ABE 66 Land and Water Conservation Engineering PDF
Document Details
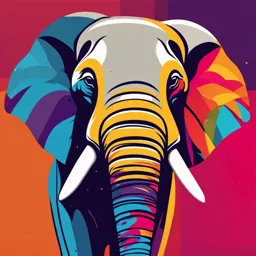
Uploaded by SuperbIridium
Central Mindanao University
2023
Indie G. Dapin and Audry L. Anacio
Tags
Summary
This presentation delves into land and water conservation engineering, focusing on soil erosion, its control, and sediment transport. It covers various aspects, from factors influencing erosion to measurement techniques and prediction methods. The presentation emphasizes research on soil erosion in Bukidnon, Philippines, utilizing the RUSLE model.
Full Transcript
ABE 66 Land and Water Conservation Engineering Indie G. Dapin Audry L. Anacio Department of Agricultural and Biosystems Engineering College of Engineering Central Mindanao University Soil Erosion and...
ABE 66 Land and Water Conservation Engineering Indie G. Dapin Audry L. Anacio Department of Agricultural and Biosystems Engineering College of Engineering Central Mindanao University Soil Erosion and Its Control Mechanics of soil erosion Measurement of soil erosion Soil erosion control Sediment transport and sedimentation Transported materials/sediments Vertical distribution of sediment flow Measurement/prediction of sediment transport and sediment yield Soil Erosion - refers to the detachment and removal of soil (rock) particles from the land surface, primarily by the action of water, wind, or other natural forces. It involves the initial phase where soil particles are loosened and displaced. Geological Erosion - natural soil erosion by water. This Primary causes of soil erosion: includes soil-forming as well as soil-eroding processes unsustainable farming practices, that maintain the soil in a favorable balance suitable for deforestation, the growth of most plants. Caused the formation of overgrazing, topographic features, such as canyons, stream channels, mining, and and valleys. Accelerated erosion is a type of erosion associated by construction activities human disturbances (anthropogenic). Dapin, Indie G., and Victor B. Ella. 2023. "GIS-Based Soil Erosion Risk Assessment in the Watersheds of Bukidnon, Philippines Using the RUSLE Model" Sustainability 15, no. 4: 3325. https://doi.org/10.3390/su15043325 Vanoni, V. A. 2006. Sedimentation Engineering. ASCE Manuals and Reports on Engineering Practice No. 54. American Society of Civil Engineers Soil Erosion by water Soil erosion by water is a natural process linked to the hydrologic cycle. On a small scale, soil particles are mainly detached by the impact of rainfall, but at larger scales, other water erosion processes become more dominant. Major factors affecting soil erosion: ▪ Climate ▪ soil properties ▪ vegetation characteristics, and ▪ Topography ▪ (Disturbances) Dapin, Indie G., and Victor B. Ella. 2023. "GIS-Based Soil Erosion Risk Assessment in the Watersheds of Bukidnon, Philippines Using the RUSLE Model" Sustainability 15, no. 4: 3325. https://doi.org/10.3390/su15043325 Huffman, R. L., Fangmeier, D. D., Elliot, W. J., Workman, S. R. 2013. Soil and Water Conservation Engineering. 7th edition. American Society of Agricultural and Biological Engineers. Soil Erosion by water Major factors affecting soil erosion: ▪ Climate ▪ soil properties Precipitation soil structure, texture, organic matter, Rainfall amount, intensity, and energy, water content, clay mineralogy, and raindrop energy, drop diameter, and runoff density, and soil chemical and biological rates, particularly on permeable soils; runoff rate; snow accumulation amount and melt rate characteristics Temperature, Wind, Humidity, and Solar Radiation Huffman, R. L., Fangmeier, D. D., Elliot, W. J., Workman, S. R. 2013. Soil and Water Conservation Engineering. 7th edition. American Society of Agricultural and Biological Engineers. Soil Erosion by water Major factors affecting soil erosion: ▪ vegetation characteristics interception, retardation, physical restraint, aggregation and porosity improvement, increased biological activity, transpiration, crop residue management, tillage management, ▪ Topography slope length and steepness, shape (including concave, uniform, or convex), and size and shape of the watershed. ▪ Disturbances Natural Prolonged period of wet weather, severe storms, fire Human-induced (anthropogenic) Construction, tillage, agricultural practices Huffman, R. L., Fangmeier, D. D., Elliot, W. J., Workman, S. R. 2013. Soil and Water Conservation Engineering. 