A Guide to Goniometry PDF
Document Details
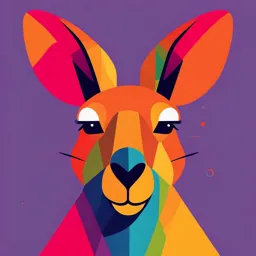
Uploaded by ProfoundFuchsia6830
George Washington University
D. Joyce White, PT, DSc Cynthia C. Norkin, PT, EdD
Tags
Summary
This document is a guide to goniometry, a technique used in physical therapy to measure the range of motion in joints. It explains the basic concepts, kinematics, and how to perform the measurements.
Full Transcript
Goniometry The term **goniometry** is derived from two Greek words: *gonia,* meaning "angle," and *metron,* meaning "measure." Therefore, goniometry refers to the measurement of angles, in particular the measurement of angles created at human joints by the bones of the body. The examiner obtain...
Goniometry The term **goniometry** is derived from two Greek words: *gonia,* meaning "angle," and *metron,* meaning "measure." Therefore, goniometry refers to the measurement of angles, in particular the measurement of angles created at human joints by the bones of the body. The examiner obtains these measurements by placing the parts of the measuring instrument, called a goniometer, along the bones immediately proximal and distal to the joint being evaluated. Goniometry may be used to determine both a particular joint position and the total amount of motion available at a joint. Example: The elbow joint is evaluated by placing the parts of the measuring instrument on the humerus (proximal segment) and the forearm (distal segment) and measuring either a specific joint position or the total arc of motion (Fig. 1.1). Goniometry is an important part of a comprehensive examination of joints and surrounding soft tissue. A comprehensive examination typically begins by interviewing the individual and reviewing records to obtain an accurate description of current symptoms; functional abilities and activities of daily living; occupational, social, and recreational activities; and medical history. Observation of the individual's body to assess bone and soft tissue contour, as well as skin and nail condition, usually follows the interview. Gentle palpation is used to determine skin temperature and the quality of soft tissue deformities and to locate pain symptoms in relation to anatomical structures. Anthropometric measurements such as leg length, leg circumference, and body volume may be indicated. The performance of active joint motions by the individual during the examination allows the examiner to screen for abnormal movements and gain information about the individual's willingness to move. If abnormal active motions are found, the examiner performs passive joint motions in an attempt to determine reasons for joint limitation. Performing *1* **Basic Concepts** C H A P T E R *D. Joyce White, PT, DSc* *Cynthia C. Norkin, PT, EdD* **FIGURE 1.1** The left upper extremity of an individual in the supine position is shown. The parts of the measuring instrument have been placed along the proximal (humerus) and distal (radius) body segments and centered over the axis of the elbow joint. When the distal segment has been moved toward the proximal segment (elbow flexion), a measurement of the arc of motion can be obtained. 145° Distal segment Proximal segment 4 PART I Introduction to Goniometry and Muscle Length Testing passive joint motions enables the examiner to assess the tissue that is limiting the motion, detect pain, and make an estimate of the amount of motion. Goniometry is used to measure and document the amount of active and passive joint motion as well as abnormal fixed joint positions. Following the examination of active and passive range of motion, resisted isometric muscle contractions, joint integrity and mobility tests, and special tests for specific body regions are used in conjunction with goniometry to help identify the injured anatomical structures. Tests to assess muscle performance and neurological function are often included. Diagnostic imaging procedures and laboratory tests may be needed. Functional outcome measures are often required for Medicare, Medicaid, and health insurance documentation. Goniometric data used in conjunction with other information can provide a basis for the following: Determining the presence, absence, or change in impairment1 Establishing a diagnosis Developing a prognosis, treatment goals, and plan of care Evaluating progress or lack of progress toward rehabilitative goals Modifying treatment Motivating the individual Researching the effectiveness of therapeutic techniques or regimens (for example, measuring outcomes following exercises, medications, and surgical procedures) Fabricating orthotics and adaptive equipment Kinematics Kinematics is the study of motion without regard for the forces that are creating the motion. When referring to the human body, kinematics describes the motion of bony segments including the type, direction, and magnitude of motion; location of the bony segment in space; and the rate of change or velocity of the segment. The three types of motion that a bony segment can undergo are translatory (linear displacement), rotary (angular displacement), or more often a combination of translatory and rotary motion.2 In translatory motion, all points on a segment move in the same direction at the same time. In rotary motion, the bone spins around a fixed point. These three types of motion will be explained in more detail in the following subdivisions of kinematics: arthrokinematics and osteokinematics. In arthrokinematics, the focus is on how joint surfaces move and interact, whereas in osteokinematics, the focus is on movements of the shafts of bones. Arthrokinematics Motion at a joint occurs as the result of movement of one joint surface in relation to another joint surface. **Arthrokinematics** is the term used to refer to the movement of joint surfaces.3,4 The movements of joint surfaces are described as slides (or glides), spins, and rolls. A **slide (glide),** which is a translatory motion, is the sliding of one joint surface over another, as when a braked wheel skids (Fig. 1.2). A **spin** is a rotary motion, similar to the spinning of a toy top. All points on the moving joint surface rotate around a fixed axis of motion (Fig. 1.3). A **roll** is also a rotary motion, similar to the rolling of the bottom of a rocking chair on the floor or the rolling of a tire on the road (Fig. 1.4). In the human body, slides, spins, and rolls usually occur in combination with one another and result in angular movement of the shafts of the bones. The combination of the sliding and rolling is referred to as roll-gliding or roll-sliding4 and allows for increased motion at a joint by postponing the joint **FIGURE 1.2** A slide (glide) is a translatory motion in which the same point on the moving joint surface comes in contact with new points on the opposing surface, and all the points on the moving surface travel the same amount of distance. Axis **FIGURE 1.3** A spin is a rotary motion in which all the points on the moving surface rotate around a fixed central axis. The points on the moving joint surface that are closer to the axis of motion will travel a smaller distance than the points farther from the axis. CHAPTER 1 Basic Concepts 5 compression and separation that would occur at either side of the joint during a pure roll. The direction of the rolling and sliding components of a roll-slide will vary depending on the shape of the moving joint surface. If a convex joint surface is moving, the convex surface will roll in the same direction as the angular motion of the shaft of the bone but will slide in the opposite direction (Fig. 1.5A). If a concave joint surface is moving, the concave surface will roll and slide in the same direction as the angular motion of the shaft of the bone (Fig. 1.5B). Arthrokinematic motions are examined for amount of motion, tissue resistance at the end of the motion, and effect on the individual's symptoms.5 The ranges of arthrokinematic motions are very small and cannot be measured with a goniometer or standard ruler. Instead, arthrokinematic motions are subjectively compared with the same motion on the contralateral side of the body or with an examiner's past experience testing people of similar age and gender as the individual. An ordinal grading scale of 0 to 6 is often used to describe the amount of