaCO,). Soil with a high degree of phosphorus saturation has elevatcd H,PO,, relative to the amount of extract able Al that could bind with H,PO,. Therefore, more water-soluble H,PO,"remains in the soil pore water and could leach after snowmelt,rainfall, and irrigation events. In addition, erosion of soil particles that contain adsorbed H,PO, will transport particulate P, while surface runoff will transport the dissolved phosphorus compounds at the soil surface through overland flow. The magnitude of phosphorus loss through thesepath ways depends upon the concentration of water-soluble H,PO, and particulate P in the surface soil,as well as theamount of leaching and severity of surface erosion processes. 204 Chapter 9 Soil Fertility Sulfur Cycling Plant-availablesulfur can originate from weathering of parent material, atmospheric deposition, dissolution of gypsum (CaSO,), and mineralization of organic matter (Figure9.7). Plutonic rocks such as pyrite contain clemental sulfur (So) that is oxidized to SO during the weathering process. Most oxidation reactions are mediated by autotrophic fungi and bacteria, such as Thiobacillus, which is abundant in the rhizosphere. Rocks so SO, Minerals Organo-mineral so, SO, complex (eg, Caso,) So, so, so, Soil pore water so, Soil organic matter Leaching Figure 9.7 Figure Simplified version of the soil sulfur cycle. Source: Joann Whalen is licensed under a CC BY (Attribution) license. The conversion of So to SO is extremely variable in soils, due to natural variations in the distribution of sulfur-oxidizing microorganisms. In general, sulfur oxidation reactions occur rapidly in warmer soils with moisture content with around 60% water-filled pore space and soil pH from 6 to 7.5. In addition, So, is released from the dissolution hydrated salts in soil, such as CaSO, 2H,0 and MgSO, 7 H,0. of Sulfur enters soil from atmospheric sources through dry and wet deposition. Several natural phenomena emit sulfur to the atmosphere, including particulate sulfur compounds that come from the weathering of con tinental crusts (dust) and sea spray. Smallparticles of sulfur are transported by wind and deposited onto the surface of soil or vegetation. Gascousemissions of sulfur dioxide, hydrogen sulfide, dimethyl sulfide, and other gases from volcanic eruptions, swamps, and coastal wetlands are another source. In the atmosphere, these gases tend to be oxidized to sulfuric acid and aerosol sulfate and then return to the ground in rainfall and snow.Thesebiogenic sources of sulfuraccount for