7th edition. American Society of Agricultural and Biological Engineers. Soil Erosion Effects of Soil erosion ▪ Soil erosion from catchment areas and the subsequent sediment buildup in waterways ▪ can decrease reservoir storage capacity and ▪ deteriorate downstream water quality. ▪ Excessive soil erosion ▪ leads to a decline in soil fertility and crop yields, posing serious risks to local, national, and global food production and environmental sustainability. ▪ limits the agricultural potential of the land by reducing soil productivity and contributes to water pollution by adding suspended particles and nutrients to water bodies. ▪ eroded land becomes more vulnerable to other environmental challenges. Dapin, Indie G., and Victor B. Ella. 2023. "GIS-Based Soil Erosion Risk Assessment in the Watersheds of Bukidnon, Philippines Using the RUSLE Model" Sustainability 15, no. 4: 3325. https://doi.org/10.3390/su15043325 Soil Erosion Soil erosion is the product of many interactive subprocesses In the Philippines, water is primary agent of soil erosion. Two interactive processes: detachment of soil particles from the soil surface by falling raindrops and flowing water transport of detached particles Note: both processes need to be at work for considerable soil loss to occur without the detachment process, erosion will not even start without the transport process, erosion will be much limited soil detachment and transport could either be by water, wind or any mechanical force applied to the soil Luyun, R.A. (2015). Lecture notes in AENG 243: Advanced Soil and Water Conservation Engineering David, W.P. Soil and Water Conservation Planning–Policy Issues and Recommendations. Soil Erosion Factors influencing the two primary interactive processes Land cover, soil physical and chemical properties, raindrops energy, flow velocities and hydraulic properties, cover management, slope, slope length and stiffness, and conservation practices. Luyun, R.A. (2015). Lecture notes in AENG 243: Advanced Soil and Water Conservation Engineering David, W.P. Soil and Water Conservation Planning–Policy Issues and Recommendations. Mechanics of soil erosion Soil and Water Conservation Engineering Soil Web. SoilWeb/Soil Management/Soil Erosion; The University of British Columbia: 2003. Available online: http://wiki. ubc.ca/LFS:SoilWeb/Soil_Management/Soil_Erosion (accessed on 23 May 2016) Soil Erosion by water Erosive agents are rainfall and runoff each has a detaching and transporting capability soil erosion over a land surface is the product of raindrop impact and overland flow four interactive erosion sub-processes, namely: 1. detachment by rainfall 2. transport by rainfall 3. detachment (scour) by runoff (overland or channelized flow) 4. transport by runoff Luyun, R.A. (2015). Lecture notes in AENG 243: Advanced Soil and Water Conservation Engineering Measurement of soil erosion Selecting the methods of estimating soil erosion Must be objective-based ▪ scale (field or river basin-scale); ▪ the rate at which a study will be undertaken (short or long- duration); ▪ the level of prediction accuracy, ▪ the level of simplicity and complexity, and ▪ the level of application Dapin, Indie G., and Victor B. Ella. 2023. "GIS-Based Soil Erosion Risk Assessment in the Watersheds of Bukidnon, Philippines Using the RUSLE Model" Sustainability 15, no. 4: 3325. https://doi.org/10.3390/su15043325 Methods of estimating soil erosion Plot-experiment (Field method) - Conducted at several locations in a field and extrapolating the results to the entire landscape Employing soil erosion models (Soil erosion modeling) - for applications on a much broader scale Ways to obtaining information about erosion Qualitative observations involve the simple viewing of the land, looking for various signs or indicators of erosion; are not all that precise, but they can be very informative. Indicators of erosion: 1. Rills or gullies reflect concentrated overland flow where sheet flow might once have existed. 