arthrokinematic motions6 (Table 1.1). These motions are also called accessory or joint play motions. Osteokinematics **Osteokinematics** refers to the gross movement of the shafts of bony segments rather than the movement of joint surfaces. The movements of the shafts of bones are usually described in terms of the rotary or angular motion produced, as if the movement occurs around a fixed axis of motion. Goniometry measures the angles created by the rotary motion of the shafts of the bones. Some translatory shifting of the axis of motion usually occurs during movement; however, most clinicians find the description of osteokinematic movement in terms of Axis Axis **FIGURE 1.4** A roll is a rotary motion in which new points on the moving joint surface come in contact with new points on the opposing surface. The axis of rotation has also moved, in this case to the right. A B Angular motion Angular motion Roll Roll Slide Slide **FIGURE 1.5** (A) If the joint surface of the moving bone is convex, sliding is in the opposite direction to the rolling and angular movement of the bone. (B) If the joint surface of the moving bone is concave, sliding is in the same direction as the rolling and angular movement of the bone. TABLE 1.1 Arthrokinematic (Accessory/Joint Play) Joint Motion Grades Grade Joint Status 0 Ankylosed 1 Considerable hypomobility 2 Slight hypomobility 3 Normal 4 Slight hypermobility 5 Considerable hypermobility 6 Unstable 6 PART I Introduction to Goniometry and Muscle Length Testing just rotary motion to be sufficiently accurate and use goniometry to measure osteokinematic movements. Planes and Axes Osteokinematic motions are classically described as taking place in one of the three **cardinal planes** of the body (sagittal, frontal, transverse) around three corresponding **axes** (medial--lateral, anterior--posterior, vertical). The three planes lie at right angles to one another, whereas the three axes lie at right angles both to one another and to their corresponding planes. The **sagittal plane** proceeds from the anterior to the posterior aspect of the body. The median sagittal plane divides the body into right and left halves.7 The motions of flexion and extension occur in the sagittal plane (Fig. 1.6). The axis around which the motions of flexion and extension occur may be envisioned as a line that is perpendicular to the sagittal plane and proceeds from one side of the body to the other. This axis is called a **medial--lateral axis.** All motions in the sagittal plane take place around a medial-- lateral axis. The **frontal plane** proceeds from one side of the body to the other and divides the body into front and back halves. The motions that occur in the frontal plane are abduction and adduction (Fig. 1.7). The axis around which the motions of abduction and adduction take place is an **anterior--posterior** **axis.** This axis lies at right angles to the frontal plane and proceeds from the anterior to the posterior aspect of the body. Therefore, the anterior--posterior axis lies in the sagittal plane. The **transverse plane** is horizontal and divides the body into upper and lower portions. The motion of rotation occurs in the transverse plane around a vertical axis (Fig. 1.8). The **vertical axis** lies at right angles to the transverse plane and proceeds in a cranial to caudal direction. The osteokinematic motions described previously are considered to occur in a single plane around a single axis. Combination motions such as circumduction (flexion-- abduction--extension--adduction) are possible at many joints, but because of the limitations imposed by the uniaxial design of the measuring instrument, only motion occurring in a single plane can be measured in goniometry. Medial-- lateral axis Sagittal plane **FIGURE 1.6** The shaded areas indicate the sagittal plane. This plane proceeds from the anterior aspect of the body to the posterior aspect. Motions in this plane, such as flexion and extension of the upper and lower extremities, take place around a medial--lateral axis. Anterior-- posterior axis Frontal plane **FIGURE 1.7** The frontal plane, indicated by the shaded area, proceeds from one side of the body to the other. Motions in this plane, such as abduction and adduction of the upper and lower extremities, take place around an anterior-- posterior axis. CHAPTER 1 Basic Concepts 7 The type of motion that is available at a joint varies according to the structure of the joint. Some joints, such as the interphalangeal joints of the digits, permit a large amount of motion in only one plane around a single axis: flexion and extension in the sagittal plane around a medial--lateral axis. A joint that allows motion in only one plane is described as having one **degree of freedom of motion.** The interphalangeal joints of the digits have one degree of freedom of motion. Other joints, such as the glenohumeral joint, permit motion in three planes around three axes: flexion and extension in the sagittal plane around a medial--lateral axis, abduction and adduction in the frontal plane around an anterior-- posterior axis, and medial and lateral rotation in the transverse plane around a vertical axis. The glenohumeral joint has three degrees of freedom of motion. The planes and axes for each joint and joint motion to be measured are presented in Chapters 4 through 13. Range of Motion **Range of motion (ROM)** is the arc of motion in degrees between the beginning and the end of a motion in a specific plane.1 The arc of motion may occur either at a single joint or at a series of joints.5 The starting position for measuring all ROM is either the anatomical or neutral position. The anatomical position is described in the 41st edition of *Gray's* *Anatomy* as a posture in which the upper limbs are by the person's side and the palms of the hands are facing forward with the fingers extended7 (Fig. 1.9A). The lower limbs are together and facing forward. The neutral position, which is used to measure rotation ROM in the transverse plane, places the upper extremity joints halfway between medial and lateral rotation, and supination and pronation (Fig. 1.9B). **FIGURE 1.8** The transverse plane is indicated by the shaded area. Movements in this plane take place around a vertical axis. These motions include rotation of the shoulder (A), head (B), and hip, as well as pronation and supination of the forearm. A B Anatomical position Neutral position **FIGURE 1.9** (A) In the anatomical position, the forearm is supinated so that the palms of the hands face anteriorly. \(B) When the forearm is in a neutral position (with respect to rotation), the palm of the hand faces the side of the body. 8 PART I Introduction to Goniometry and Muscle Length Testing The three notation systems used to define ROM are the 0- to 180-degree system, the 180- to 0-degree system, and the 360-degree system. In the **0- to 180-degree notation system,** the upper- and lower-extremity joints are at 0 degrees for flexion--extension and abduction--adduction when the body is in the anatomical position, and at 0 degrees for rotation when the body is in the neutral position (see Fig. 1.9). Normally, a ROM begins at 0 degrees and proceeds in an arc toward 180 degrees. This 0- to 180-degree system of notation, also called the **neutral zero method,** is widely used throughout the world. First described by Silver8 in 1923, its use has been supported by many authorities, including Cave and Roberts,9 Moore,10 the American Academy of Orthopaedic Surgeons,11,12 and the American Medical Association.1 Example: The ROM for shoulder flexion, which begins with the shoulder in the anatomical position (0 degrees) and ends with the arm overhead in full flexion (180 degrees), is expressed as 0 to 180 degrees. In the preceding example, the portion of the extension ROM from full shoulder flexion back to the zero starting position does not need to be measured because this ROM represents the same arc of motion that was measured in flexion. However, the portion of the extension ROM that is available beyond the zero starting position must be measured (Fig. 1.10). Documentation of extension ROM usually incorporates only the extension that occurs beyond the zero starting position. The term **hyperextension** is used to describe a greater than normal extension