Textbook of Production Technology PDF
Document Details
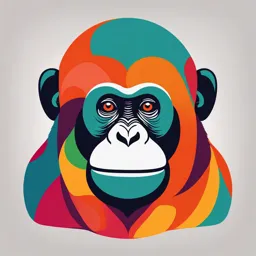
Uploaded by ImaginativePeachTree
STC/JHS
Tags
Summary
This document discusses various aspects of production technology, focusing on unconventional manufacturing methods like electromagnetic forming and high-velocity forming. It covers different machines and their characteristics.
Full Transcript
590 A Textbook of Production Technology 4. Only relatively small components can be made. 9.8.3. Electro-Magnetic Forming. Electro-magnetic forming, also called magnetic pulse forming, is based on the fundamental principles of...
590 A Textbook of Production Technology 4. Only relatively small components can be made. 9.8.3. Electro-Magnetic Forming. Electro-magnetic forming, also called magnetic pulse forming, is based on the fundamental principles of electrical technology viz., when a current flows in a conductor, a magnetic field is set up around it. If the current and hence the magnetic field changes, a current is induced in any other conductor placed in the magnetic field. The direction of this induced current is such that the induced magnetic field opposes the magnetic field producing it. The two conductors get repelled due to the interaction of the two magnetic fields. In electro- magnetic forming, one conductor is a coil, through which current is suddenly discharged, giving rise to a rapidly changing magnetic field. The work-piece is the second conductor, in which the eddy current are set up. The repelling forces between the coil and the work-piece are high (of the order of 350 N/mm2 for several micro/seconds), and since the coil is held rigidly in place, the work-piece is repelled and forced against a die. Fig.9.18 shows the basic circuit for electro-magnetic forming. The energy source is a bank of capacitors, charged to a predetermined voltage. The electric energy is discharged through a forming coil. By suitably designing the coil, the process can be used for compression, expansion or for forming contours from sheet metal and tubes. For example, for compressing a tube, the coil is wound around the tube which is contracted onto a mandrel and for expanding, it is inserted inside of a tube surrounded by die. The product applications of this process are : it works effectively on relatively thin materials and is particularly suitable for ; bulging of tubes, shrinking of tubes, flaring and swaging tubes over rods etc. The work-piece must be an electrical conductor, but it need not be magnetic. In this process, since the pulse duration is very short, sufficient pressures are not generated for forming thick material; so, its use is only limited to sheet metal working. Work Piece Power Supply C Coil Fig. 9.18. Electro-Magnetic Forming. To minimise the magnetic cushion effect, the die materials should be of low electrical conductivity. Due to this, the dies are made usually of steel or epoxy resin. To ensure good reproduction of detail and prevent distortion, air is evacuated from the die. Steel dies have longer life than resin dies but with these, the cushion effect is not entirely prevented. Electro-magnetic forming has got the following characteristics : 1. The process is simple, clean and safe to operate. The operating costs are low. A high cycle rate can be attained and the process is suitable for automation. 2. Deep drawing is not possible, because of the very short duration of the pressure pulse. 3. Asymmetrical parts are not suitable for this method. 4. Irregularities such as slots, holes and slits present problems, because the magnetic field is disrupted. Unconventional Manufacturing Methods 591 9.8.4. High Velocity Forming. The HERF methods discussed above are used for the deformation of metals in the form of flat sheet or thin walled tubes. So, these methods are sheet metal forming methods. when the metal is in the form of solid billets, HVF methods (Called Billet forming methods) are used. The conventional sheet metal forming machines are mainly : Crank and hydraulic presses. In high speed sheet metal forming (HERF) machines, there are no moving parts at all. The energy for metal formation is derived solely from the shock wave from the source. The conventional billet forming machines are usually :- Hydraulic presses, crank and eccentric presses, screw presses, drop hammers and power hammers. Their comparison with high speed billet forming machines is : (i) Crank or Hydraulic Press. Large force, low kinetic energy and large mass of the ram. (ii) Drop Hammer. Zero force, large kinetic energy and fairly large mass of ram. (iii) High Speed Machine. Small force, large kinetic energy and small mass of ram. The speeds of movement of the deforming material in conventional and high speed machines is compared below : (1) Conventional Machines (a) Hydraulic press : Upto 0.3 m/s (b) Crank press : Upto 1.5 m/s (c) Drop hammer : Upto 4.5 m/s. (2) High Speed Machines (a) Billet forming machines : 6 to 24 m/s (b) Sheet forming machines : 30 to 300 m/s. Comparison of Conventional and High Speed Billet Forming Machines (1) Impact Speed. The speed of impact of the ram or punch with the work-piece is the most obvious difference between conventional and high speed machines. However, this difference is only significant in the early stages of forming, since, the ram speed is always zero at the end of the forming operation, regardless of the type of the machine used. Below is given the impact speeds of the various metal forming machines : (i) Hydraulic press : 0.03 to 0.3 m/s (ii) Crank press : 0.3 to 1.5 m/s (iii) Screw press : 0.3 to 1.5 m/s (iv) Drop hammer : 3 to 4.5 m/s (v) Power hammer : 4.5 to 9 m/s (vi) High speed machine : 6 to 24 m/s Fig. 9.19. shows the variation of ram speed with ram stroke for the various machines. Full lines denote the speeds set by machines and dotted lines denote the speeds set up by the resistance of the work-piece. In a hydraulic press, the ram speed is virtually independent of the resistance of the work-piece, provided the press capacity is big enough. At the end of the stroke, the ram speed falls to zero, usually as a result of opening of the relief valve. Ram Speed Ram Stroke (a) Hydraulic Press (b) Crank Press (c) Friction screw (d ) Drop Hammer high Speed Press Machine Fig. 9.19. Variation of Ram Speed with Ram Stroke. 592 A Textbook of Production Technology In a crank or eccentric press, the ram speed depends upon the geometry of the crank and connecting rod. It decreases gradually as the ram advances towards the end of its downward stroke. Thus the ram speed is not much influenced by the resistance of the work-piece. For screw press, the design of the driving mechanism and the resistance of the work-piece both affect the ram speed. In drop hammer, power hammer and high speed machines, the ram speed before impact depends upon the design of the drive mechanism, but after impact, it will be determined by the resistance of the work-piece. While setting up the hydraulic and crank presses, the stroke is adjusted to suit the particular work-piece. These machines are essentially constant stroke devices, their capacities being determined by the resistance of the work-piece. For hammers and high speed machines, the energy level is pre- set by the operator and the length of the stroke is largely determined by the resistance to deformation of the work-piece. (2) Energy Available for Forming. The energy supplied by the machine may either be pressure energy or kinetic energy. Pressure energy can only be supplied when the ram experiences resistance, that is, during the forming operation, whereas, the kinetic energy is supplied before the forming operation begins. The pressure energy is supplied directly from the power source, whereas, the kinetic energy is invested into the whole moving ram or platen and is absorbed by the work- piece. The proportion of pressure energy and kinetic energy in various forming machines is given in Table 9.1. Table 9.1 Machine Percentage of total energy available Pressure energy Kinetic energy Hydraulic Press 100 0 Crank Press 90 10 Screw Press 60 40 Power Hammer 5 95 Drop Hammer 0 100 High Speed machine 5 95 It is clear from this table that the high speed machine is more closely allied to the power hammer and so is a logical development of the hammer. As has already been discussed (chapter 4), the hydraulic, crank and screw presses are rated in terms of the maximum force which can be exerted on the work- piece. High speed machines and hammers are rated in terms of the maximum energy which can be transferred to the work-piece. The practice of rating a hammer by the weight of the moving ram or tup is only a very approximate guide about the capacity of the hammer to do work and, therefore, is not recommended. High Speed Forming Machines. High speed billet forming machines were first introduced in the midÄ1950's, in U.S.A. All these machines operate on the principle of the sudden release of stored energy. The most common method of storing energy is compressed gas. The sudden expansion of the gas accelerates the mass (ram or tup). Use is also made of the energy stored chemically in the form of petrol. Electrical energy can also be used to accelerate the mass directly. The mass is accelerated to its impact speed in about 20 to 30 milliseconds. This constitutes about 1 per cent of the total cycle time for the machine. The energy is usually generated over the