2. Exposed E horizons often appear as white patches on hilltops or convex slopes where dark soils are otherwise prevalent. https://www.la.utexas.edu/users/wd/courses/373F/notes/lec17ero.html Qualitative observations Indicators of erosion: 3. Exhumed rocks, either relatively large boulders that were exposed by removal of overlying soil, or rocks lying loose on the surface due to the soil in which they were once firmly embedded, typically indicate soil loss 4. Exposed roots are similar to exhumed boulders--what should be beneath the ground surface is not. One must be careful, however, and not confused exposed roots with buttress root https://www.la.utexas.edu/users/wd/courses/373F/notes/lec17ero.html Qualitative observations Indicators of erosion: 5. Pedestals are the result of items on the surface, typically rocks but sometimes artifacts of human activities, protecting the underlying soil from the impact of raindrops. They indicate that surrounding, unprotected soils have been eroded, while those directly under the rock have not. 6. Debris dams or traps are comprised of fallen trees and branches. They tend to catch sediment that has eroded from upslope or upstream. https://www.la.utexas.edu/users/wd/courses/373F/notes/lec17ero.html Ways to obtaining information about erosion Quantitative observations involve collection and measurement Types: 1. Direct or Passive measurements most accurate; most aborious and time consuming; involve collecting and weighing deposited materials (in small setting); involve measuring the widths, depths, and lengths of gullies and calculating the volume of material lost (large setting); a. A-horizon reconstruction, whereby the thickness of the no longer existent A-horizon on eroded lands can be estimated by comparison with surrounding lands of otherwise similar soil that have not been eroded; and b. Natural benchmarks such as trees or boulders that might have soil marks, not unlike the high water marks on buildings in recently flooded areas. Volumetric measurements can be estimated on the basis of the distance between that mark and the current surface. https://www.la.utexas.edu/users/wd/courses/373F/notes/lec17ero.html Ways to obtaining information about erosion Quantitative observations Types: 2. Indirect or Active measurements center around preselected points, devices set-up to carry- out long-term study, and the actual measuring of ongoing soil loss; more appropriately considered estimates as the removed material is not available for study Erosion pins, metal rods set into the ground [examples], typically with a portion sticking up above the surface some known and recorded amount (10 cm). Flagging is tied to the stake to warn possible disturbers. The distances between the tops of the pins and the surface are recorded at regular intervals (e.g., once a month) over an extended period of time. A variation on this theme is to use a very long spike driven through a washer to ground level. Over time, the distance the washer drops from the top of the spike to the eroded ground surface can be recorded. A variation on this theme is to use bottle caps with nails driven through them, thereby becoming tantamount to pedestalling as described above. Determining the height of the underlying pedestal and the time it took to develop will inform of the rate of erosion. https://www.la.utexas.edu/users/wd/courses/373F/notes/lec17ero.html Field method of soil measurement Volumetric measurement for rills, gullies, and fans; can capture the erosion rate of an actual landscape; real volume of eroded soil can be computed; may only be useful at the farm scale and is impracticable at the river basin scale; requires constant monitoring at several sampling sites (more sampling sites – resulting higher measurement accuracy). Using rainfall simulator and structure-from-motion (SfM) photogrammetry technique for plot experiments (esp. bare soil); Steps: Initial DEM of the plot -> artificial rainfall (rainfall simulator) to moisten the soil surface -> after RF DEM of the plot -> determine the difference between the two DEMs = reflect the amount of soil loss over a specific intensity and duration of precipitation (the eroded soil) -> compare the collected soil to the result of the difference between DEMs to evaluate a good match. Field method Radionuclide Fallout (RNF) isotope (e.g. 