ROM. Two other systems of notation have been described. The **180- to 0-degree notation system,** first described by Clark, defines the anatomical position as 180 degrees.13 The ROM begins at 180 degrees and proceeds in an arc toward 0 degrees. The **360-degree notation system,** first described by West, also defines the anatomical position as 180 degrees.14 The motions of flexion and abduction begin at 180 degrees and proceed in an arc toward 0 degrees. The motions of extension and adduction begin at 180 degrees and proceed in an arc toward 360 degrees.15 These two notation systems are more difficult to interpret than the 0- to 180-degree notation system and are infrequently used. Therefore, we have not included them in this text. Active Range of Motion **Active ROM** is the arc of motion produced by the individual's voluntary unassisted muscle contraction. Having an individual perform active ROM provides the examiner with information about the individual's willingness to move, coordination, muscle strength, and joint ROM. If pain occurs during active ROM, it may be due to contracting or stretching of "contractile" tissues, such as muscles, tendons, and their attachments to bone. Pain may also be due to stretching or pinching of noncontractile (inert) tissues, such as ligaments, joint capsules, bursa, fascia, and skin. Testing active ROM is a good screening technique to help focus a physical examination. If an individual can complete active ROM easily and painlessly, further testing of that motion probably is not needed. If, however, active ROM is limited, painful, or awkward, the physical examination should include an examination of passive ROM and additional testing to clarify the problem. **Active assistive ROM** is the arc of motion produced by the individual's muscle contraction assisted by an external force. During the examination process the external force is provided by the examiner. In other instances the external force may be provided by an unimpaired region of the individual's body, or by a mechanical device. Passive Range of Motion **Passive ROM** is the arc of motion produced by the application of an external force by the examiner. The individual remains relaxed and plays no active role in producing the motion. Normally, passive ROM is slightly greater than active ROM16--18 because each joint has a small amount of motion that is not under voluntary control. The additional passive ROM that is available at the end of the normal active ROM is due to the stretch of tissues surrounding Flexion to zero Extension from zero Extension to zero Flexion from zero **FIGURE 1.10** Flexion and extension of the shoulder begin with the shoulder in the anatomical position. The ROM in flexion proceeds anteriorly from the zero position through an arc toward 180 degrees. The long, bold arrow shows the ROM in flexion, which is measured in goniometry. The ROM in extension proceeds posteriorly from the zero position through an arc toward 180 degrees. The short, bold arrow shows the ROM in extension, which is measured in goniometry. CHAPTER 1 Basic Concepts 9 the joint and the reduced bulk of relaxed muscles compared with contracting muscles. This additional passive ROM helps to protect joint structures because it allows the joint to absorb extrinsic forces. Testing passive ROM provides the examiner with information about the integrity of the joint surfaces and the extensibility of the joint capsule and associated ligaments, muscles, fascia, and skin. Comparisons between passive ROM and active ROM provide information about the amount of motion permitted by the associated joint structures (passive ROM) relative to the individual's ability to produce motion at a joint (active ROM). In cases of impairment such as muscle weakness, passive ROM and active ROM may vary considerably. Example: An examiner may find that an individual with a muscle paralysis has full passive ROM but no active ROM at the same joint. In this instance, the joint surfaces and the extensibility of the joint capsule, ligaments, muscles, tendons, fascia, and skin are sufficient to allow full passive ROM. The lack of muscle strength prevents active motion at the joint. If pain occurs during passive ROM, it is often due to moving, stretching, or pinching of noncontractile (inert) structures. Pain occurring at the end of passive ROM may be due to stretching of contractile structures as well as noncontractile structures.19 Pain during passive ROM is not due to active shortening (contracting) of contractile tissues. By comparing which motions (active versus passive) cause pain and noting the location of the pain, the examiner can begin to determine which injured tissues are involved. Careful consideration of the end-feel and location of tissue tension and pain during passive ROM also adds information about structures that are limiting ROM. **End-Feel** The amount of passive ROM is determined by the unique structure of the joint being tested. Some joints are structured so that the joint capsules limit the end of the ROM in a particular direction, whereas other joints are structured so that ligaments limit the end of a particular ROM. Other normal limitations to motion include passive tension in soft tissue such as muscles, fascia, and skin; soft tissue approximation; and contact of joint surfaces. The type of structure that limits a ROM has a characteristic feel that may be detected by the examiner who is performing the passive ROM when slight overpressure is applied at the end of the motion. This feeling, which is experienced by an examiner as a barrier to further motion, is called the **endfeel.** 6,19,20 Developing the ability to determine the character of the end-feel requires practice and sensitivity. Determination of the end-feel must be carried out slowly and carefully to detect the end of the ROM and to distinguish among the various normal and abnormal end-feels. The ability to distinguish among the various end-feels helps the examiner identify the type of limiting structure. Cyriax,19 Kaltenborn,6 and Paris20 have described a variety of types of normal (physiological) and abnormal (pathological) end-feels. Table 1.2, which describes normal end-feels, and Table 1.3, which describes abnormal end-feels, have been adapted from the works of these authors but are most similar to those presented by Kaltenborn.6 Only recently have researchers begun to conduct studies to determine the validity and reliability of end-feels. Petersen and Hayes investigated Cyriax's theory that abnormal endfeels are significantly more painful than normal end-feels. The authors found partial confirmation of Cyriax's theory in that some abnormal end-feels were significantly more painful than normal end-feels at the two joints (knee and shoulder) included in their study.21 Hayes and Petersen found that, generally, end-feel identification reliability was considered to be good when the same examiner made the identification of Cyriax's three normal and six abnormal end-feels at the knee and shoulder.22 However, the ability of different examiners to agree on the same end-feels was poor. Manning et al23 conducted a study to evaluate the reliability of end-feel identification, pain provocation, and hypomobility at each cervical joint from C2--C3 to C6--C7 in symptomatic individuals. Clinically acceptable reliability was found primarily for assessment of joint hypomobility and end-feel in the lower cervical disc segment of the less painful side but not in the more painful side. TABLE 1.2 Normal End-Feels End-Feel Description Example Soft Soft tissue approximation Knee flexion (contact between soft tissue of posterior leg and posterior thigh) Firm Muscular stretch Hip flexion with the knee straight (passive tension of hamstring muscles) Capsular stretch Extension of metacarpophalangeal joints of fingers (tension in the anterior capsule) Ligamentous stretch Forearm supination (tension in the palmar radioulnar ligament of the inferior radioulnar joint, interosseous membrane, oblique cord) Hard Bone contacting bone Elbow extension (contact between the olecranon process of the ulna and the olecranon fossa of the humerus) 10 PART I Introduction to Goniometry and Muscle Length Testing In Chapters 4 through 11, we describe what we believe are the normal end-feels and the