whole of the cycle, and stored until required. By this means, a comparatively small power source can be used. Below, we discuss some of the high speed forming machines : 1. Petro - Forging or Petro - Forge Forming. In this process, the stored chemical energy of a hydrocarbon, like petrol or diesel is utilized to move the dies at very high velocity. The principle of working of a Petro-forge hammer is just similar to I.C. engine. Fig. 9.20 illustrates the working Unconventional Manufacturing Methods 593 of a petro-forge hammer. It is a piston-cylinder arrangement and a piston drives a ram (piston rod) and a die. At the beginning of a cycle, the piston is raised upward by admitting a low pressure air at the bottom of the cylinder. A projection is provided at the centre of the piston and when it enters a hole in the cover of the cylinder (during the upward stroke of the piston), it seals the combustion chamber. The seal area is 1/6 to 1/10 of area of the bottom of piston. Due to this the back pressure can support 6 to 10 times the pressure in the combustion chamber. The air-fuel mixture is now ignited in the combustion chamber. The pressure increases by 5 to 7 times which breaks the seal and the high pressure gases act on the top face of the piston. The piston, ram and die are accelerated at a very rapid rate. This is the working stroke. At the end of the working stroke, the piston is again raised upwards. The exhaust valve is opened simultaneously for the escape of exhaust gases from the combustion chamber. Spark Fuel Combustion Plug Injector Chamber Exhaust Inlet Valve Valve Piston Seal Cylinder Low Pressure Air Ram Die (a) (b) (c) (d) Fig. 9.20. Petro-forge Hammer. 2. Dynapak. In this process, the Vent required energy for driving the piston, ram Seal H.P. Gas Cylinder and die is obtained by releasing instantly high Supporting pressure gas from storage and expanding it Structure above the piston. The return of the piston to Ram Frame Hydraulic the top of the cylinder is achieved by Jacks hydraulic jacks. This process is commonly Hydraulic used for : forging, powder compacting and Fluid Pneumatic sheet metal working etc. Bellows The working of a Dynapak machine (b) (a) (manufactured by General Dynamics L.P. Gas Corporation U.S.A.) is illustrated in Fig. 9.21. The main parts of the machine are : (1) The integral piston and ram which is an alloy steel forging (2) Cylinder (3) Face seal (4) Vent (5) Frame (Single alloy steel forging) (6) Pneumatic bellows which support the frame (7) Hydraulic jacks (8) Supporting structure (welded steel), which guides the small movement of the frame. The working medium (c) (d) is air or gas (preferred). Fig. 9.21. Dynapak Machine. Position (a) it is the ready-to-fire position. The piston is held against the seal and it is in static balance. The upward force due to gas pressure acting on the shoulder of the piston will be equal to the down-ward force due to gas acting on the very small area outside the seal. 594 A Textbook of Production Technology Position (b) a small surge of gas is admitted through the vent into the volume inside the seal. This disturbs the static equilibrium of piston which then moves away from the seal. Now, the gas in the main cylinder acts over the full top face of the piston. This downward force will be much greater than the upward force acting on the piston shoulder. The piston and ram are then accelerated rapidly downward, the pressure energy of gas being converted to the kinetic energy of the ram. Position (c) The ram strikes the work-piece by impact. Since the same is supported on bellows, it moves upwards as the ram moves downwards. Since action and reaction are equal and the frame is very heavy, its upwards movement will be very small as compared to the piston stroke. In due course, the frame returns to its original position under gravity. Since the frame is supported on bellows, very little thrust is transmitted to the ground. So, the foundations are needed only to support the structure. Position (d) The hydraulic jacks lift the piston until it seats against the seal. The small amount of gas trapped by the seal is vented to the atmosphere. The jacks are withdrawn and the machine is ready for next cycle. A Dynapak machine has the following specifications : Maximum energy output : 11 to 310 kNm Piston diameter : 76 mm to 305 mm. Maximum impact speed : 18 m/s Cycles per minute : 20 for the smallest machine to 7 for the largest machine. High Velocity processes. Hot forging of steel has received the maximum attention under HVF. One of the main advantages of HVF is that the job is completed in a single blow. Also, very short time is available for heat to be lost to the dies. Thus, the forging temperatures are maintained throughout the operation. As a result, HVF is particularly advantageous for the forging of components having thin webs and intricate contours and made of high strength materials. If possible, closed dies should be used for high speed hot forging, since the effectiveness of a flash land is reduced under high speed condition. Other processes under HVF are : extrusion, powder compaction, cold forging, bar drawing, hydrostatic extrusion, blanking and bar cropping. HVF is employed for the production of such items as expanded tubes, ducts, drawing tubes, forming of sheet products, extruding and forging of steel, piercing of holes and the compression of metal and ceramic powders into shapes. HVF is not a mass production process. 9.9. SOME OTHER NON-TRADITIONAL MACHINING METHODS (NTMM) We have noted in the beginning of the chapter that NTMM have been developed to machine workpiece materials of increased strength and hardness. The other factors behind their development are : 1. Complexity of the die-cavity shape to meet the higher productivity demands, and 2. Need for attaining higher accuracy combined with automation. Out of the NTMM discussed, the following methods have not been proved economic, commercially : USM, CHM, EBM and PAM. The other NTMM in this category are : Abrasive Jet Machining (AJM), Water Jet Machining (WJM) and Ion Beam Machining (IBM). In AJM, high velocity abrasive particles are used to do machining. In WJM, high velocity hydraulic fluid is used. In both these methods, the machining is achieved by erosion of the work material. In IBM, the source of energy is electric voltage and the transfer medium is ionised material. Below, we discuss in short, AJM, IBM, and WJM. 9.9.1.Abrasive Jet Machining (AJM). In abrasive jet machining method, the material is removed from the surface of a workpiece, by impinging a focussed jet of fine abrasive particles carried by a compressed gas which imparts kinetic energy to the stream of fine abrasives. The stream leaves through a nozzle at a velocity of the order of 300 m/s and strikes the surface of the workpiece, producing impact loading on it. Severe plastic deformation or micro-cracks occur in the vicinity of the impact. Due to repeated impacts, small chips of material get loosened and a fresh Unconventional Manufacturing Methods 595 surface gets exposed to the jet. The carrier gas can be air, nitrogen or carbon dioxide, but never oxygen. The air must be filtered to remove water, oil and other contaminants. Nozzle pressure can range between 0.20 N/ mm2 and 0.85 N/mm2 but is usually about 0.5 N/mm2 Higher pressure results in rapid nozzle wear, low pressure gives slow metal removal rates. To resist abrasion and wear of nozzles, these are made of hard materials such as tungsten carbide and synthetic sapphire. The useful life of sapphire nozzles is about 10 times that of tungsten carbide nozzles. The sizes of the nozzles are usually : Tungsten Carbide Round : 0.0125 to 0.80 mm diameter Rectangular : 0.075 mm × 0.50 mm to : 0.175 mm × 3.75 mm Square : 0.65 mm square Sapphire nozzle are made only round : 0.20 to 0.65 mm diameter The various abrasive materials used in AJM include : aluminium oxide, silicon carbide, glass powder or beads, dolomite (calcium magnesium carbonate) and specially prepared sodium bicarbonate. When sodium bicarbonate is used, it must be kept dry. The cutting performance will depend upon : hardness, strength, particle size and particle shape of the abrasive. Al2O3 is a general purpose abrasive and is used in sizes of 10, 25 and 50 microns. SiC is used for faster cutting on extremely hard materials. It is used in 25 and 50 microns sizes. Dolomite is used in 200 mesh size for light cleaning and etching. Sodium bicarbonate is used for extra fine cleaning and glass beads (0.635 to 1.27 mm diameter) are used for light polishing and fine deburring. In general, larger sizes are used for rapid removal rate while smaller sizes are used for good surface finish and precision. The metal removal rate will depend upon : the diameter of nozzle, composition of abrasive gas mixture, hardness of abrasive particles and that of the work-material, particle size, velocity of jet and distance of workpiece from the jet. The typical metal removal rates vary from 0.016 to 0.02 cm3/min. Pressurised Air Filter Air + Abrasive Pressure Pressure Valve Vibrating Gauge Gauge Mixing Opening Chamber Valve Abrasive Compressor Powder Nozzle Drain Pressure Regulator (a) High Velocity Gas + Abrasive particles Nozzle Tip Nozzle Tip Distance (NTD) Abrasive and 0.81 mm (Typically) Work Particles Workpiece (b) Fig. 9.22. Schematic Diagram of AJM. 596 A Textbook of Production Technology Masks