𝟏𝟑𝟕𝑪𝒔) a technique for determining the distribution of soil loss rate; developed to qualitatively measure erosion using isotope traces; innovative, quick, and cost- effective way for assessing soil erosion, complementing the conventional method; For this procedure, two types of 137Cs soil samples are required: one for the reference of intact soils and the other for the assessment of soil loss. The magnitude of measured 137Cs soils surface radioactivity at sampling locations for soil loss is always less than the magnitude at reference sites. The greater the number of survey locations for both types, the clearer the distribution of soil loss will be. This method of calculating soil erosion loss may be useful on a broader scale. Yet, to account for the corresponding amount of soil erosion for the magnitude of 137Cs soils surface radioactivity, another model will be required. Classification of soil erosion models Quantitative - empirical, semi-empirical, deterministic, or physical and process-based. - empirical and semi-empirical models: Universal Soil Loss Equation (USLE), Revised USLE (RUSLE), and Modified USLE (MUSLE) models, - physical and process-based models: Water Erosion Prediction Project (WEPP), Soil and Water Assessment Tool (SWAT), European Soil Erosion Model (EUROSEM), etc. Qualitative - tend to be probabilistic, statistical, or based on artificial intelligence. Models: ▪ Frequency Ratio (FR), ▪ Evidential belief function (EBF), ▪ Multi-criteria Decision Analysis (MCDA) - Analytical Hierarchy Process (AHP), and Multi Influence Factor (MIF) Employing classifiers such as Random Forest and Decision Trees. The RUSLE Model - The Revised Universal Soil Loss Equation, is a well-known and widely used empirical model for estimating soil erosion 𝐴 = 𝑅 𝑥 𝐾 𝑥 𝐿𝑆 𝑥 𝐶 𝑥 𝑃; in t/ha/y A = soil loss rate in tons/ha/yr R = rainfall and runoff erosivity factor (MJ mm/ha/h/y), K = soil erodibility factor LS = slope length and steepness factor (which may be approximated on the basis of percent slope) C = cover management factor P = conservation or management or support practice factor GIS can be used to streamline the application of the RUSLE model For more information about RUSLE, read the following: Dapin, Indie G., and Victor B. Ella. 2023. "GIS-Based Soil Erosion Risk Assessment in the Watersheds of Bukidnon, Philippines Using the RUSLE Model" Sustainability 15, no. 4: 3325. https://doi.org/10.3390/su15043325 Ghosal, K.; Das Bhattacharya, S. A review of RUSLE Model. J. Indian Soc. Remote Sens. 2020, 48, 689–707. David, W. P. (1988). Soil and Water Conservation Planning: Policy Issues and Recommendations. Journal of Philippine Development, 15(1), 47–84. Huffman, R. L., Fangmeier, D. D., Elliot, W. J., Workman, S. R. 2013. Soil and Water Conservation Engineering. 7th edition. American Society of Agricultural and Biological Engineers. Reading assignment Read about the types of soil erosion. Types of Soil Erosion 1. Water erosion ▪ Splash / Raindrop Erosion – primarily caused by raindrop ▪ Sheet erosion- a thin film of soil layer detached and transported by water flowing on the land surface. ▪ Interrill erosion – combination and splash and sheet erosion ▪ Rill erosion- finger-like rills appear on the soil surface. ▪ Gully erosion- advanced stage of rill erosion. Rills when neglected develop in size and become gullies. ▪ Stream bank erosion – erosion of stream banks by flowing water. ▪ Coastal erosion- erosion caused by wave action on the seashore. 2. Wind erosion – caused by high velocity winds moving over barren land surfaces. 3. Slip erosion – land slides and slips due to saturation of steep hills and slopes. Raindrop/Splash Erosion - soil detachment and transport resulting from the impact of water drops directly on soil particles or on thin water surfaces. relationship between erosion, rainfall momentum, and energy is determined by raindrop mass, size distribution, shape, velocity, and direction. factors affecting the direction and distance of soil splash are slope, wind, surface condition, and impediments to splash such as vegetative cover and mulches. Raindrop impact on bare soil not only causes splash but also breaks down soil aggregates and causes deterioration of soil structure. This leads to increased surface sealing, runoff, and erosion. Sheet Erosion - considered to be a uniform removal of soil in thin layers from sloping land, resulting from sheet or overland flow. rarely occurs because minute channels (rills) form almost simultaneously with the first detachment and movement of soil particles The eroding and transporting ability of overland flow depends on the rainfall intensity, infiltration rate, slope steepness, soil properties, and vegetative cover. Interrill Erosion - Splash and sheet erosion combined a function of soil properties, rainfall intensity, runoff rate, and slope. 