Chapter Basics Principles of CT PDF
Document Details
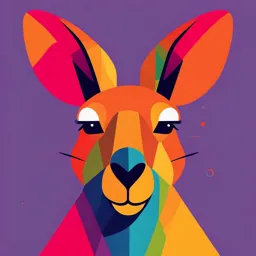
Uploaded by EasierPeninsula
Galala University
Tags
Summary
This document provides a basic overview of computed tomography (CT), explaining how it works and its advantages over conventional radiography. It describes the principles, terminology, image quality, and beam attenuation in CT.
Full Transcript
Chapter Basics Principles of CT Conventional radiographs depict a three-dimensional object as a two-dimensional image. This results in overlying. tissues being superimposed on the image, a major limitation of conventional radiography....
Chapter Basics Principles of CT Conventional radiographs depict a three-dimensional object as a two-dimensional image. This results in overlying. tissues being superimposed on the image, a major limitation of conventional radiography. Computed tomography (CT) overcomes this problem by scanning thin sections of the body with a narrow X-ray beam that rotates around. the body, producing images of each cross-section. nother limitation of the conventional radiograph is its A inability. to distinguish between two tissues with similar densities. The unique physics of CT allows for the differentiation between tissues of similar densities. The main advantages of CT over conventional radiography are the elimination of superimposed structures, the ability to differentiate small differences in density of anatomic structures and abnormalities, and the superior quality of the images. TERMINOLOGY he word tomography has as its root tomo, T meaning to cut, section, or layer from the Greek tomos (a cutting). In the case of CT, a sophisticated computerized method is used to obtain data and transform them into "cuts," or cross-sectional slices of the human body. common to refer to older scanning systems as computerized axial tomography, hence the common acronym, CAT scan, The preliminary image each scanner produces may be referred to as a "topogram" (Siemens), "scout" (GE Healthcare), or "scanogram" (Toshiba). Another well-known example is a method of scanning that, generically, is referred to as continuous acquisition scanning; this method can also be called "spiral" (Siemens), "helical" (GE Healthcare), or "isotropic" (Toshiba) scanning. CT image quality T image quality is typically evaluated using several C criteria: Spatial resolution describes the ability of a system to define small objects' positions distinctly. Low-contrast resolution refers to the ability of a system to differentiate, on the image, objects with similar densities. Temporal resolution refers to the speed at which the data can be acquired. This speed is particularly important to reduce or eliminate artifacts that result from object motion, such as those commonly seen when imaging the heart Each CT slice represents a specific plane in the patient's body. The thickness of the plane is referred to as the Z- axis. The Z-axis determines the thickness of the slices. Selecting a slice thickness limits the X-ray beam so that it passes only through this volume: hence, scatter radiation and superimposition of other structures are greatly diminished. Limiting the x-ray beam in this manner is accomplished by mechanical hardware that resembles small. shutters, called collimators, which adjust the opening based on the operator's selection. he data that form the CT slice are further sectioned into elements: T width is indicated by X, while height is indicated by Y. Each one of these two-dimensional squares is a pixel (picture element). A composite of thousands. of pixels creates the CT image that is displayed on the CT monitor. If the Z axis is considered, the result is a cube, rather than a square. This cube is referred to as a voxel (volume element). A matrix is a grid formed from the rows and columns of pixels. In CT, the most common matrix size is 512. This size translates to 512 rows of pixels down and 512 columns of pixels across. The total number of pixels in a matrix is the product of the number of rows and the number of columns, in this case 512x512. Each pixel contains information that the system obtains from scanning. BEAM ATTENUATION n X-ray beam consists of bundles of energy known as photons. These A photons may pass through or be redirected (i.e., scattered) by a structure. A third option is that the photons may be absorbed by a given structure in varying amounts, depending on the strength (average photon energy) of the X-ray beam and the characteristics of the structure in its path. The degree to which a beam is reduced is a phenomenon referred to as attenuation. In conventional film-screen radiography, the X-ray beam passes through the patient's body and exposes the photographic film. Similarly, in CT, the X-ray beam passes through the patient's body and is recorded by the detectors. The computer then processes this information to create the CT image. In both cases, the quantities of X- ray photons that pass through the body determine the shades of gray on the image y convention, x-ray photons that pass-through objects unimpeded are B represented by a black area on the image. These areas on the image are commonly referred to as having low attenuation. Conversely, an X-ray beam that is completely absorbed by an object cannot be detected; the place on the image is white. An object that can absorb much of the X-ray beam is often referred to as having high attenuation. Areas of intermediate attenuations are represented by various shades of gray. The number of photons that interact depends on the thickness, density, and atomic number of the object. Density can be defined as the mass of a substance per unit volume. More simply, density is the degree to which matter is crowded together or concentrated. For example, a tightly packed snowball has a higher density than a loosely packed one. Dense elements, those with a high atomic number, have many circulating electrons and heavy nuclei and, therefore, provide more opportunities for photon interaction than elements of less density. To better understand how these physical properties of an object affect the degree of beam attenuation, envision a single X-ray photon passing through an object. The more atoms in its path (the greater the object's thickness and density), the more likely that an atom in the object will interact with the photon. Similarly, the more electrons, neutrons, and protons in each atom, the higher the likelihood of photon interaction. Therefore, the number of photons that interact increases with the density, thickness, and atomic number of the object. The