defining the machining area are sometimes used to prevent stray cutting. Copper is a good all purpose masking material. Glass gives excellent definition but has short life. Rubber has long life but gives poor definition. Applications of AJM include : abrasive, cutting, or cleaning (for example electrical contacts) and for machining semi-conductors such as silicon, gallium or germanium, for making holes and slots in glass, quartz, sapphire, mica and ceramics. Other applications are : cleaning hard surfaces, deburring, scribing, grooving, polishing and radiusing. A dimensional tolerance of 0.05 mm can be obtained. Surface finish obtained is in the range of 0.5 to 1.2 μ m CLA. Typical power input is 0.25 kW. The method is used chiefly to cut materials that are sensitive to heat damage and thin section of hard materials that chip easily and to cut indicate holes that would be more difficult to produce by other methods. The other advantage is its low capital cost. The disadvantages of the method are : low metal removal rate, possibility of stray cutting, embedding of abrasive particles in soft workpiece and dust control requirements. A schematic diagram of AJM is shown in Fig. 9.22. Dry and filtered gas (in this case air) is raised to a high pressure in the compressor. The pressurised air flows to the vibrating mixing chamber containing abrasive powder. The mixture of pressurised air and abrasive powder than flows to the nozzle. It then impinges on the workpiece after shooting out of the nozzle exit. The pressure regulator regulates the gas flow and its pressure. The feed rate of abrasive powder is controlled by amplitude or vibrations of the mixing chamber. The movement of the nozzle towards the workpiece or vice versa is controlled by a cam mechanism or a pantograph mechanism. 9.9.2. Water Jet Machining (WJM). Water jets alone (without abrasive) can be used for cutting. Thin jets of high pressure and high velocity have been used to cut materials such as wood, coal, textiles, rubber, rocks, concrete, asbestos and leather. The method has also now been used for hydraulic mining of coal, tunneling and cleaning and descaling operation. Also, automated deburring machines are being used by the automotive industry. As mentioned above, the mechanism of material removal is by erosion. When high pressure water jet emerges out of a nozzle, it attains a large kinetic energy. When this high velocity jet strikes the workpiece, its kinetic energy is converted into pressure energy inducing high stresses in the work material. When the induced stress exceeds the ultimate shear stress of the material, rupture takes place. The schematic diagram of the process, Fig. 9.23 is very similar to that for AJM. In place of compressor, we have a pump or intensifier to raise the pressure of water. Pressures normally used I A N C Regulating T C Control Chamber E U Valve P N M U S U M I L P F A I T E O R R Nozzle Fig. 9.23. Schematic Diagram of WJM. in the system are in the range of 1500 to 4000 N/mm2. From the pump, the water goes to an accumulator. The accumulator helps in eliminating pulsation and also acts as an energy reservoir since the cutting action may not be continuous. From the accumulator the water is lead to the Unconventional Manufacturing Methods 597 nozzle through a high pressure thick tube which may be carbon steel jacketed stainless steel tube. The material of the nozzle may be sintered diamond, sapphire or Tungsten Carbide. The exit diameter of the nozzle is in the range of 0.05 to 0.35 mm. WJM has got the following advantages: 1. The energy transfer media (water) is cheap, non-toxic and easy to dispose off. 2. The work area remains clean and dust free. 3. The system has no moving parts, therefore, its operating and maintenance costs are low and the process is very safe. 4. Intricate contours can be cut. 5. There is no thermal damage to the work surface as little heat is generated during cutting operation. 6. The process is very convenient for cutting soft and rubber like materials for which the teeth of the conventional saw get clogged. The limitations of the above method are : the initial cost is high and hard materials can not be cut. Recently, a model of the WJM machine which uses high velocity water jets (with abrasives) has been developed at the University of Rhode island in U.S.A., for cutting hard materials. The principle of working is as follows : The process filters tap water to remove particles larger than 0.45microns. The water is then compressed to about 420 MPa, after which it moves through reinforced hoses to the cutting head. There, the abrasive is added and the needle thin stream of water shoots out at 600 m/s. It has been claimed that in such a machine, water jets zip through 25 mm thick pieces of Aluminium at a rate of 100 mm/minute. While lasers don't cut transparent materials, water jets dissect an approximately 32 cm thick glass in a minute. Again, it took just a minute to make a 35.5 mm long slice in a 25 mm thick Zinc-nickel steel composite. In comparison, an EDM machine goes through approximately only 2.5 mm. Also, the water jet (high tech knife) carves up hard ceramics on which even diamond tipped saws bounce off without making a scratch. 9.9.3. Ion Beam Machining (IBM). In IBM, method, the metal is removed by bombarding the work with accelerated ions. These ions collide with the surface atoms of the workpiece and knock them out of the surface. thus the metal removal occurs on atomic scale and hence the process is also called : ion etching, ion milling or ion polishing. Unlike other thermo- electric processes (EBM, LBM, PAM and EDM), no large scale heating of the workpiece surface takes place during the process. The schematic arrangement of IBM is basically simple and is similar to EBM. At the top end of the vacuum chamber is the ion beam generating apparatus, which consists of an electron gun which discharges free electrons into the vacuum chamber filled with argon gas. The gas gets ionized by the electrons. A stream of ions is thus generated and is directed on to the target material, which is clamped to a table at the other end of the vacuum chamber. The work table can be oscillated and rotated. This makes it possible to subject different points on the work surface to the ion beam. It is not necessary to work in an absolute vacuum. About 10–4 torr is suitable. Depending upon the type of power used, there are two systems of IBM. One system uses a d.c. source. It is simple and less expensive but can be used only for conductive materials. The more universal system uses radio frequency equipment. It is more expensive but can be used for any material. The method is similar to chemical etching in the need for masking. However, no undercutting takes place in this method. Also, powerful etchants that can propagate along cracks and degrade the masking material, are not needed in this technique. Metal removal rates will depend upon the workpiece material (for example for aluminium it is twice that for tungsten). The MRR vary upto 598 A Textbook of Production Technology 2 × 10–4 mm3/min. The accuracy of etching is very high. Tolerances in the range of 5 106 mm are possible. Regarding the applications of IBM, the method is mainly used in micromachining of electronic components, like computer memories, figuring optical surfaces and for the precision fabrication of fine wire dies in refractory materials. Typical materials which can be etched by IBM include glass, aluminium, quartz, crystals, silica, agates, porcelains, cermets, mixtures of quartz and asbestos, and numerous metals and oxides including rare earth metals. The method holds greatest promise in the field of electronics. It can be used to etch multilayered structures (for example to form a terminal) without the risk of incompatibility. This is an important advantage since multilayered I.C. circuits are becoming increasingly common. The method has proved extremely valuable in etching surfaces of specimens prior to studying their microstructure. The material microstructure is clearly revealed, since the method does not alter the work surface. Also, the etched surface is equally well suited to both optical and electron microscope. The method is also used for the deposition of thin film of material, particularly in electronic industries. Typically, the material to be deposited is made the anode in a low-pressure argon or other rare gas atmosphere. Gaseous ions bombard the cathode, sputtering its material on the substrate. Deposition of thin coatings on such products like razor blades is also common. The Advantages of IBM Are : MRR are easily controlled, no residue, no undercutting as with CHM, no etchants are required, in figure etching resolution is limited only by the resolution of the mask and the method is almost universal. The main drawbacks of the method are : MRR are very slow, it is relatively expensive and thermal or radiation damage may occur in some materials. PROBLEMS 1. What prompted the development of unconventional machining and forming processes ? 2. What are the advantages of electrical machining methods ? 3. What are the limitations of electrical machining methods ? 4. What is the principle of EDM ? 5. Explain the function of the dielectric fluid in EDM ? 6. Name the common dielectric fluids used in EDM. 7. Explain the function of servo - mechanism in EDM. 8. What are the requirements of tool material for EDM ? Name the common tool materials. 