𝐷𝑖 = 𝐾𝑖 𝑖 𝑞 𝑆𝑓 𝐶𝑣 𝐷𝑖 = interrill erosion rate (𝑘𝑔 𝑚−2 𝑠 −1), 𝐾𝑖 = interrill erodibility of soil (𝑘𝑔 𝑠 𝑚−4) (refer Huffman, et al (2013) Chapter 7), 𝑖 = rainfall intensity (m/s), 𝑞 = runoff rate (m/s), 𝑆𝑓 = interrill slope factor = 1.05 – 0.85𝑒 −4sin𝜃 , 𝐶𝑣 = cover adjustment factor, including effects of soil surface cover, canopy cover, root characteristics and/or sealing and crusting, with values from near zero to 1.0, 𝜃 = interrill slope angle. Huffman, R. L., Fangmeier, D. D., Elliot, W. J., Workman, S. R. 2013. Soil and Water Conservation Engineering. 7th edition. American Society of Agricultural and Biological Engineers. Rill Erosion - the detachment and transport of soil by a concentrated flow of water - the predominant form of surface erosion under most conditions - a function of the flow rate or hydraulic shear 𝜏 of the water flowing in the rill, the soil’s rill erodibility 𝐾𝑟 , and critical shear 𝜏𝑐 , the shear below which soil detachment is negligible Rills are eroded channels that are small enough to be removed by normal tillage operations Rill Erosion 𝑞𝑠 𝐷𝑟 = 𝐾𝑟 𝜏 − 𝜏𝑐 1− The sediment transport capacity 𝑇𝑐 𝐷𝑟 = rill detachment rate (𝑘𝑔 𝑚−2 𝑠 −1 ), 𝑇𝑐 = 𝐵𝜏 1.5 𝐾𝑟 = rill erodibility, due to shear (𝑘𝑔 𝑚−2 𝑠 −1 4) (refer Huffman, et al (2013) Chapter 7) 𝜏 = hydraulic shear of flowing water (Pa) 𝑇𝑐 = sediment transport capacity of rill 𝜏𝑐 = critical shear below which no rill erosion occurs (Pa) (𝑘𝑔 𝑚−1 𝑠 −1 ), 𝑞𝑠 = rate of sediment flow in the rill (𝑘𝑔 𝑚−1 𝑠 −1 ), 𝐵 = transport coefficient based on soil 𝑇𝑐 = sediment transport capacity of rill (𝑘𝑔 𝑚−1 𝑠 −1 ), and water properties generally between 𝜃 = interrill slope angle. 0.01 and 0.1 (dimensionless) (Elliot et al., 1989). The hydraulic shear τ is defined as 𝜏 = 𝛾𝑅𝑆 𝛾 = specific weight 𝑅 = hydraulic radius 𝑆 = hydraulic gradient of rill flow Huffman, R. L., Fangmeier, D. D., Elliot, W. J., Workman, S. R. 2013. Soil and Water Conservation Engineering. 7th edition. American Society of Agricultural and Biological Engineers. Gully Erosion - produces channels larger than rills. These are ephemeral channels that carry water during and immediately after rains. - gullies cannot be obliterated by tillage Factors affecting the rate of gully erosion: runoff-producing characteristics of the watershed; drainage area; soil characteristics; the alignment, size, and shape of the gully; and slope in the channel Gully Erosion Processes involve in gully development: 1. waterfall erosion or headcutting at the gully head, 2. erosion caused by water flowing through the gully or by raindrop splash on exposed gully sides, 3. alternate freezing and thawing of the exposed soil banks, and 4. slides or mass movement (sloughing) of soil into the gully. Stream Channel Erosion - applies to the lower end of headwater tributaries and to streams that have nearly continuous flow and relatively flat gradients, - includes soil removal from stream banks and soil scour of the channel bed. - stream banks erode either by runoff flowing over the side of the stream bank, or by scouring and undercutting below the water surface. Stream Channel Erosion - Stream bank erosion, less serious than scour erosion, is often increased by the removal of vegetation, overgrazing, tilling too near the banks, or straightening the channel. - Scour erosion is influenced by the velocity and direction of flow, depth and width of the channel, and soil texture. - Bank erosion can also lead to stream meandering and rechannelization, resulting in major erosion and deposition within the floodplain. At the same time, stream meandering can increase undercutting of banks, and bank erosion, as these two processes tend to complement each other in active channels. Soil Erosion Control Vegetative