Why the Utility of Mathematical Entities is Unlike the Utility of Theoretical Entities PDF
Document Details
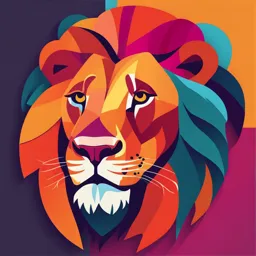
Uploaded by UndamagedRhodium3661
Tags
Related
- El plano y las rectas PDF
- Practice Questions - Mathematical Induction PDF
- CSIR Mathematical Sciences Past Paper February 2022 PDF
- Arjuna JEE (2025) Physics - Mathematical Tools PDF
- History of Extraterrestrial Life Debate PDF
- Science Without Numbers PDF - A Defense of Nominalism (2016, Oxford University Press)
Summary
This document explores the utility of mathematical entities in comparison to theoretical entities, highlighting their structural differences in the context of physics. It then discusses the usefulness of mathematical assertions in various scenarios. The document also covers nominalistic assertions and their relationship to mathematical theories.
Full Transcript
OUP CORRECTED PROOF – FINAL, //, SPi Why the Utility of Mathematical Entities is Unlike the Utility of Theoretical Entities No one can sensibly deny that the invocation of mathematical entities in some contexts is useful. The question arises as to whether the...
OUP CORRECTED PROOF – FINAL, //, SPi Why the Utility of Mathematical Entities is Unlike the Utility of Theoretical Entities No one can sensibly deny that the invocation of mathematical entities in some contexts is useful. The question arises as to whether the utility of mathematical existence-assertions gives us any grounds for believing that such existence-assertions are true. I claim that in answering this question one has to distinguish two different ways in which mathematical existence-assertions might be useful; I grant that if such assertions are useful in a certain respect, then that would indeed be evidence that they are true; but the most obvious respect in which mathematical existence assertions are useful is, I claim, quite a different one, and I will argue that the utility of such assertions in this respect gives no grounds whatever for believing the assertions to be true. To be more explicit, I will argue that the utility of mathematical entities is structurally disanalogous to the utility of theoretical entities in physics. The utility of theoretical entities lies in two facts: (a) they play a role in powerful theories from which we can deduce a wide range of phenomena; and (b) no alternative theories are known or seem at all likely which explain these phenomena without similar entities. [The unsympathetic reader may dispute (b): if any body of sentences counts as a ‘theory’ and any deduction from such a ‘theory’ counts as an explanation, then there clearly are alternatives to the usual theories of subatomic particles: e.g., take as your ‘theory’ the set T∗ of all the OUP CORRECTED PROOF – FINAL, //, SPi m at h e m at i c a l e n t i t i e s an d t h e oret i c a l e n t i t i e s consequences of T that don’t contain reference to subatomic particles (where T is one of the usual theories that does contain reference to sub- atomic particles); or if you want a recursively axiomatized ‘theory’, let T∗∗ be the Craigian reaxiomatization of the theory T∗ just described. Since I don’t know any formal conditions to impose which would rule out such bizarre trickery, let me simply say that by ‘theory’ I mean reasonably attractive theory; ‘theories’ like T∗ and T∗∗ are obviously uninteresting, since they do nothing whatever toward explaining the phenomena in question in terms of a small number of basic principles.] The upshot of (a) and (b) is that subatomic particles are theoretically indispensable; and I believe that that is as good an argument for their existence as we need. Now, later on in the monograph I will argue that mathematical entities are not theoretically indispensable: although they do play a role in the powerful theories of modern physics, we can give attractive reformulations of such theories in which mathematical entities play no role. If this is right, then we can safely adhere to a fictionalist view of mathematics, for adhering to such a view will not involve depriving ourselves of a theory that explains physical phenomena and which we can regard as literally true. But the task of arguing for the theoretical dispensability of mathemat- ical entities is a matter for later. What I want to do now is to give an account, consistent with the theoretical dispensability of mathematical entities, of why it is useful to make mathematical existence-assertions in certain contexts. The explanation of why mathematical entities are useful involves a fea- ture of mathematics that is not shared by physical theories that postulate unobservables. To put it a bit vaguely for the moment: if you take any body of nominalistically stated assertions N, and supplement it with a mathematical theory S, you don’t get any nominalistically stated con- clusions that you wouldn’t get from N alone. The analog for theories postulating subatomic particles is of course not true: if T is a theory that involves subatomic