9. Give the advantages and disadvantages of EDM. 10. Give the product applications of EDM. 11. Explain the principle of ECM. 12. List the advantages of ECM. 13. What is the function of electrolyte in ECM ? List the common electrolytes used in ECM. 14. List the requirements of tool material for ECM. Write the commonly used tool materials. 15. List the product applications of ECM. 16. Compare the similarities and differences of ECM and EDM. 17. Explain the principle of ECG. 18. What is the difference between ECG and conventional grinding ? Unconventional Manufacturing Methods 599 19. List the product applications of ECG. 20. List the advantages of ECG over conventional grinding. 21. Explain the principle of chemical milling. 22. Explain the purpose of a chemical milling. 23. What is the purpose of a photosensitive resist ? 24. Explain the steps in chemical milling using photosensitive resists. 25. Differentiate between the chemical blanking and contour machining. 26. List the advantages of chemical milling. 27. List the limitation of chemical milling. 28. List the product applications of chemical milling. 29. Explain the principle of USM. 30. List the common materials used for tool in USM. 31. List some abrasive powders commonly used in USM. 32. Compare the methods of attaching tool faces to tool holders. 33. What is the difference between USM and conventional grinding ? 34. Give the leading advantages of USM. 35. Define an electron beam. 36. Explain why EBM process is performed usually in a vacuum chamber. 37. List the product applications of EBM. 38. List the limitations of EBM. 39. What is laser ? 40. Explain the principle of LBM. 41. Contrast LBM and EBM. 42. List the product applications of LBM. 43. Explain the principle of PAM. 44. What is the difference between HERF and HVF. 45. Compare confined system and non-confined systems of explosive forming. 46. Explain the principle of explosive forming. 47. Compare confined system and non-confined systems of explosive forming. 48. List the common explosives used in explosive forming. 49. What is “Stand-off” distance. Explain its importance in explosive forming. 50. Explain the principle of electro-hydraulic forming. 51. What are the main advantages and limitations of electro-hydraulic forming ? 52. Explain the principle of electro-magnetic forming. 53. List the product applications of electro-magnetic forming. 54. With the help of neat diagrams, explain the working of a petro- forge hammer. 55. What is the principle of pneumatic forming ? 56. What is high velocity forming ? Is drop hammer forging a high velocity process ? Describe the basic principles of high velocity forming methods and discuss the advantages and limitations of the high velocity forming methods in comparison with their low velocity counterparts. 57. Explain the method of AJM with the help of a schematic diagram. 600 A Textbook of Production Technology 58. Name the abrasives and carrier gases used in AJM. 59. Write the applications of AJM. 60. Explain the method of WJM with the help of a schematic diagram. 61. Write the applications of WJM. 62. Explain the method of IBM. 63. Write in detail about the applications of IBM. 64. With help of neat sketches, describe the following processes : WCEDM, EDG, ECH, ECD. 65. Explain why the mechanical properties of workpiece materials are not significant in most of the NTMM ? 66. What should be the properties of maskants used in CHM ? 67. Differentiate between ECM and CHM. 68. List the advantages of WJM. 69. What is the purpose of abrasives in ECG ? 70. What type of work is most suitable for LBM ? 71. List the main specifications of NTMM Machines. Machine Main Specifications EDM Tank Size USM Hole , kW EBM kW LBM kW ECM Amp. kVA CHM Tank Size Jet Machining Jet Pressure 72. Write a short note on Powder Mixed Electric Discharge Machining (PMEDM). PMEDM :- It has been noted under Art. 9.2 that the surface finish obtained by conventional EDM is of the order of 0.2 μm. To get high surface quality and mirror like characteristics, parts are subjected to mechanical polishing after EDM, which is a wastage of time and energy. A new variant of EDM has been developed to improve the efficiency and the surface finish of the workpiece. It consists in mixing a suitable material in the powder form, in the dielectric fluid, as a suspension at the tool-workpiece interface, and is termed as Powder mixed EDM (PMEDM). The mixed powder facilitates the bridging action and minimizes the insulation strength of the dielectric fluid. The machining becomes more stable and efficient. Graphite powder is mixed in the dielectric fluid and the performance is measured in terms of MRR, Surface roughness (SR), and Tool Wear Rate (TWR). The results show that MRR increases, whereas, SR and TWR are reduced. 73. What for CHM is used? 74. What are the limitations of USM? 75. Where is USM employed? 76. How much power density a LASER can produce? 77. What are the different types of Lasers used in machining operations. 78. What are the limitations of LBM? 79. What is the effect of ECM on mechanical properties of work materials? 80. What is the effect of EDM on mechanical properties of work materials? Chapter 10 Powder Metallurgy 10.1. INTRODUCTION Powder Metallurgy, P/M, is a manufacturing process in which components are directly produced by bringing a powder of the starting material into the desired final shape by compressing the powder in dies. Strength and other properties are imparted to the components by subsequent sintering operations. It is one of the cheapest mass production process for manufacturing high quality, high strength, complex parts with a high degree of accuracy. Porous parts as well as parts with high density structures may be produced. The process is commercially and economically adaptable only to mass production, due to expensive dies and machines. Basic steps of the process:— The production of a component by P/M involves the following basic steps:— 1. Preparation of powder/powders of the desired composition. 2. Mixing and Blending: In this step, more than one powders are mixed thoroughly and blended to ensure their even distribution, or a powder is mixed with lubricants, additives or binders that might be used. 3. Compacting: In this step, the blended mixture is compacted to bring the finely divided particles or powder into close proximity while imparting the desired part configuration and providing green strength to the part. 4. Sintering: In this step, the compacts obtained after step 3 are heated at elevated temperature to establish permanent strong bonds between adjacent particles and thereby to impart strength to the compacts. 10.2. ADVANTAGES AND LIMITATIONS OF P/M Advantages: 1. One major advantage is its cost effectiveness in producing certain parts as compared to other manufacturing processes. This is due to the fact that parts can be made with close tolerance and surface finish which eleminates the necessity of any further processing. So, scrap is eliminated. Also labour cost is low since no skilled workers are required as the process can be fully automated. 2. The second major advantage is that certain types of parts can be made only by P/M by mixing different metals, metals and non-metals, metals and ceramics etc., to achieve the desired properties of the component. Some examples are: (a) Electrical contact points must be both wear resistant and refractory and have good electrical conductivity. For this, many combinations of metals have been developed: W-Cu, W-Co, W-Ag, Mo-Ag, Ni-W-Cu, and Ni-Ag. As is evident, the combinations include metals that are wear resistant and refractory (W, Mo, Ni etc) and metals 601 602 A Textbook of Production Technology which are electrical conductors (Cu and Ag). Some compositions are: W or Mo with 25 to 50% Ag or Cu and WC with 35 to 55% Ag. There is no other way of combin- ing them except P/M. If melted, these metals will not mix but will separate like oil and water. (b) Combining metallic and non-metallic materials: Electrical brushes for motor are made by blending Cu or Ag (20 to 97%) with graphite. Tin, lead, iron and silica may also be added in small quantities to wear resistance (iron and lead can not mix in a liquid state). Another example is of producing heavy duty metallic frictional materials for brakes and clutches, such as Cu-Sn or Cu-Zn alloys with embedded SiO2, Al2O3 etc. (c) Metals with melting point temperatures too high for castings can be processed by P/ M. For example, particles of WC and Co are mixed together (Co acts as the binder for WC particles) to produce cemented carbides which act as tool materials. Simi- larly W filaments for incandescent light bulbs are manufactured by P/M. The fila- ments should have ductility, high melting point and proper electrical conductivity. For this W is the most suitable material. The process consists of forming billets of W from its powder by P/M method. Then the wires are made by first forging and then wire drawing these billets. (d) Production of ‘Cermets’ (ceramics + metals). A typical composition is: Al2O3 + upto 10% of (W, Mo, Boron, Ti etc.).The material is being increasingly used as tool material. (e) Diamond bonded with metal (typically Cu and 15 to 20% tin). (f) Mechanically alloyed super alloys. (g) Nuclear fuel elements with UO2 dispersed in alumina or stainless steel. (h) Silver infiltrated tungsten is