structures that limit the ROM for each joint and motion. Because of the paucity of specific literature in this area, these descriptions are based on our experience in evaluating joint motion and on information obtained from established anatomy7,24--28 and biomechanics texts.29,30 Controversy exists among experts concerning the structures that limit the ROM in some parts of the body. Normal individual variations in body structure may also cause instances in which the end-feel differs from our description. Examiners should practice trying to distinguish among the different types of end-feels. **Exercise 1 in Chapter 2** is included for this purpose. Hypomobility The term **hypomobility** refers to a decrease in ROM that is substantially less than normal values for that joint, given the individual's age and gender. For example, the end-feel occurs early in the ROM and may be different in quality from what is expected. This limitation in passive ROM may be due to a variety of causes, including abnormalities of the joint surfaces; passive shortening of joint capsules, ligaments, muscles, fascia, and skin; and inflammation of these structures. Hypomobility has been associated with many orthopedic conditions such as osteoarthritis,31 spinal disorders,32 and metabolic disorders such as diabetes.33,34 Decreased ROM is also a common consequence of immobilization after fractures and scar development after burns.35,36 Neurological conditions such as stroke, head trauma, cerebral palsy, and complex regional pain syndrome can result in hypomobility owing to loss of voluntary movement, increased muscle tone, immobilization, and pain. Hypomobility also has been shown to impair function in the hand37 and the ankle.38 **Capsular Patterns of Restricted Motion** Cyriax19 proposed that pathological conditions involving the entire joint capsule cause a particular pattern of restriction involving all or most of the passive motions of the joint. This pattern of restriction is called a **capsular pattern.** The restrictions do not involve a fixed number of degrees for each motion but rather a fixed proportion of one motion relative to another motion. Example: The capsular pattern for the elbow joint is a greater limitation of flexion than of extension. The elbow joint normally has a passive flexion ROM of 0 to 150 degrees. If the capsular involvement is mild, the last 15 degrees of flexion and the last 5 degrees of extension might be restricted so that passive ROM is 5 to135 degrees. If the capsular involvement is more severe, the last 30 degrees of flexion and the last 10 degrees of extension might be restricted so that the passive ROM is 10 to120 degrees. Capsular patterns vary from joint to joint (Table 1.4). The capsular patterns for each joint, as presented by Cyriax19 and Kaltenborn,6 are noted in the beginning of Chapters 4 through 10. Additional studies are needed to test the hypotheses regarding the cause of capsular patterns and to determine the capsular pattern for each joint. Several studies21,39--41 have examined the construct validity of Cyriax's capsular pattern in individuals with arthritis or arthrosis of the knee. Although differing opinions exist, the findings seem to support the concept of a capsular pattern of restriction for the knee but with more liberal interpretation of the proportions of limitation than suggested by Cyriax.19 Two studies41,42 examining capsular patterns for the hip found decreases in all hip motions in osteoarthritic TABLE 1.3 Abnormal End-Feels End-Feel Description Example Soft Occurs sooner or later in the ROM than is usual or in a joint that normally has a firm or hard end-feel. Feels boggy. Soft tissue edema Synovitis Firm Occurs sooner or later in the ROM than is usual or in a joint that normally has a soft or hard end-feel. Increased muscular tonus Capsular, muscular, ligamentous, and fascial shortening Hard Occurs sooner or later in the ROM than is usual or in a joint that normally has a soft or firm end-feel. A bony grating or bony block is felt. Chondromalacia Osteoarthritis Loose bone fragments in joint Myositis ossificans Fracture Empty No real end-feel because pain prevents reaching end of ROM. No resistance is felt except for individual's protective muscle splinting or muscle spasm. Acute joint inflammation Bursitis Abscess Fracture Psychogenic disorder CHAPTER 1 Basic Concepts 11 hips as compared with nonosteoarthritic hips but raised questions concerning specific patterns of limitation proposed by Kaltenborn6 and Cyriax.19 Hertling and Kessler43 have extended Cyriax's concepts on causes of capsular patterns. They suggest that conditions resulting in a capsular pattern of restriction can be classified into two general categories: 1\. Conditions in which there is considerable joint effusion or synovial inflammation 2\. Conditions in which there is relative capsular fibrosis Joint effusion and synovial inflammation accompany conditions such as traumatic arthritis, infectious arthritis, acute rheumatoid arthritis, and gout. In these conditions, the joint capsule is distended by excessive intra-articular synovial fluid, causing the joint to maintain a position that allows the greatest intra-articular joint volume. Pain triggered by stretching the capsule and muscle spasms that protect the capsule from further insult inhibit movement and cause a capsular pattern of restriction. Relative capsular fibrosis often occurs during chronic low-grade capsular inflammation, immobilization of a joint, and resolution of acute capsular inflammation. These conditions increase the relative proportion of collagen compared with that of mucopolysaccharide in the joint capsule or they change the structure of the collagen. The resulting decrease in extensibility of the entire capsule causes a capsular pattern of restriction. TABLE 1.4 Capsular Pattern of Extremity Joints Joint Restricted Motions Glenohumeral joint Greatest loss of lateral rotation, moderate loss of abduction, minimal loss of medial rotation Elbow complex (humeroulnar, humeroradial, proximal radioulnar joints) Loss of flexion greater than loss of extension; rotations full and painless except in advanced cases Forearm (proximal and distal radioulnar joints) Equal loss of supination and pronation, only occurring if elbow has marked restrictions of flexion and extension Wrist (radiocarpal and midcarpal joints) Equal loss of flexion and extension, slight loss of ulnar and radial deviation (Cyriax) Equal loss of all motions (Kaltenborn) Hand Carpometacarpal joint---digit 1 Loss of abduction (Cyriax); loss of abduction greater than extension (Kaltenborn) Carpometacarpal joint---digits 2--5 Equal loss of all motions Metacarpophalangeal and interphalangeal joints Equal loss of flexion and extension (Cyriax) Restricted in all motions, but loss of flexion greater than loss of other motions (Kaltenborn) Hip Greatest loss of medial rotation and flexion, some loss of abduction, slight loss of extension; little or no loss of adduction and lateral rotation (Cyriax) Greatest loss of medial rotation, followed by less restriction of extension, abduction, flexion, and lateral rotation (Kaltenborn) Knee (tibiofemoral joint) Loss of flexion greater than extension Ankle (talocrural joint) Loss of plantarflexion greater than dorsiflexion Subtalar joint Loss of inversion (varus) Midtarsal joint Loss of inversion (adduction and medial rotation); other motions full Foot Metatarsophalangeal joint---digit 1 Loss of extension greater than flexion Metatarsophalangeal joint---digits 2--5 Loss of flexion greater than extension Interphalangeal joints Loss of extension greater than flexion Adapted from Dyrek, DA: Assessment and Treatment Planning Strategies for Musculoskeletal Deficits. In O'Sullivan, SB, and Schmitz, TJ (eds): Physical Rehabilitation: Assessment and Treatment, ed 3. FA Davis, Philadelphia, 1994. Capsular patterns are from Cyriax19 and Kaltenborn.6 12 PART I Introduction to Goniometry and Muscle Length Testing **Noncapsular Patterns of Restricted Motion** A limitation of passive motion that is not proportioned similarly to a capsular pattern is called a **noncapsular pattern** of restricted motion.19 A noncapsular pattern is usually caused by a condition involving structures other than the entire joint capsule. Internal joint