Measure ▪ Reforestation – replanting of forest trees species in the watershed area ▪ Agroforestry – a practical adaptation of reforestation whereby the species planted have economic value, such as mango, pili and so on. ▪ Strip cropping – is the practice of growing different crops in alternate strips across the slope to serve as barriers for soil erosion. Soil Erosion Control Vegetative Measure ▪ Mulching – the process of covering the land surface with plant residues, plastic or other materials appropriate to arrest loss of moisture through evaporation ▪ Contour cultivation – consists of carrying out agricultural operations very nearly on the contour. It reduces the velocity of overland flow and retards soil erosion ▪ Cropping systems –modification of copping system such as crop rotation and mixed cropping Soil Erosion Control Engineering Measure Terracing – construction of earth embankment or ridge and channel across the slope at an acceptable grade to control the flow of runoff as well as soil particles. Grassed Waterways – establishment of natural waterways or construction of canals and planting it with grasses to make it stable and arrest soil erosion Check dams or weirs – constructed along the channel or waterway to control the velocity of flowing water and encourage deposition of sediments carried by water Farm Ponds / Water Impounding Dams – temporary detainment of water in farm pond and dams to mitigate the erosive capacity of water Soil Erosion Control Diversion Canal – a channel constructed around the slope and given a slight gradient to cause water to flow to a suitable and stable outlet. Gabions – similar to stone check dams however the stones in this case are placed on rectangular wire mesh, piled-up as blocks and tied together to form a reinforced wall or dam structure. Riprap – concrete structure made of stones constructed along steep embankments to prevent landslide or gully erosion Stone wall – made of stones carefully and properly piled-up and arranged on steep embankments to protect from gully erosion or landslide Gully erosion and control Gully erosion usually starts as small rills and gradually develops into deeper crevices. The rate of gully formation depends on the amount of runoff, slope and the soil characteristics. Control measures: Grassed waterways – is constructed across the slope above the gully. The grassed waterway intercepts the runoff coming from above the gully. Grassed waterways are open channels protected with suitable grasses constructed along the slope and act as outlet for terraces and graded bunds. They are also used to safely convey runoff from contour furrows, diversion channels and serve as emergency spillways in farm ponds. The velocity of flow through the grassed waterways is dependent upon the ability of the vegetation to resist erosion. For design purposes, an average of 1.5 m/s to 2 m/s is used. Vegetation – can stabilize the slopes of the gully and hence reduce runoff velocities. Chute spillways, drops, and drop spillways are permanent structures that can reduce the energy of the runoff water. Soil Erosion Control Terracing Functions: Reduce sheet and rill erosion Prevent gully formation Moisture conservation Types of Terraces 1. Bench - construction of series of platforms along the contours cut into the hill slope in a step like formation used for 25-30% slopes used for maximum moisture conservation used where land is at a premium conventional difficult to farm 2.Zingg or Conservation bench terrace used for 9-24% slope easier to farm than conventional used for maximum soil and water conservation 3. Broad-based A. Graded or channel type primary purpose is to remove excess water in such a way as to minimize erosion reduce slope length, conducts intercepted runoff water to outlet at non-erosive velocity outlet either surface or subsurface type B. Level or ridge type no grade in channel primary purpose is moisture conservation, erosion control is secondary channel is normally closed at both ends to assure maximum detention adopted to deep, permeable soils more formable than bench types used where outlets are a problem Terrace Design Vertical Interval (VI)= aS + b (for graded terraces) (VI)= aS + 0.85b (for level terraces) Horizontal Interval (HI) = (VI x 