used in nozzles for rockets and missiles. (i) Production of heavy metal: an alloy of W containing 6% Ni and 4% Cu. It can be used for radium containers, balancing weights and similar applications where high density is desirable. (j) Production of electric resistance welding electrodes from mixtures of copper and tungsten. Here, it is possible to retain much of the relative high electric conductivity of copper while realizing high strength and ability to withstand high temperatures of tungsten. (k) Dispersion strengthened alloys in which a high hot strength is secured by the pres- ence of finely divided and distributed stable oxides, for example, (i) Jet engine components of thoriated dispersed nickle with 2% ThO2 (ii) Copper welding electrodes with dispersed alumina (Al2O3). (l) Porosity of the part can be controlled over wide limits to fabricate products with special features. Special screens and filters (from bronze, Ni and stainless steel pow- ders) used in controlling the flow of gases and liquids are made with porosity as high as 80%. Less porous structures are created for self lubricating bearings, slide strips, expan- sion plates, gears, gear pumps, cams etc. Parts of this kind are sintered with a porosity of 40% of the total volume followed by impregnation with a suitable lubri- cant. Parts with unusual damping properties resulting in quieter operation of mating parts can be produced. (m) Magnetic applications: These include magnetically soft materials such as Fe, Fe-3Si, Fe-50Ni. These, because of their mechanical softness are difficult to machine, but are easily formed into final shape by P/M. Alnico permanent magnets (Fe-Al-Ni-Co) can be sintered, being superior to those cast. Powder Metallurgy 603 Other advantages of P/M are: (3) Powders available in pure state produce items of extreme purity. (4) High production rates; can be 500 to 1000 pieces per hour (5) Complex shapes can be produced (6) Bimetallic and laminated special purpose parts can be made from mould layers of different metal powders, securely and permanently inter locked. Limitations 1. Dies and equipment costs are high. So, the process is economically feasible only for mass production. Minimum production run of 10,000 parts is needed to offset the tooling costs. Dies are made for long production runs, of the order of 1,50,000 to 200,000 parts. 2. The material cost in powder form is high. However, high powder material costs are usually offset by an almost total absence of scrap. Part costs are also reduced by eliminating machining. Labour costs are also comparitively low. 3. Metal powders are sometimes difficult to store without some deterioration. 4. There is little flow of metal during compaction, so intricate designs in the products are difficult to attain. 5. A completely dense product is not possible by this process. Also, a uniformly high-density product is very difficult to produce. 6. Part size and weight are restricted. These are regulated by the capacity of available press and compressibility characteristics of the various powder combinations. P/M is principally restricted to the manufacture of comparatively small parts. Part weight can be under 5 newtons. However, parts weighing upto 900 newtons have been successfully mass produced by P/M. Typical part sizes: 100 mm 150 mm long on 900 kN capacity press 250 mm 200 mm long on 900 to 2700 kN capacity press. 7. Some metal powders in a finely divided state, for example, of Al, Mg, Zr and Ti, present explosion and fire hazards. 8. Product Design limitations, which will be discussed ahead. 9. The residual porosity, makes sintered compacts rougher than the compacting die. 10. Low mechanical properties compared to other methods for similar parts. Low impact and fatigue properties 11. Some thermal difficulties are experienced during sintering operation. This is particularly true for low melting point metals such as Sn, Pb, Zn and Cd. During the sintering operations, the atmosphere in the furnace should be reducing to avoid the formation of oxides which will have adverse affects on sintering process and result in an inferior product. The oxides of the above mentioned metals can not be reduced at temperatures below the melting point of the metal, whereas the sintering temperature is always less than the melting point of the metal. 10.3. MANUFACTURE OF METAL POWDERS There are many methods available for the production of powders, depending upon the type and nature of the metal. We discuss below, some of the common methods :— 1. Atomization. In this method, Fig 10.1a, molten metal is broken up into small droplets by spraying it against an oncoming stream of compressed air, inert gas or water jets. With air and water atomization, the powder always gets oxidised. The particle shape and size can 604 A Textbook of Production Technology Molten Metal Tundish Nozzle Atomizing Atomizing Spray Crusher Chamber (water or gas) Wheels Powder Metal Metal Powder T (a) Atomization R (b) Crushing (Gyratory Crusher) U N N Metal I Balls O N or S Rods (c) Milling (Ball or Rod) (d) Milling (Hammer) + – Electrolyte Powdered Metal (e) Electrolytic Deposition Fig. 10.1. (a to e). Methods of Producing Metal Powders. be readily varied by controlling the process parameters, that is, metal flow rate or the pressure and temperature of the stream of air or gas. The powder particles will generally be spherical or pear shaped. A standard dust collector system may be used to collect the powder. The method is mainly used for low melting point metals/alloys such as brass, bronze, zinc, tin, lead and aluminium powders, because of the corrosion action of the metal on the orifice or nozzle (through which the molten metal emerges) at high temperature. With suitable design, the method can be employed for producing powders of other materials also, such as : stainless steel, nickle-based super alloys, titanium alloys and cobalt-base alloys etc. The other variations of the melt atomization method are : (a) An electric arc is struck between non-rotating, non-consumable tungsten electrode and rotating consumable electrode. It is carried out in a chamber filled with an inert gas (Argon gas). The metal droplets from the rotating consumable electrode are thrown off, are collected and are finally crushed to the required powder size. Powder Metallurgy 605 (b) In the “Soluble gas process”', the molten metal in the heated container is forced under pressure to flow through a pipe into a vacuum and break into small particles. The melt atomization method is mainly used for producing pre- alloyed powders. 2. Machining. This method produces coarse powder particles and is used mainly for producing magnesium powder. 3. Reduction. Pure metal is obtained by reducing its oxide with a suitable reducing gas at an elevated temperature (below the melting point). This pure metal is then crushed and milled to a powder. The resulting particles are of irregular shape and are quite porous. The process is cheap and a large amount of powder is made by this method. For iron, the reaction is: heat Fe3O4 + + 4 CO 3 Fe + 4 CO2 Hydrogen reduction: heat Cu 2 O + H 2 2 Cu + H 2 O Powders of W, Mo, Ni and Co are also made by this method by hydrogen reduction. 4. Crushing and Milling. These methods are mainly used for brittle metals and utilize various types of crushers, rotary mills and stamping mills to break down the metals by crushing and impact. In earlier stages of powder preparation, gyratory, cone or roll crushers (Fig 10.1b) are used to crush brittle metals. For finer powder, the metal particles are fractured by impact. In ball mill (Fig 10.1c) which is a horizontal barrel shaped container holding a quantity of balls (made of a metal or ceramic), which being free to tumble about as the container rotates, crush and abrade any powder particles that are introduced into the container. Higher energy is imparted to the balls and milling is accelerated by using a vibratory mill in which the drum rotates about the horizontal as well as vertical axes. A similar action is achieved by rotating blades in hammer mill (Fig. 10.1d) or by rods placed in a horizontal axis rotating drum (rod mill). Finer particles are obtained when particles are hurled against a hard, stationary surface, either by an air or gas jet or in a slurry (impact milling). Finer particles can also be obtained when particles entrained in two opposing fluid streams impact on one another (fluid energy milling). 5. Electrolytic deposition. The process is similar to electroplating. For producing iron, impure steel plates act as anodes in tanks containing an electrolyte. Sheets of stainless steel are also placed in the tank and act as cathodes. When D.C. current is passed through the electrolyte, pure iron gets deposited on cathode. The cathode plates are then removed and the electrolytic iron is stripped from them. The deposited material usually exists as a soft spongy dendritic structure. Additional crushing and milling is necessary. The method is used for copper, iron, silver and tantalum powders, (Fig. 10.1e). 6. Shotting. In this method, the molten metal is poured through a sieve or orifice and is cooled by droping into water. Most metals can be shotted. The droplets are transformed into small particles as they are agitated and cooled in water. 7. Granulation. In this method, the molten metal is converted into small particles by rapidly stirring the metal while it is cooling. 