particles and is at all interesting, then there are going to be lots of cases of bodies P of wholly macroscopic assertions which in conjunction with T yield macroscopic conclusions that they don’t yield in absence of T; if this were not so, theories about subatomic particles could never be tested. I’ll state these claims more precisely in a moment, but first I should say that the claim about mathematics would be almost totally trivial if mathematics consisted only of theories like number theory or pure set OUP CORRECTED PROOF – FINAL, //, SPi m at h e m at i c a l e n t i t i e s an d t h e oret i c a l e n t i t i e s theory, i.e. set theory in which no allowance is made for sets with mem- bers that are not themselves sets. But these theories are by themselves of no interest from the point of view of applied mathematics, for there is no way to apply them to the physical world. That is, there is no way in which they are even prima facie helpful in enabling us to deduce nominalistically statable consequences from nominalistically statable premises. In order to be able to apply any postulated abstract entities to the physical world, we need impure abstract entities, e.g. functions that map physical objects into pure abstract entities. Such impure abstract entities serve as a bridge between the pure abstract entities and the physical objects; without the bridge, the pure objects would be idle. Consequently, if we regard func- tions as sets of a certain sort, then the mathematical theories we should be considering must include at least a minimal amount of impure set theory: set theory that allows for the possibility of urelements, where an urelement is a non-set which can be a member of sets. In fact, in order to be sufficiently powerful for most purposes, the impure set theory must differ from pure set theory not only in allowing for the possibility of urelements, it must also allow for non-mathematical vocabulary to appear in the comprehension axioms (i.e. in the instances of the axiom schema of separation or of replacement). So the ‘bridge laws’ must include laws that involve the mathematical vocabulary and the physical vocabulary together. Something rather analogous is true of the theory of subatomic particles. One can artificially formulate such a theory so that none of the non-logical vocabulary that is applied to observable physical objects is applied to the subatomic particles; in general it seems to me pointless to formulate physical theories in this way, but to press the analogy with the mathematical case as far as it will go, let us suppose it done. If it is done, and if we suppose that T is a physical theory stated entirely in this vocabulary, then of course, it will be the case that if we add T to a bunch of macroscopic assertions P, we will be able to derive no results about observables that weren’t derivable already. But that is for a wholly uninteresting reason: it is because the theory T by itself is not even prima facie helpful in deducing claims about observables from other claims about observables. In order to make it even prima facie helpful, we have to add ‘bridge laws’, laws which connect up the entities Count ‘=’ as logical. OUP CORRECTED PROOF – FINAL, //, SPi m at h e m at i c a l e n t i t i e s an d t h e oret i c a l e n t i t i e s and/or the vocabulary of the (artificially formulated) physical theory with observables and the properties by which we describe them. So far, then, like the mathematical case. But there is a fundamental difference between the two cases, and that difference lies in the nature of the bridge laws. In the case of subatomic particles, the theory T, interpreted now so as to include the bridge laws (and perhaps also some assumptions about initial conditions), can be applied to bodies of premises about observables in such a way as to yield genuinely new claims about observables, claims that would not be deriv- able without T. Whereas in the mathematical case the situation is very different. Here, if we take a mathematical theory that includes bridge laws (i.e. includes assertions of the existence of functions from physical objects into ‘pure’ abstract objects, including perhaps assertions obtained via a comprehension principle that uses mathematical and physical vocabulary in the same breath), then that mathematics is applicable to the world, i.e. it is useful in enabling us to draw nominalistically statable conclusions from nominalistically statable premises. But here, unlike in the case of physics, the conclusions we arrive at by these means are not genuinely new, they are already derivable in a more long-winded fashion from the premises, without recourse to the mathematical entities. This claim, unlike the one I will make later about the theoretical dis- pensability of mathematical entities, is pretty much of an incontrovertible fact, but one very much worth emphasizing. So first let me state the point more precisely than I have done. A first stab at putting the point precisely would be