100)/S = a(100) + b (100)/S Where: a = constant for geographic location (value range from 0.3 – 0.8 depending on the intensity of rainfall) S = average slope above the terrace in percent b = constant for soil erodibility and cover conditions b = 1 erodible soil and poor cover b = 2 resistant soil and good cover Vanoni, V. A. 2006. Sedimentation Engineering. ASCE Manuals and Reports on Engineering Practice No. 54. American Society of Civil Engineers Question With an understanding of soil erosion, how would you define sediment transport? Sediment Transport Sediment Transport is the movement of these detached soil particles (sediment) from one location to another, typically by water (streams, rivers) or wind. After the soil particles are eroded, they are carried away by these forces and can be deposited elsewhere. Sedimentation is the process of allowing particles in suspension in water to settle out of the suspension under the effect of gravity. Vanoni, V. A. 2006. Sedimentation Engineering. ASCE Manuals and Reports on Engineering Practice No. 54. American Society of Civil Engineers Mechanics of sediment transport Soil and Water Conservation Engineering Soil Web. SoilWeb/Soil Management/Soil Erosion; The University of British Columbia: 2003. Available online: http://wiki. ubc.ca/LFS:SoilWeb/Soil_Management/Soil_Erosion (accessed on 23 May 2016) Sediment Transport Sediment Transport is the combination of Bed movement (sliding and rolling) Saltation (bouncing/impact of particle) Suspension (due to the velocity of flow) Total Sediment Transport = bed load + suspended load Sediment discharge = rate of transport of sediment Vanoni, V. A. 2006. Sedimentation Engineering. ASCE Manuals and Reports on Engineering Practice No. 54. American Society of Civil Engineers Transported Materials/ Sediments Definition: Transported materials, often referred to as sediments, are particles of soil, sand, silt, clay, and organic matter that are carried by water (runoff) or wind from one location to another. Sediment transport refers to the movement of solid particles, typically soil, sand, silt, or gravel, by water, wind, or ice. In water bodies like rivers and streams, sediments are eroded from the riverbed or surrounding land and transported downstream. Classification of Sediment Load Bed Load: This refers to larger, coarser sediments (like gravel or cobbles) that roll or slide along the bed without being lifted much. - rolling/sliding along the bottom Suspended Load: This consists of fine particles (like silt and clay) that are carried in suspension, kept aloft by the turbulence of the fluid flow. - particles remain suspended in the flow Saltation Load: Falls between bed load and suspended load in terms of particle size and energy. -Mode of sediment transport: bouncing or hopping Factors Influencing Sediment Transport: Channel Morphology: Flow velocity: Higher flow velocities can The shape and slope of the transport larger and river or streambed influence more sediment. sediment transport dynamics. Water depth: Particle size: Larger, Shallow water tends Turbulence: More heavier particles to transport less turbulent water settle faster than sediment compared suspends more fine smaller, lighter to deeper, faster- particles. ones. flowing water. Vertical Distribution of Sediment Flow Concept: The vertical distribution of sediment flow refers to how sediments are distributed at different depths in a water column. This distribution is largely governed by particle size, water velocity, and turbulence. The concentration of sediments varies from the bed to the surface of the water body. Sediment concentration in water bodies varies with depth. Bedload: Predominantly moves near the Sediment bottom of the stream or river. Stratification: Suspended load: Spread throughout the water column but tends to be higher in concentration near the bottom where turbulence is greater. Sediment Transport Mechanisms: Shear stress: The force exerted by moving water on the sediment, causing transport. Settling velocity: The rate at which particles fall through the water column. Larger particles settle more quickly, while finer particles remain in suspension. Entrainment and deposition: Entrainment occurs when particles are lifted from the bed into the