8. Condensation of Metal Powders. The powders of some metals like magnesium, zinc and cadmium can be produced by boiling them and then condensing the vapours. 606 A Textbook of Production Technology 9. Some powders of ductile titanium alloys are made from castings. Hydrogen gas is introduced to form brittle hydrides which can be milled into a powder. Ductility is then restored by driving off the hydrogen by heating to about 350°C when the powder will dissociate into pure metal and hydrogen. 10. Thermal Decomposition. In this method, metals are made to combine with carbon monoxide to from volatile carbonyls. At suitable temperature and pressure, these carbonyls decompose to get pure metal. The method is used for Iron, Nickel, Molybdenum and Tungsten. The reactions are : Ni + 4CO Ni (CO) 4 Fe + 5CO Fe (CO)5 Mo + 6CO Mo (CO)6 W + 6CO W (CO)6 The powder particles obtained are almost spherical in shape. 10.3.1. Metal Powder Characteristics :— Both the consolidation and the properties of the final product depend upon certain characteristics of metal powders which are discussed below :— 1. Surface Area. This is the area per unit mass of the powder. It indicates the area available for bonding and also the area on which adsorbed films or contaminants may be present. 2. Density :— The degree of porosity in a sintered or unsintered powder metallurgy component is determined indirectly by making density measurements. The density of a metal in three different conditions is commonly dealt with in the science of powder metallurgy. These are given below : (i) Theoretical density :— Theoretical density or True density is the mass per unit volume of the solid material. It is the density of a non-porous metal in the solid state, that is, when it has been manufactured by non-powder metallurgy methods such as casting followed by hot and cold working. Any and all the pores get completely closed and the material becomes fully homogeneous. The theoretical density of a material is the highest possible density (100%) it can have. This density is used as a standard basis of comparison with other types of density. For iron, its value is 7.87 gm/cm3. (ii) Apparent density :— The ‘Apparent density’ in gm/cm3 defines the actual volume filled out by the loose powder. It is a very important property of the powder and depends upon the particle shape, size and size distribution. The apparent density of irregularly shaped particles will be lower than that of the spherical particles and so also of the coarser particles as compared to finer particles of the same shape. It is often expressed as a percentage of the fully dense material, that is, as a percentage of true density. It is measured by subtracting the weight of an empty container from the weight of that container and the powder filling it, and then dividing by the inside volume of the container. For iron powder, its value ranges from 2.5 to 3.5 gm/cm3. (iii) Tap density :— This density is obtained by tapping or vibrating the container and signifies the conpaction achievable without the application of pressure. (iv) Green density :— It is the mass of compacted powder in an unsintered pressed briquette per unit volume of the briquette. The green density of iron powder usually ranges from 6.7 to 7.5 gm/cm3. Powder Metallurgy 607 3. Compressibility and Compression Ratio. The term compres- sibility denotes the change in green density with increasing compacting pressure. It is usually given as the density at some specified pressure (450 to 500 MPa). Compression ratio is defined as the ratio of the unpressed volume and pressed volume of the powder for a given mass. Both these properties depend upon the shape, size and size distribution of the powder particles. The compression ratio usually varies between 1.5 and 3. Of course, for very fine powders it may be as high as 10. However a lower, compression ratio is preferred to reduce the depth of die and the plunger movement in the die and thereby the wear and tear of the tools. ‘Compacting ratio’ is defined as the ratio of the green density of the part to the apparent density and it means the same thing as the compression ratio. Other related terms used in powder Metallurgy practice are :– (a) A compact :- It is the briguette made by compacting powder. This compact is called “Green compact”. After this compact is sintered, it is called “Sintered compact” (b) Fill or Powder fill :– It is the amount of powder needed to completely fill the die or container cavity, prior to the compacting operation. The cavity should be filled in the shortest possible time to avoid delay of production. The amount of powder representing this fill is usually expressed as the height of the powder in the die-cavity needed to result in a compact of the desired dimensions and density. Thus, Fill = Median height of compact desired × Compresion Ratio 4. Flow Rate. It is defined as the time required for a measured quantity of powder to flow out of a standard orifice. It is an important property of the powder because the time needed to fill the die should be small to obtain higher production rates and economy. Flow rate or flowability depends upon the shape, size and size distribution of the powder particles. Smaller and spherically shaped particles have the maximum flow rate. Very fine particles (of the order of 1 to 10 microns) will flow just like a liquid. When such a powder is pressed in die, it will flow into complex die cavities. Flowability can also be increased by the addition of stearate to the mix. 5. Particle Shape, Size and Size Distribution. As discussed above, these three properties greatly influence the apparent density, conpressibility and flow rate of the powder. The particle shape depends largely on the method of powder manufacture. It may be spheroidal, nodular, irregular, angular, lamellar, acicular and dendritic. Spheroidal particles have excellent sintering properties. However, irregularly shaped particles are superior for practical moulding and achieving good green strength of the part because they will interlock on compacting. Particle size or fineness is expressed by the diameter of the spheriodal particles and by the average diameter of the other particles. It is determined by passing the powder through standard sieves or by microscopic measurement. Particle size should neither be too large nor too small. Too large particles may not display the required structure for the P/M route and may not result in high densities. On the other hand, it is difficult to handle fine particles and they may tend to agglomerate. Also, their large surface area-to-volume ratio may introduce large quantities of undesirable adsorbed substances and on metals, also oxides. However, finer powders have excellent sintering qualities and are particularly important for ‘Slip Casting’. The usual size of powders used in P/M varies from 4 to 200 microns, with 100 microns being the most used size. Particle size distribution refers to the quantity of each standard particle size in the mix (powder). It influences apparent density, compressibility, flowability, final porosity and the strength of the part. For a given material, the strength of the part will be proportional to its final density after pressing. Theoretically, a powder containing varying particle sizes will result in greater density, because smaller particles will fill up the interspaces between the large particles. However, when mixing, the finer particles have the tendency to separate and segregate. Due to this reason, many users prefer uniform size particles and rely on the compacting pressure to get the final density required. 608 A Textbook of Production Technology The ISO has defined the following qualitative particle shape classification as per ISO-3252: 1. Spherical : Nominally spherical, three dimensional. Produced by : atomization, carbonyl (Fe), precipitation from a liquid. 2. Rounded or : Rounded, irregular shape (3D). Nodular Produced by : atomization, chemical decomposition. 3. Angular : Roughly polyhedral with sharp edges (3D). Produced by : Mechanical disintegration, Carbonyl (Ni) 4. Acicular : Needle shaped (1D) Produced by : Chemical decomposition. 5. Dendritic : Branched, crystalline shape (2D). Produced by Electrolytic Process. 6. Irregular : Lacking any symmetry (3D). Produced by : Chemical decomposition, Mechanical communication. 7. Granular : Irregular but approximately equidimensional, (3D). Produced by : reduction of oxides. 8. Flaky : Plate like (2D), Produced by : Mechanical Comminution 9. Fibrous : Regularly or irregularly thread like (1D). Produced by chemical decomposition, mechanical comminution These shapes have been illustrated in Fig. 10.2. Spherical Dendritic Rounded Irregular Angular Porous Acicular Fragmented Fig. 10.2. Particle Shapes. 10.3.2. Pre-Alloyed and Precoated Powders. Conventionally an alloyed powder product is made from thoroughly mixed and blended powders of alloying metals. However, superior properties are attained if pre-alloyed powders are used, each powder particle being an alloy. Pre-alloyed powders are alloyed in the melting process. Pre-alloyed powders of : Stainless steels. Ni base alloys such as Monel, Titanium alloys, Cobalt base alloys and some low-alloy steels are available. Pre-alloyed powder products have high strength and are corrosion and high temperature resistant. However, mixed and blended powder products are cheaper to produce and require lower pressures to compact. In pre - coated powders, each particle is coated with another element. The product will have different properties from those of the base material, for example, superior corrosion resistance is Powder Metallurgy 609 obtained by the use of stainless steel-clad plate. Precoated powder products are more homogeneous than those made by blending. Pre - coated powders are obtained by passing the powder through a carrier gas, resulting in uniformly coated individual particle. 