derangement, adhesion of a part of a joint capsule, ligament shortening, muscle strains, and muscle contractures are examples of conditions that typically result in noncapsular patterns of restriction. Noncapsular patterns usually involve only one or two motions of a joint, in contrast to capsular patterns, which involve all or most motions of a joint.6,19 Example: A strain of the biceps muscle may result in pain and restriction at the end of the range of passive elbow extension. The passive motion of elbow flexion would not be affected. Hypermobility The term **hypermobility** refers to the ability of one or more joints to actively or passively move beyond normal limits given the individual's age and gender. For example, in adults the normal ROM for extension at the elbow joint is about 0 degrees.11,12 A ROM measurement of 30 degrees or more of extension at the elbow is well beyond normal ROM and is indicative of a hypermobile joint in an adult. Children have some normally occurring specific instances of increased ROM compared with adults. For example, neonates 6 to 72 hours old have been found to have a mean ankle dorsiflexion passive ROM of 59 degrees,44 which contrasts with mean adult ROM values of between 12 and 20 degrees.11,12 The increased motion that is present in these children is normal for their age. If the increased motion persists beyond the expected age range, it would be considered abnormal and hypermobility would be present. Hypermobility is due to the laxity of soft tissue structures such as ligaments, capsules, and muscles that normally prevent excessive motion at a joint. In some instances, the hypermobility may be due to abnormalities of the joint surfaces. A frequent cause of hypermobility is trauma to a joint. Hypermobility also occurs in serious hereditary disorders of connective tissue such as Marfan syndrome, rheumatic diseases, osteogenesis imperfecta, and Ehlers-Danlos syndrome. Research involving Ehlers-Danlos syndrome has found that in addition to joint hypermobility and widespread musculoskeletal pain, the syndrome involves all of the major systems of the body.45 Hypermobility syndrome (HMS) or benign joint hypermobility syndrome (BJHS) is used to describe otherwise healthy individuals who have generalized hypermobility accompanied by musculoskeletal symptoms.46,47 An inherited abnormality in collagen and regular physical exercise are thought to be responsible for the joint laxity in these individuals. 48--50 Traditionally, the diagnosis of HMS involves the exclusion of other conditions, a score of at least 4 on the Beighton score (Table 1.5), and arthralgia for longer than 3 months in four or more joints.51 Some researchers have noted that these criteria are inadequate for children because scores greater than 4 on the Beighton scale were found in 65% of a sample of 1,120 children aged 4 to 7 years in Brazil.50 Jelsma and colleagues53 also found an extremely high prevalence of hypermobility when they applied the cutoff score of 5 in children ages 3 to 9 years and a score of 4 in the 10 to 16 years age-group; they suggested that a cutoff of 7 would be more appropriate. The authors also stressed the need for international agreement on firm cutoff points and the use of standardized measurement for Beighton mobility tasks.51 Other criteria have also been proposed, including additional joint motions and extra-articular signs.52,53 According to Grahame,48 the following joint motions should also be considered: shoulder lateral rotation greater than 90 degrees, cervical spine lateral flexion greater than 60 degrees, distal interphalangeal joint hyperextension greater than 60 degrees, and first metatarsophalangeal joint extension greater than 90 degrees. In addition to Grahame's findings, Smith, Jermane, and Easton,47 in a systematic review of studies involving BJHS, found evidence to suggest that people with the syndrome have significantly poor joint position sense compared with people without BJHS. Smits- Engelsman, Klerks, and Kirby54 conducted a prospective study of 551 Dutch children aged 6 to 12 years to evaluate the validity of the Beighton score as a generalized measure of hypermobility. Qualified physical therapists assessed the children using goniometry to measure passive ROM. More TABLE 1.5 Beighton Hypermobility Score The Ability to Points Passively appose thumb to forearm Right 1 Left 1 Passively extend fifth MCP joint more than 90 degrees Right 1 Left 1 Hyperextend elbow more than 10 degrees Right 1 Left 1 Hyperextend knee more than 10 degrees Right 1 Left 1 Place palms on floor by flexing trunk with knees straight 1 Total Beighton Score = sum of points. 0--9 Adapted from Beighton, P, Solomon, L, and Soskolne, CL: Articular mobility in an African population. Ann Rheum Dis 32:23, 1973. CHAPTER 1 Basic Concepts 13 than 35% of children scored greater than 5/9 on the Beighton score. The authors concluded that when goniometry is used, the Beighton score is a valid instrument to measure generalized joint mobility in children aged 8 to 12 years and that additional items to improve the score, as suggested by Grahame,48 are not needed. Factors Affecting Range of Motion Range of motion varies among individuals and is influenced by factors such as age, gender, and whether the motion is performed actively or passively. A fairly extensive amount of research on the effects of age and gender on ROM has been conducted for the upper and lower extremities as well as the spine. Other factors relating to characteristics such as body mass index, occupational activities, and recreational activities may affect ROM but have not been as extensively researched as age and gender. In addition, factors relating to the testing process, such as the testing position, type of instrument employed, experience of the examiner, and even time of day, have been identified as affecting ROM measurements. A brief summary of research findings that examine age and gender effects on ROM is presented in this introductory chapter. To assist the examiner, more detailed information about the effects of age and gender on the featured joints is presented at the end of Chapters 4 through 13. Information on the effects of characteristics and the testing process is included if available. Ideally, to determine whether a ROM is impaired, the value of the ROM of the joint under consideration should be compared with ROM values from people of the same age and gender, and from studies that used the same method of measurement. Often such comparisons are not possible because age-related and gender-related norms have not been established for all groups. In such situations, the ROM of the joint should be compared with the same joint of the individual's contralateral extremity, providing that the contralateral extremity is not impaired or used selectively in athletic or occupational activities. Most studies have found little difference between the ROM of the right and left extremities.54--57 A few studies17,59,60 have found slightly less ROM in some joints of the upper extremity on the dominant or right side compared with the contralateral side, which Allender and coworkers58 attribute to increased exposure to stress. If the contralateral extremity is inappropriate for comparison, the individual's ROM may be compared with average ROM values in handbooks of the American Academy of Orthopaedic Surgeons11,12 and other standard texts.1,3,7,60,61 However, in some of these texts, the populations from which the values were derived as well as the testing positions and type of measuring instruments used are not identified. Mean ROM values published in several standard texts and research studies are summarized at the beginning of the Range of Motion Testing Procedures for each motion and in tables at the end of Chapters 4 through 13. The ROM values presented should serve as only a general guide to identifying normal versus impaired ROM. Considerable differences in mean ROM values are sometimes noted between the various references. **Age** Numerous studies have been conducted to determine the effects of age on ROM of the extremities and spine. General agreement exists among investigators regarding the age- related effects on the ROM of the extremity joints of newborns, infants, and young children up to about 2 years of age.44,62--66 