flow, while deposition is when they settle out of suspension. Distribution Models: Rouse (1937) Profile: A widely used model to describe the vertical distribution of suspended sediments. It predicts that finer particles remain suspended in the upper water column, while larger particles settle closer to the bed. The first model to simulate convincingly the vertical distribution of dilute suspended sediment in steady flow over a sediment bed, without net deposition, i.e., the profile. Measurement Techniques: Measurement/ Prediction of 1. Direct Sampling: Sediment Transport and Suspended Load Samplers: Devices like Sediment depth-integrating samplers or point- integrating samplers are used to collect Yields suspended sediment at various depths. Bed Load Samplers: These are specialized devices placed on the bed of a stream or river to collect sediments that are being transported along the bottom (e.g., Helley-Smith sampler). Measurement Techniques: Measurement/ Prediction of 2. Turbidity Sensors: Sediment Transport and Sediment Yields o These sensors measure the cloudiness (turbidity) of the water, which can be correlated with the concentration of suspended sediments. Measurement Techniques: Measurement/ Prediction of Sediment 3. Acoustic Doppler Current Profilers (ADCP): Transport and Sediment This instrument measures the Yields velocity of water and can be used to estimate sediment transport indirectly by correlating velocity with sediment concentration. Measurement Techniques: Measurement/ Prediction of Sediment 4. Optical and Laser Sensors: Transport and Sediment Yields o Devices like optical backscatter sensors (OBS) measure light scattering caused by suspended particles, allowing real-time sediment concentration measurement. Measurement Techniques: Measurement/ Prediction of Sediment 5. Gravel Traps: Transport and Sediment Yields o For bed load measurement, gravel traps can be installed to collect transported sediment particles in controlled environments like rivers. Prediction Methods: Empirical Equations: Sediment Transport Models: Numerical Models: Einstein-Brown Formula: This Hjulström Curve: Shows the HEC-RAS (Hydrologic is used to estimate bed load relationship between particle Engineering Center’s River transport based on flow size and velocity required for Analysis System): This model parameters, sediment size, erosion, transport, and simulates sediment transport and density. deposition. by integrating hydrologic and Meyer-Peter Müller Equation: Shields Diagram: Used to hydraulic models to estimate A classical formula to estimate determine the initiation of sediment erosion, transport, bed load transport rates in sediment movement based on and deposition. rivers. flow conditions and particle SWAT (Soil and Water size. Assessment Tool): A watershed-scale model used to predict sediment yields and evaluate the impact of land management practices on sediment movement. Sediment Yield: Definition: The total sediment outflow from a watershed or drainage basin, measurable at a cross-section of reference and in a specified period of time. Commonly expressed in units of weight (tons), volume (acre-feet or cubic meters), or uniformly eroded depth of soil (inches or millimeters). Another useful measure of sediment outflow, the solids concentration of runoff, is parts per million (ppm) by weight or by such metric counterparts as grams per cubic meter and milligrams per liter. Factors Affecting Sediment Yield: o Watershed characteristics: Size, shape, slope, and land cover all influence sediment yield. o Rainfall intensity: Heavier rainfall leads to higher erosion and sediment transport. o Land use: Deforestation, agriculture, and urbanization can increase sediment yields due to increased erosion. Methods of Estimating Sediment Yield: o Sediment Rating Curves: Developed by plotting sediment discharge against water discharge at a monitoring point. o USLE (Universal Soil Loss Equation): Predicts soil erosion and sediment yield based on rainfall, soil type, topography, and land use. Variants: Modified USLE (MUSLE): Incorporates additional factors such as sediment yield from surface runoff events. Revised USLE (RUSLE): An updated version of USLE with improvements for various terrain types and land use patterns. END