10.4. MIXING AND BLENDING The aim of this step is to obtain uniform distribution of particle sizes. This step is necessary in the following situations : 1. Making of alloyed powders by combining a homogeneous mixture of carefully weighed and blended mixtures. 2. Combining metallic and non-metallic powders. 3. To ensure filling up of the interstices between large particles, coarser fraction is blended with a fine fraction. This will result in high tap density. 4. Binders are added to powder that would other wise fail to develop adequate green strength, for example, for the production of sintered carbide tool material, cobalt powder is added as a binder to the WC powder. 5. Sintering aids are added to accelerate densification on heating. 6. Lubricants are added to the powders to reduce die wall friction and friction of the individual particles relative to one another. This will result in the attainment of high green compact densities. They also increase the flowability of powders and aid in the ejection of the compacted part. Although the lubricants add to the porosity, they increase the production rate. The principal lubricants are: stearic acid, lithium stearate or powdered graphite. Mixing and blending must be thorough. It is done mechanically often in ‘ball mill’. It may be done either dry or wet. For wet, water or a solvent is added to the dry powder to reduce the possible hazards of dust and explosion. There is a difference in these two operations. Mixing means the thorough intermixing (intermingling) of powders of two or more materials, intermixing of a powder and binder, and intermixing of a powder and lubricants etc. On the other hand, blending means the thorough intermixing of powders (of different grain sizes) of the same nominal composition. 10.5. COMPACTING (BRIQUETTING) The purpose of this step has already been explained under Art. 10.1. The powders are compacted in moulds by the application of pressure to form a so called green compact (a compact without permanent bonding). The compacting pressure may range from 80 to 1600 MPa with more commonly used pressures being 320 to 800 MPa. The density, hardness and also the strength increases with pressure. However, for every metal powder, there is an optimum compacting pressure beyond which there is little improvement in properties. The die cost and press capacity and hence the production cost will increase with increased compacting pressure. (a) For soft powders such as brass, bronze and aluminium, the compacting pressure may range from 100 to 350 MPa. (b) For iron, steel and Ni alloys, the range in 400 to 700 MPa. (c) The pressure range is still higher for harder materials such as WC. During compacting, the green strength of a compact is achieved due to the following factors :— 1. Sliding combined with pressure promotes adhesion and even cold welding with some powders. 2. Mechanical interlocking of particles, especially with particles of irregular shape. So, green strength is lower for more spherical powders even though they pack more closely. 610 A Textbook of Production Technology 3. By the addition of bonding agents which evaporate during sintering, Of course, the green strength is lower when lubricants are added to the powders. Most compacts are made by pressing in dies which have been set up in a sufficiently powerful mechanical or hydraulic presses. The compacting equipment consists of: Die or container, Upper (top) punch and Lower (bottom) punch (Fig. 10.3). The upper punch forms the top shape of the compact and applies pressure during the compacting operation. The lower punch conforms to the lower end of the die cavity. It may or may not apply pressure for compacting depending upon the design, but it acts as ejector for forcing the compact out of the die cavity at the end of the compacting stroke. The die cavity is automatically filled with the required amount of blended powder. Upper Upper Punch Punch Lower Spring Punch Die (Acts only (Container) Lower Punch as Ejector) (Ejector) (a) Single Punch, (b) Single Punch, Fixed Die Floating Die Upper Punch Lower Punch (c) Double Punch, Fixed Die Fig. 10.3. Compacting Equipment (Punch and Die). 1. In the ‘single punch design’ (Fig. 10.3a), the bottom punch acts as a support at the start of the operation and then as ejector at the end of the compacting stroke. The upper punch enters the die cavity to compress the powder. At the end of its downward stroke when it just starts rising upward, the lower punch also starts moving upward to eject the compact out of the die cavity. The drawback of this design is that, since powder does not transmit pressure as a continuous solid would and wall friction also opposes compaction, the compacting pressure tapers off rapidly, giving compacts of higher density close to the upper punch and of diminishing density far away. This limits the depth-to-diameter ratio of the part. This design is suitable only for parts upto about 5 mm thick. The design is also satisfactory under high speed conditions for high density components where it has been found that upto 98% of the force was transmitted. Powder Metallurgy 611 2. The improved design is to have the die or container as floating (supported on springs) and not fixed, Fig. 10.3 (b). It will also move downward by the friction force between the powder and container, when the upper punch enters the die cavity and starts compressing the powder. There will be more uniform density in the compact as compared to the first design. The container should have as low a mass as possible consistent with strength requirements, because it has to be accelerated downward by friction between the powder and the container walls during pressing. The design is suitable for high speed compaction also. 3. In the double punch or counteracting punch, fixed container design, Fig. 10.3c, both the punches enter the die simultaneously to compress the powder. At the end of the downward stroke of the upper punch when it starts moving upwards, the lower punch continues its upward journey to force the compact out of the die cavity. In this design, the minimum density is in the middle section. The variation in density is appreciably less than the single punch design. This design is not suitable for high speed compaction due to the difficulty of closely synchronising the two punches. Multiple-punch Design. With irregular shaped parts and when the thickness of the part changes considerably from point to point, even with the double punch design, powder compacting creates a serious problem of pressure gradients on the part. To equalize these pressure gradients and hence the green density, dies with multiple punches guided within each-other, are used so that the same degree of compaction can be applied everywhere by applying varying pressure on the powder, (Fig. 10.4). Rotary Table Design. In this design, 6 to 30 or more die sets, each complete with top and bottom punches,are arranged in a circle concentric with the axis of the rotating table. The dies are rotated to the stations, where the required operations (filling the die cavity, compacting etc.) are performed automatically. At the last station, the compacts are ejected and the individual dies start another cycle. These machines have high rate of production since work can be done on all of the parts at the same time. Production rate on such a machine may reach even 1000 compacts per minute. Dies. There are two types of dies used in P/M Inner Punch process : non-collapsible and Collapsible. Non - collapsible dies are used when the compact can be Outer conveniently forced out of the die cavity, without Punch damaging the compact. When the compact design is such that it can't be forced out of the die cavity, Step collapsible dies (dies with removable panels) must be used. More complicated designs can be produced on these dies as compared to non-collapsible dies. However, such dies are more expensive and the production rate gets reduced. Core Dies are usually made of high strength tool steels. Rod For larger production runs and severe abrasive conditions, sintered tungsten carbide can be used. Die cavities and punch faces should be lapped and polished Finished to a very high surface finish, preferably below 0.254 Part μ m. Clearances between die walls and punches should Bottom Punch not exceed 0.127 mm and should be held between 0.005 mm and 0.0075 mm for precision parts. To Fig. 10.4. Multiple Punch Design. facilitate ejection of the compact and thereby avoid excessive wear, a slight taper or draft (usually less than 1°) is machined in the die. Dies of WC do not need taper in compacting dies. 612 A Textbook of Production Technology Dies should include an allowance for shrinkage during sintering and also another allowance if sizing is to be done. The die design should also take into account the elastic recovery of the compact when ejected from the die, which can be of the order of 0.5 to 1.5%. Presses. Mechanical presses being faster than hydraulic presses, are adaptable to high production rate, even upto 100 compacts per hour. The maximum production rate for a large hydraulic press may be only 100 compacts per hour. Hydraulic presses are more powerful then mechanical presses. The press capacity can reach a maximum of about 27.5 MN. Mechanical presses are used when less than about 1.5 MN compacting pressure is needed. Hydraulic presses are used for those compacts that require from 1.5 to 20 MN compacting pressure. Press capacity Maximum area of the compact Required compacting pressure From the press capacities and compacting pressures discussed above, most P/M products have cross-sectional area of 1935 mm2. However, sizes upto 6452 mm2 are quite common and even larger ones are being produced. 