amount of the x-ray beam that is scattered or absorbed per unit thickness of the absorber is expressed by the linear attenuation coefficient, represented by the Greek letter µ. For example, if a 125- kVp x-ray beam is used, the linearI attenuation coefficient for water is approximately 0.18 cm (the unit cm indicates per centimeter). This means that about 18% of the photons are either absorbed or scattered when the X-ray beam passes through 1 em of water. In general, the attenuation coefficient decreases with increasing photon energy and increases with increasing atomic number and density. It follows that if the kVp is kept constant, the linear attenuation coefficient will be higher for bone than it would be for lung tissue. This corresponds with what we commonly see in practice. That is, bone attenuates more of the x-ray beam than does lung, allowing fewer photons to reach the CT detectors. Ultimately, this results in an image in which bone is represented by a lighter shade of gray than that representing lung. Differences in linear attenuation coefficients among tissues are responsible for x-ray image contrast. In CT, the image is a direct reflection of the distribution of linear attenuation coefficients. For soft tissues, the linear attenuation coefficient is roughly proportional to physical denisty For this reason, the values in a CT image are sometimes referred to as density. Metals are generally quite dense and have the greatest capacity for beam attenuation. Consequently, surgical clips and other metallic objects are represented on the CT image as white areas. Air (gas) has a very low density, so it has little attenuation capacity. Air-filled structures (such as lungs) are represented on the CT image as black areas. To differentiate an object on a CT image from adjacent objects, there must be a density difference between the two objects. An oral or intravenous administration of a contrast agent is often used to create a temporary artificial density difference between objects. Contrast agents fill a structure with a material that has a different density than that of the structure. In the cases of agents that contain barium sulfate and iodine, the material is of a higher density than the structure. These are typically referred to as positive agents Low-density contrast agents, or negative agents, such as water, can also be used. A figure shows an image taken at the level of the kidneys without contrast enhancement. A figure shows the same slice after the intravenous injection of an iodinated contrast agent. The kidneys and blood vessels are highlighted because of the high-density contrast they contain. These agents simply fill the structures with a higher density fluid so that their margins can be visualized on the CT image (analogy a glass of water either clear or color). We have to learn that a contrast agent does not permanently change the physical properties of the structure containing it. HOUNSFIELD UNITS In CT, we are better able to quantify the beam attenuation capability of a given object. Measurements are expressed in Hounsfield units (HU), named after Godfrey Hounsfield, one of the pioneers in the development of CT. These units are also referred to as CT numbers or density values. Hounsfield units quantify the degree to which a structure*.attenuates an X-ray beam Hounsfield arbitrarily assigned distilled water the number 0. He assigned the number 1000 to dense bone and - 1000 to air. Objects with a beam attenuation less than that of water have an associated negative number. Conversely, substances with an attenuation greater than that of water have a proportionally positive Hounsfield value. The Hounsfield unit of naturally occurring anatomic structures falls within this range of 1000 to 1000. The Hounsfield unit value is directly related to the linear attenuation coefficient: 1 HU equals a 0.1% difference between the linear attenuation coefficient of the tissue compared with the linear attenuation coefficient of water. Using the system of Hounsfield units, a measurement of an unknown structure that appears on an image is taken and compared with measurements of known structures It is then possible to approximate the composition of the unknown structure. For example, a slice of the abdomen shows a circular low- attenuation (dark) area on the left kidney. By taking a density reading of this area, it is discovered to measure 4 HU. It can then be assumed that it is fluid-filled (most likely a cyst). It is important to keep in mind that the reading for the assumed cyst is not exact. In this example, it is suspected that the mass is fluid because its measurement is close to that of pure water. The difference of 4 units could be caused by impurities in the cyst; it is not likely that a cyst would consist of pure water. Factors that contribute to an inaccurate Hounsfield measurement include poor equipment calibration,.image artifacts, and volume averaging POLYCHROMATIC X RAY BEAMS All X-ray beam sources for CT and conventional radiography produce polychromatic x-ray energy. That is, the X- ray beam comprises photons with varying energies. The spectrum ranges from weak X-ray photons to others that are relatively strong. It is important to understand how this basic property affects the image. Low- energy X-ray photons are more readily attenuated by the patient. The detectors cannot differentiate and adjust for differences in attenuation that are caused by low-energy X-ray photons. To the detectors, any x-ray photon that reaches the detector is treated identically, whether it.began with high or low energy This phenomenon can produce artifacts, Artifacts are objects seen in the image but not present in the object scanned. Artifacts always degrade the image quality. Artifacts that result from preferential absorption of the low energy photons, which leaves higher-intensity photons to strike the detector array, are called beam-hardening artifacts. This effect is most obvious when the X-ray beam must first penetrate a dense structure, such as the base of the skull. Beam-hardening artifacts appear as dark streaks or vague areas of decreased density, sometimes called cupping artifacts. Filtering the X-ray beam with a substance, such as Teflon or aluminum, helps to reduce the range of X- ray energies that reach the patient by eliminating the photons with weaker energies. It makes the X-ray beam more homogeneous. Creating a more uniform beam intensity improves the CT image by reducing artifacts. Additionally, filtering the soft (low energy) photons reduces the radiation dose to the patient.