These age effects are joint and motion specific but do not seem to be affected by gender; both males and females are affected similarly. The youngest age-groups have more hip flexion, hip abduction, hip lateral rotation, ankle dorsiflexion, and elbow motion compared with adults. Limitations in hip extension, knee extension, and plantar flexion are considered to be normal for these youngest age-groups. Mean values for these age-groups differ by more than 2 standard deviations from mean values for adults published by the American Academy of Orthopaedic Surgeons,12 and the American Medical Association.1 Therefore, age-appropriate norms should be used whenever possible for newborns, infants, and young children up to 2 years of age. Most investigators who have studied a wide range of agegroups have found that older adult groups have somewhat less ROM of the extremities than younger adult groups. These age-related changes in the ROM of older adults also are joint and motion specific and may affect males and females differently. Allender and associates58 found that wrist flexion-- extension, hip rotation, and shoulder rotation ROM decreased with increasing age, whereas flexion ROM in the metacarpophalangeal (MCP) joint of the thumb showed no consistent loss of motion. Roach and Miles67 generally found a small decrease (3 to 5 degrees) in mean active hip and knee motions between the youngest age-group (25 to 39 years) and the oldest age-group (60 to 74 years). Except for hip extension ROM, these decreases represented less than 15% of the arc of motion. Stubbs, Fernandez, and Glenn69 found a decrease of between 4% and 30% in 11 of 23 joints studied in men between the ages of 25 and 54 years. James and Parker16 found systematic decreases in 10 active and passive lower-extremity motions in individuals who were between 70 and 92 years of age. Steinberg and associates68 in a study of dancers and nondancers of the same ages (8 to 16 years) found that age differences not only occurred in different joints and motions but also varied with activity. For example, hip flexion and internal rotation and knee flexion ROM decreased with increasing age in both groups, but ankle plantar flexion and hip external rotation decreased with increasing age in nondancers and did not change in dancers. As with the extremities, age-related effects on spinal ROM appear to be motion specific. Youdas and associates75 found that with each decade, both females and males lose approximately 5 degrees of active motion in neck extension and 3 degrees in flexion, lateral flexion, and rotation. Chen and colleagues,76 in a review of the literature regarding the effects of aging on cervical spine ROM, concluded that active cervical ROM decreased by 4 degrees per decade, which is 14 PART I Introduction to Goniometry and Muscle Length Testing similar to the findings of Youdas and associates. Salo and colleagues77 in a study of 220 healthy women aged 20 to 59 years found that passive ROM of the cervical spine decreased with increasing age in all motions except forward flexion. Lansade and associates,74 using the noninvasive infrared polaris system to investigate the effects of age on cervical ROM, found less of a decrease (only 0.55 to 0.79 degree) per decade between 20 and 93 years. Investigators have reached varying conclusions regarding how large a decrease in ROM occurs with increasing age in the thoracolumbar spine. Loebl78 found that thoracolumbar spinal mobility (flexion--extension) decreases with age an average of 8 degrees per decade. Fitzgerald and colleagues79 found a systematic decrease in lateral flexion and extension of the lumbar spine at 20-year intervals but no differences in rotation and forward flexion. In contrast to Fitzgerald, Intolo and colleagues80 in a systematic review and meta-analysis to determine the effect of age on lumbar range of motion found 16 studies with results that showed age-related reductions in flexion, extension, and lateral flexion occurred primarily from 40 to 50 years and after 60 years of age. There was little evidence of age effects on lumbar rotation. Trudelle-Jackson and associates81 compared measurements of lumbar spine flexion and extension in a group of white and African American women between 20 and 83 years. Flexion and extension ROM in the young group (aged 20 to 39) was significantly greater than in the middle group (aged 40 to 59) and in the older group (aged 60 plus). In addition, the difference in extension ROM between the middle and older groups was also significant, but this difference was not significant for flexion ROM. Decreases in lumbar flexion ranged from 2.4 to 7.3 degrees, whereas differences in extension ranged from 4.9 degrees to 10.8 degrees. Extension and flexion showed a decreasing trend with increasing age in both racial groups. **Gender** The effects of gender on the ROM of the extremities and spine also appear to be joint and motion specific. If gender differences in the amount of ROM are found, females are more often reported to have slightly greater ROM than males. In general, gender differences appear to be more prevalent in adults than in young children. Bell and Hoshizaki71 found that females across an age range of 18 to 88 years had more flexibility than males in 14 of 17 joint motions tested. Beighton, Solomon, and Soskolne51 in a study of an African population found that females between 0 and 80 years of age were more mobile than their male counterparts. Walker and coworkers70 in a study of 28 joint motions in 60- to 84-year-olds reported that 8 motions were greater in females and 4 motions were greater in males, whereas the other motions showed little gender difference. Almquist and colleagues73 found that women had 10% to 20% greater knee ROM than men in all age-groups between 15 and 60 plus years. Kalscheur and associates72 measured 24 upper-extremity and cervical motions in men and women between the ages of 63 and 86 years. Gender differences were noted for 14 of the motions, and in all cases the older women had greater active ROM than the older men. Lansade74 and associates found that gender had no significant influence on three-dimensional cervical range of motion except in the 70- to 79-year-old group. Muscle Length Testing Maximal **muscle length** is the greatest extensibility of a muscle-tendon unit.5 It is the maximal distance between the proximal and the distal attachments of a muscle to bone. Clinically, muscle length is not measured directly; instead, it is measured indirectly by determining the maximal passive ROM of the joint(s) crossed by the muscle.82--85 Muscle length, in addition to the integrity of the joint surfaces and the extensibility of the capsule, ligaments, fascia, and skin, affects the amount of passive ROM of a joint. The purpose of testing muscle length is to ascertain whether hypomobility or hypermobility is caused by the length of the inactive antagonist muscle or other structures. By ascertaining which structures are involved, the health professional can choose more specific and more effective treatment procedures. Muscles can be categorized by the number of joints they cross from their proximal to their distal attachments. **Onejoint** **muscles** cross and therefore influence the motion of only one joint. **Two-joint muscles** cross and influence the motion of two joints, whereas **multi-joint muscles** cross and influence multiple joints. No difference exists between the measurement of the length of a one-joint muscle and the measurement of passive joint ROM in the direction opposite to the muscle's active motion. Usually, one-joint muscles have sufficient length to allow full passive ROM at the joint they cross. If a one-joint muscle is shorter than normal, passive ROM in the direction opposite to the muscle's action is decreased and the end-feel is firm owing to a muscular stretch. At the end of the ROM, the examiner may be able to palpate tension within the muscle-tendon unit if the structures are superficial. In addition, the individual may complain of pain in the region of the tight muscle and tendon. These signs and symptoms help to confirm muscle shortness as the cause of the joint limitation. If a one-joint muscle is abnormally lax, passive tension in the capsule and ligaments may initially maintain a normal ROM. However, with time, these joint structures often lengthen as well and passive ROM at the joint increases. Because the indirect measurement of the length of one-joint muscles is the same as the measurement of passive joint ROM, we have not presented specific muscle length tests for one-joint muscles. Example: The length of one-joint hip adductors such as the adductor longus, adductor magnus, and adductor brevis is assessed by measuring passive hip abduction ROM. The indirect measurement of the length of the hip adductor muscles is identical to the measurement of passive hip abduction ROM (Fig. 1.11). CHAPTER 1 Basic Concepts 15 **FIGURE 1.11** The indirect measurement of the muscle length of one-joint hip adductors is the same as measurement of passive hip abduction ROM. In contrast to one-joint muscles, the length of two-joint and multi-joint muscles is usually not sufficient to allow full passive ROM to occur simultaneously at all joints crossed by these muscles. This inability of a muscle to lengthen and allow full ROM at all of the joints the muscle crosses is termed **passive** **insufficiency.