10.5.1 Other Methods of Compacting 1. Impact Compaction :– Impact compacting of powders on fast hammers or with the aid of explosive charges results in compacts of high density. This reduces the sintering time and limits shrinkage of the compact. The method is particularly advantageous for compact resistant powders. High speed compaction of powders using petro-forge machines has already been explained in chapter 9. In explosive compacting, one or more plungers, placed next to the powder in a closed system, are actuated by buffer plates against which the powerful explosives act. 2. Rolling and Extrusion. Improved consolidation is achieved when individual powder particles are moved relative to each other, as in extrusion or rolling of powders. The products are with uniform and excellent mechanical properties. `Rolling of powders is usually used to manufacture sheets and for cladding a solid base metal. The main applications of extrusion of powders are the production of long solid bars, hollow tubes and multihole filters, which can be cut up as required. The typical applications are: nuclear solid fuel elements and other materials for high temperature applications. Al, Cu, Ni and many other metals can also be extruded. Metals that can be rolled are: Cu, Brass, Bronze, Monel and Stainless Steel. These processes can be done both cold as well as hot. In cold rolling of powders, the powder mixed with a binder is fed from a hopper between two rolls and interlock the powder particles into a sheet. Compaction by rolling is following by sintering and perhaps re-rolling. Some metal powders are extruded cold with a binder. However, the more common procedure is that the powder is first pressed into a billet which is then extruded in the conventional procedure. Hot rolling and extrusion of powders will be discussed after ‘Sintering’. 3. Centrifugal Compacting. In this method, the mould after it is filled with powder, is centrifuged to get a compact of high and uniform density and for this the parts should be of nearly uniform section. The method is particularly suitable for heavy metals such as WC. The pressure generated during centrifuging may be of the order of 3 MPa. 4. Slip or Slurry Casting. In this method, the powder is converted into a slurry (particle size < 0.02 mm) and then poured in a plaster of paris mould, after mould release agents are applied to the mould surface. The mould being porous, the liquid in the slurry gradually drains off into the plaster leaving a solid compact within the mould. Vacuum aids mould filling and speeds up the process of liquid extraction. Centrifuging also helps filling. An invariant of slip casting is ‘drain casting’ for producing hollow compacts. The mould is filled up with slip or slurry and after sufficient Powder Metallurgy 613 time has elapsed to form a dewatered particulate shell, the mould is inverted to pour out the excess slip, leaving behind a hollow product. The compacts are dried and sintered in the usual manner. The main advantage is that expensive dies are not needed and large and complex parts can be made, with improved properties. The main drawback is the time lag in producing parts.“ An important variant of slurry casting involves the forming of thin ( < 1.5 mm thick) tapes, usually of cera- mic powders in an organic carrier. Rolling is one of the many techniques used for this purpose. The green tape obtained is flexible enough to be rolled up. It can be blank- ed, mechanically scored or marked with a laser beam prior to firing so that it can be made into small components such as substrates for integrat- ed circuits. 5. Cold Isostatic Pressing :— In this Pressurised method a uniform density throughout the Fluid (Gas) compact can be achieved. An elastic Deformable Mould deformable mould (reusable rubber or single use metal) contains the metal powder while omni directional pressure is applied by means of a pressure medium inside a pressure vessel, (Fig. 10.5). If the medium is a gas, the method is called iso-static pressing and when the medium is a liquid (usually water) it is called hydrostatic pressing. Pressures of 300 MPa to 550 MPa can be achieved. Other advantages of the method are: uniform strength in all directions, low equipment costs and a higher green compact strength as compared to most other methods. Metal powders which can be compacted by this Powder Container method are: Al, Mg, W, Beryllium, Iron and Stainless Steel. An internal cavity of the moulding is made by a metal core or mandrel properly positioned in the container. Upon Fig. 10.5. Cold Iso-Static Pressing. completion of the compacting operation, the compact is taken out of the container and the mandrel withdrawn. In the above method, the pressurizing medium acts directly on the powder-filled mould. This is known as “Wet-bag tooling”. In “Dry-bag tooling”the pressurizing medium acts on a membrane, which is in contact with the powder-filled mould. Advantages 1. Uniform density distribution, even in shapes with large aspect ratios and those containing undercuts. 2. No power lubrication is necessary. Thus, no burn-off cycle is necessary during sintering. 3. Larger parts can be compacted economically. Limitations 1. Dimensional control is not very precise. 2. Production rates are lower, compared to punch-die method. 3. Lesser surface smoothness, compared to punch-die method. 614 A Textbook of Production Technology 10.6. SINTERING Green compact as obtained after compacting operation is not very strong and is very brittle and can't be used as it is. In order that it becomes a usable powder metal product, it must undergo the sintering operation. The green compact is placed in a furnace and is soaked in heat until the individual powder particles are no longer discernible. So, sintering can be defined as the bonding of adjacent surfaces of particles in a mass of metal powder, by heating. The time of exposure, the temperature and the rate of cooling are closely controlled. The first stage in the sintering operation is the purging or drying stage. The compact is heated at low temperatures to drive off liquid constituents (lubricants, water etc.). The heating should be gradual since, fast heating will cause sudden vaporization and could result in the disintegration of the compact. The time for drying increases with increasing wall thickness of the compact. Vacuum accelerates drying. If organic binders are to be burnt off, sufficient oxygen must be available for their combustion. After the first stage, the actual sintering operation starts at an elevated temperature. The sintering temperature is normally below the melting points of all powder constituents. However, in certain cases, the sintering temperature may be above the melting point of one of the metal powders. The sintering temperature for a powder varies over a range (0.7 to 0.9 times the melting point), but there is usually an optimum maximum sintering temperature for a given set of conditions beyond which no gain is affected. Sintering time varies with different metals but in most cases, the effect of heat is complete in a very short time and there is no economy in prolonging the operation. Typical sintering temperatures and times for some important metal powders are given in Table 10.1. Table 10.1 S. No. Material Temp °C Time, min. 1. Iron 1010 — 1150 8 — 45 2. Copper 840 — 900 12 — 45 3. Brass 840 — 900 10 — 45 4. Bronze 760 — 870 10 — 20 5. Nickle 1010 — 1150 30 — 45 6. Stainless steel 1095 — 1285 30 — 60 7. Tungsten and Tantalum 2345 480 8. Alnico magnets 1200 — 1300 120 At high temperatures, there is a tendency of surface oxide films to form due to the reaction between the compact and the furnace atmosphere. This is avoided by sintering in inert atmosphere (neutral or reducing). N2 is neutral and is extensively used. H2 is a very effective reducing agent but must be handled with care to avoid explosion. N2 + 10% H2+ methane, dissolved NH3 and partially combusted hydrocarbon gases are frequently used. These gases will react with the scale on the metals and clean away the contaminations. In the sintering of steel, the carbon content is also controlled and in some cases, steels are carburized in a CO-containing atmosphere. Vacuum sintering also provides a neutral atmosphere, but is a more costly process. It improves ductility and strength. The major type of furnace used for sintering is the electric resistance furnace. It can be batch type or continuous type. Continuous type furnace has a wire mesh belt to carry the compacts through the furnace. A typical sintering furnace has three main parts, (Fig. 10.6) :