** If a two-joint or multi-joint muscle crosses a joint that the examiner is assessing for ROM, the individual must be positioned so that passive tension in the muscle does not limit the joint's ROM. To allow full ROM at the joint under consideration and to ensure sufficient length in the muscle, the muscle must be put on slack at all of the joints the muscle crosses that are not being assessed. A muscle is put on slack by passively approximating the origin and insertion of the muscle. Example: The triceps is a two-joint muscle that extends the elbow and shoulder. The triceps is passively insufficient during full shoulder flexion and full elbow flexion. When an examiner assesses elbow flexion ROM, the shoulder must be in a neutral position so there is sufficient length in the triceps to allow full flexion at the elbow (Fig. 1.12). **FIGURE 1.12** During the measurement of elbow flexion ROM, the shoulder must be in neutral to avoid passive insufficiency of the triceps, which would limit the ROM. 16 PART I Introduction to Goniometry and Muscle Length Testing of the muscle length tests and information on the reliability and validity of these tests, if available, are presented as well. In the next chapter, the examiner will have an opportunity to learn about the various instruments used to measure joint motion as well as participate in exercises designed to assist in identifying end-feels. Additional exercises are provided to assist the examiner in developing the skills that are necessary to use the instruments and record a ROM examination. **FIGURE 1.13** To assess the length of the two-joint triceps muscle, elbow flexion is measured while the shoulder is positioned in flexion. To assess the length of a two-joint muscle, the individual is positioned so that the muscle is lengthened over the proximal and distal joints that the muscle crosses. One joint is held in a full ROM position while the examiner attempts to further lengthen the muscle by moving the second joint through full ROM. The end-feel in this situation is firm owing to the development of passive tension in the stretched muscle. The length of the two-joint muscle is indirectly assessed by measuring the passive ROM in the direction opposite to the muscle's action at the second joint. Example: To assess the length of a two-joint muscle such as the triceps, the shoulder is positioned and held in full flexion. The elbow is flexed until tension is felt in the triceps, creating a firm end-feel. The length of the triceps is determined by measuring passive ROM of elbow flexion with the shoulder in flexion (Fig. 1.13). The length of multi-joint muscles is assessed in a manner similar to that used in assessing the length of two-joint muscles. However, the individual is positioned and held so that the muscle is lengthened over all of the joints that the muscle crosses except for one last joint. The examiner attempts to further lengthen the muscle by moving the last joint through full ROM. Again, the end-feel is firm owing to tension in the stretched muscle. The length of the multi-joint muscle is determined by measuring passive ROM in the direction opposite to the muscle's action at the last joint to be moved. Commonly used muscle length tests that indirectly assess two-joint and multi-joint muscles have been included in Chapters 4 through 10 as appropriate. Normative values on joint angle at the end R E F E R E N C E S 1\. Rondinelli, RD (ed): Guides to the Evaluation of Permanent Impairment, ed 6. American Medical Association Chicago, 2008. 2\. Ward, SR: Biomechanical Applications to Joint Structure and Function. In Levangie, P, and Norkin, C (eds): Joint Structure and Function: A Comprehensive Analysis, ed 5. FA Davis, Philadelphia, 2011. 3\. MacConaill, MA, and Basmajian, JV: Muscles and Movement: A Basis for Human Kinesiology, ed 2. Robert E. Krieger, New York, 1977. 4\. Kisner, C, and Colby, LA: Therapeutic Exercise, ed 5. FA Davis, Philadelphia, 2007. 5\. White, DJ: Musculoskeletal Examination. In O'Sullivan, SB, Schmitz, TJ, and Fulk, GD (eds): Physical Rehabilitation, ed 6. FA Davis, Philadelphia, 2014. 6\. Kaltenborn, FM: Manual Mobilization of the Extremity Joints, ed 5. Olaf Norlis Bokhandel, Oslo, 1999. 7\. Standring, S (ed): Gray's Anatomy, ed 41. Elsevier, New York, 2015. 8\. Silver, D: Measurement of the range of motion in joints. J Bone Joint Surg 21:569, 1923. 9\. Cave, EF, and Roberts, SM: A method for measuring and recording joint function. J Bone Joint Surg 18:455, 1936. 10\. Moore, ML: The measurement of joint motion. Part II: The technic of goniometry. Phys Ther Rev 29:256, 1949. 11\. American Academy of Orthopaedic Surgeons: Joint Motion: Methods of Measuring and Recording. AAOS, Chicago, 1965. 12\. Greene, WB, and Heckman, JD (eds): The Clinical Measurement of Joint Motion. American Academy of Orthopaedic Surgeons, Rosemont, IL, 1994. 13\. Clark, WA: A system of joint measurement. J Orthop Surg 2:687, 1920. 14\. West, CC: Measurement of joint motion. Arch Phys Med Rehabil 26:414, 1945. 15\. Cole, TM, and Tobis, JS: Measurement of Musculoskeletal Function. In Kottke, FJ, and Lehmann, JF (eds): Krusenn's Handbook of Physical Medicine and Rehabilitation, ed 4. WB Saunders, Philadelphia, 1990. 16\. James, B, and Parker, AW: Active and passive mobility of lower limb joints in elderly men and women. Am J Phys Med Rehabil 68:162, 1989. 17\. Gunal, I, et al: Normal range of motion of the joints of the upper extremity in male subjects, with special reference to side. J Bone Joint Surg Am 78(A):1401, 1996. 18\. Smahel, Z, and Klimova, A: The infl uence of age and exercise on the mobility of hand joints: 1: Metacarpophalangeal joints of the three-phalangeal fi ngers. Acta Chirurgiae Plasricae 46:81, 2004. 19\. Cyriax, J: Textbook of Orthopaedic Medicine: Diagnosis of Soft Tissue Lesions, ed 8. Bailliere Tindall, London, 1982. 20\. Paris, SV: Extremity Dysfunction and Mobilization. Institute Press, Atlanta, 1980. 21\. Petersen, CM, and Hayes, KW: Construct validity of Cyriax's selective tension examination. Association of end-feels with pain at the knee and shoulder. J Orthop Sports Phys Ther 30:512, 2000. 22\. Hayes, KW, and Petersen, CM: Reliability of assessing end-feel and pain and resistance sequence in subjects with painful shoulders and knees. J Orthop Sports Phys Ther 31(8):432--445, 2001. 23\. Manning, DM, et al: Reliability of a seated three-dimensional passive intervertebral motion test for mobility, end-feel, and pain provocation patients with cervicalgia. J Man Manip Ther 3:135, 2012. 24\. Moore, KL, and Dalley, AF: Clinically Oriented Anatomy, ed 5. Lippincott, Williams & Wilkins, Baltimore, 2005. 25\. Kapandji, IA: Physiology of the Joints, Vol 1, ed 2. Churchill Livingstone, London, 1970. 26\. Kapandji, IA: Physiology of the Joints, Vol 2, ed 2. Williams & Wilkins, Baltimore, 1970. 27\. Kapandji, IA: Physiology of the Joints, Vol 3, ed 2. Churchill Livingstone, London, 1970. 28\. Steindler, A: Kinesiology of the Human Body. Charles C. Thomas, Springfi eld, IL, 1955. 29\. Levangie, PL, and Norkin, CC: Joint Structure and Function: A Comprehensive Analysis, ed 5. FA Davis, Philadelphia, 2011. 30\. Newmann, DA: Kinesiology of the Musculoskeletal System. Mosby, St. Louis, 2010. 31\. Steultjens, MPM, et al: Range of joint motion and disability in patients with osteoarthritis of the knee or hip. Rheumatology 39:955, 2000. 32\. Hermann, KM, and Reese, CS: Relationship among selected measures of impairment, functional limitation, and disability in patients with cervical spine disorders. Phys Ther 81:903, 2000. 33\. Rao, SR, et al: Increased passive ankle stiffness and reduced dorsifl exion range of motion in individuals with diabetes mellitus. Foot Ankle Int 27:617, 2006. 34\. Sauseng, S, Kastenbauer, T, and Irsigler, K: Limited joint mobility in selected hand and foot joints in patients with type 1 diabetes mellitus: A methodology comparison. Diabetes Nutr Metab 15:1, 2002. 35\. Richard, RL, and Ward, RS: Burns. In O'Sullivan, SB, and Schmitz, TJ (eds): Physical Rehabilitation: Assessment and Treatment, ed 5. FA Davis, Philadelphia, 2014. 36\. Johnson, J, and Silverberg, R: Serial casting of the lower extremity to correct contractures during the acute phase of burn care. Phys Ther 75:262, 1995. 37\. deSouza Campos, C, Jr, Bracht, MA, and Dos Santos, MJ: The effect of joint hypomobility on precision grip force. J Hand Ther 26(4):323, 2013. 38\. Beazell, JR, et al: Treatment of lateral knee pain in addressing tibiofi bular hypomobility in a recreational runner. N Am J Sports Phys Ther 4(1):21, 2009. 39\. Fritz, JM, et al: An examination of the selective tissue tension scheme, with evidence for the concept of a capsular pattern of the knee. Phys Ther 78:1046, 1998. 40\. Hayes, KW, Petersen, C, and Falconer, J: An examination of Cyriax's passive motion tests with patients having osteoarthritis of the knee. Phys Ther 74:697, 1994. 41\. Biji, D, et al: Validity of Cyriax's concept capsular pattern for the diagnosis of osteoarthritis of hip and/or knee. Scand J Rheumatol 27:347, 1998. 42\. Klassbo, M, and Harms-Ringdahl, K: Examination of passive ROM and capsular pattern in the hip. Physiother Res Int 8:1, 2003. 43\. Hertling, DH, and Kessler, RM: Management of Common Musculoskeletal Disorders, ed 4. Lippincott, Williams & Wilkins, Philadelphia, 2005. 44\. Waugh, KG, et al: Measurement of selected hip, knee and ankle joint motions in newborns. Phys Ther 63:1616, 1983. 45\. Castori, M: Ehler-Danlos syndrome, hypermobility type: An underdiagnosed hereditary connective tissue disorder with mucocutaneous, articular and systemic manifestations. G Ital Dermatol Venereol 1:13, 2013. 46\. Everman, DB, and Robin, NH: Hypermobility syndrome. Pediatr Rev 19:111, 1998. 47\. Smith, TO, et al.: Do people with benign joint hypermobility syndrome (BJHS) have reduced proprioception? A systematic review and meta-analysis. Rheumatol Int 33(11):2709, 2013. 48\. Grahame, R: Hypermobility not a circus act. Int J Clin Pract 54:314, 2000. 49\. Russek, LN: Hypermobility syndrome. Phys Ther 79:59, 1999. 50\. Lamari, NM, Chueire, AG, and Cordeiro, JA: Analysis of joint mobility patterns among preschool children. Sao Paulo Med J 123:119, 2005. 51\. Beighton, P, Solomon, L, and Soskolne, CL: Articular mobility in an African population. Ann Rheum Dis 32:23, 1973. 52\. Remvig, L, Jensen, DV, and Ward, RC: Are diagnostic criteria for general joint hypermobility and benign joint hypermobility syndrome based on reproducible and valid tests? A review of the literature. J Rheumatol 34:798, 2007. 53\. Jelsma, LD, et al: The relationship between joint mobility and motor performance in children with and without the diagnosis of developmental coordination disorder. BMC Pediatr 13:35, 2013. 54\. Smits-Engelsman, B, Klerks, M, and Kirby, A: Beighton score: A valid measure for generalized hypermobility in children. J Pediatr 158(1):119, 2011. 55\. Stefanyshyn, DJ, and Ensberg, JR: Right to left differences in the ankle joint complex range of motion. Med Sci Sports Exerc 26:551, 1993. 56\. Mosley, AM, Crosbie, J, and Adams, R: Normative data for passive ankle plantar fl exion-dorsifl exion fl exibility. Clin Biomech 16:514, 2001. 57\. Escalanate, A, et al: Determinants of hip and knee fl exion range: Results from the San Antonio Longitudinal Study of Aging. Arthritis Care Res 12:8, 1999. 58\. Allender, E, et al: Normal range of joint movements in shoulder, hip, wrist and thumb with special reference to side: A comparison between two populations. Int J Epidemiol 3:253, 1974. 59\. Escalante, A, Lichtenstein, MJ, and Hazuda, HP: Determinants of shoulder and elbow fl exion range: Results from the San Antonio Longitudinal Study of Aging. Arthritis Care Res 12:277, 1999. 60\. Kendall, FP, et al: Muscles: Testing and Function With Posture and Pain, ed 5. Lippincott, Williams & Wilkins, Philadelphia, 2005. 61\. Reese, NB, and Bandy, WD: Joint Range of Motion and Muscle Length Testing. WB Saunders, Philadelphia, 2010. 18 PART I Introduction to Goniometry and Muscle Length Testing 62\. Drews, JE, Vraciu, JK, and Pellino, G: Range of motion of the joints of the lower extremities of newborns. Phys Occup Ther Pediatr 4:49, 1984. 63\. Phelps, E, Smith, LJ, and Hallum, A: Normal range of hip motion of infants between nine and 24 months of age. Dev Med Child Neurol 27:785, 1985. 64\. Wanatabe, H, et al: The range of joint motions of the extremities in healthy Japanese people: The differences according to age. Nippon Seikeigeka Gakkai Zasshi 53:275, 1979. Cited in Walker, JM: Musculoskeletal development: A review. Phys Ther 71:878, 1991. 65\. Schwarze, DJ, and Denton, JR: Normal values of neonatal limbs: An evaluation of 1000 neonates. J Pediatr Orthop 13:758, 1993. 66\. Broughton, NS, Wright, J, and Menelaus, MB: Range of knee motion in normal neonates. J Pediatr Orthop 13:263, 1993. 67\. Roach, KE, and Miles, TP: Normal hip and knee active range of motion: The relationship to age. Phys Ther 71:656, 1991. 68\. Steinberg, N, et al: Range of joint movement in female dancers and non-dancers aged 8--16 years: Anatomical and clinical implications. Am J Sports Med 34(5):814, 2006. 69\. Stubbs, NB, Fernandez, JE, and Glenn, WM: Normative data on joint ranges of motion for 25- to 54-year-old males. Int J Ind Ergon 12:265, 1993. 70\. Walker, JM, et al: Active mobility of the extremities in older subjects. Phys Ther 64:919, 1984. 71\. Bell, RD, and Hoshizaki, TB: Relationship of age and sex with range of motion: Seventeen joint actions in humans. Can J Appl Sport Sci 6:202, 1981. 72\. Kalscheur, JA, Costello, PS, and Emery, LJ: Gender differences in range of motion in older adults. Phys Occup Ther Geriatr 22:77, 2003. 73\. Almquist, PO, et al: Knee motion in healthy individuals related to age and gender. J Orthop Res 31(1):23, 2013. 74\. Lansade, C, et al: Three-dimensional analysis of the cervical spine kinematics: Effect of age and gender in healthy subjects. Spine 34(26):2900, 2009. 75\. Youdas, JW, et al: Normal range of motion of the cervical spine: An initial goniometric study. Phys Ther 72:770, 1992. 76\. Chen, J, et al: Meta-analysis of normative cervical motion. Spine 24:1571, 1999. 77\. Salo, PK, et al: Quantifying the effect of age on passive range of motion of the cervical spine in healthy working-age women. J Orthop Sports Phys Ther 39:478, 2009. 78\. Loebl, WY: Measurement of spinal posture and range of spinal movement. Ann Phys Med 9:103, 1967. 79\. Fitzgerald, GK, et al: Objective assessment with establishment of normal values for lumbar spinal range of motion. Phys Ther 63:1776, 1983. 80\. Intolo, P, et al: The effect of age on lumbar range of motion: A systematic review. Man Ther 14:596, 2009. 81\. Trudelle-Jackson, E, et al: Lumbar spine fl exion and extension extremes of motion in women of different age and racial groups. Spine 35(16):1539, 2010. 82\. Gajdosik, RL, et al: Comparison of four clinical tests for assessing hamstring muscle length. J Orthop Sports Phys Ther 18:614, 1993. 83\. Tardieu, G, Lespargot, A, and Tardieu, C: To what extent is the tibiacalcaneum angle a reliable measurement of the triceps surae length: Radiological correction of the torque-angle curve. Eur J Appl Physiol 37:163, 1977. 84\. Gajdosik, RL: Passive extensibility of skeletal muscle: Review of the literature with clinical implications. Clin Biomech 16:87, 2001. 85\. Gajdosik, RL, Hallett, JP, and Slaughter, LL: Passive insuffi ciency of two-joint shoulder muscles. Clin Biomech 9:377,