to say that for any mathematical theory S and any body of nominalistic assertions N, N + S is a conservative extension of N. However, this formulation isn’t quite right, and it is worth taking the trouble to put the point accurately. The problem with this formulation is that since N is a nominalistic theory, it may say things that rule out the existence of abstract entities, and so N + S may well be inconsistent. But it is clear how to deal with this: first, introduce a -place predicate ‘M(x)’, meaning intuitively ‘x is a mathematical entity’; second, for any nominalistically stated assertion A, let A∗ be the assertion that results by restricting each quantifier of A with the formula ‘not M(xi )’ (for the appropriate variable ‘xi ’); and third, That is, replace every quantification of form ‘∀x (...)’ by ‘∀x (if not M(x ) then...)’, i i i and every quantification of form ‘∃xi (...)’ by ‘∃xi (not M(xi ) and...)’. OUP CORRECTED PROOF – FINAL, //, SPi m at h e m at i c a l e n t i t i e s an d t h e oret i c a l e n t i t i e s for any nominalistically stated body of assertions N, let N∗ consist of all assertions A∗ for A in N. N∗ is then an ‘agnostic’ version of N: for instance, if N says that all objects obey Newton’s laws, then N∗ says that all non- mathematical objects obey Newton’s laws, but it allows for the possibility that there are mathematical objects that don’t. (Actually N∗ is in one respect too agnostic: in ordinary logic we assume for convenience that there is at least one thing in the universe, and in the context of a theory like N this means that there is at least one non-mathematical thing. So it is really N∗ + ‘∃x¬M(x)’ that gives the agnostic content of N.) Whether a similar point needs to be made for our mathematical theory S depends on what we take S to be. If S is simply set theory allowing for urelements, no restriction on the variables is needed, since the theory already purports to be about non-sets as well as sets: we merely need to connect up the notion of set that occurs in it with our predicate ‘M’, by adding the axiom ‘∀x(Set(x) → M(x))’. If in addition the mathematical theory includes portions like number theory, considered as independent disciplines unreduced to set theory, then we must restrict all variables in them by a new predicate ‘Number’, and add the axioms ‘∀x(Number(x) → M(x))’ and ‘∃x(Number(x))’. Presumably, however, everyone agrees that mathematical theories really ought to be written in this way (that is, presumably no one believes that all entities are mathematical), so I will not introduce a special notation for the modified version of S, I’ll assume that S is written in this form from the start. (The analogous assumption for N would be inappropriate: the nominalist wants to assert not N∗ , but the stronger claim N.) Having dealt with these tedious points, I can now state accurately the claim made at the end of the next to last paragraph. Principle C (for ‘conservative’): Let A be any nominalistically statable assertion, and N any body of such assertions; and The formal content of saying that N is ‘nominalistically statable’ is simply that it not employ the special mathematical vocabulary of the mathematical theory to be introduced, including ‘mathematical’. This is all we need to build into ‘nominalistically statable’ in order for Principle C to be true. For Principle C to be of interest, we must suppose in addition that the intended ontology of N does not include any entities in the intended extension of the predicate ‘M’ of S; for if this condition were violated, then N∗ + S would not correspond to the ‘intended’ way of combining N and S. [This footnote is changed from the st edition. There, instead of the first sentence above, I incorrectly wrote: “The formal content of saying that N is ‘nominalistically statable’ is OUP CORRECTED PROOF – FINAL, //, SPi m at h e m at i c a l e n t i t i e s an d t h e oret i c a l e n t i t i e s let S be any mathematical theory. Then A∗ isn’t a consequence of N∗ + S + ‘∃x¬M(x)’ unless A is a consequence of N. Why should we believe this principle? Well, it follows from a slightly stronger principle that is perhaps a bit more obvious: Principle C : Let A be any nominalistically statable assertion, and N any body of such assertions. Then A∗ isn’t a consequence of N∗ + S unless it is a consequence of N∗ alone. This in turn is equivalent (assuming the underlying logic to be compact) to something still more obvious-sounding: Principle C : Let A be any nominalistically statable assertion. Then A∗ isn’t a consequence of S unless it is logically true. Now I take it to be perfectly obvious that our mathematical theories do satisfy Principle C. After all, these theories are commonly regarded as being ‘true in all possible worlds’ and as ‘a priori true’; and though these characterizations of mathematics may be contested, it is hard to see how any knowledgeable person could regard our mathematical theories in these ways if those theories implied results about concrete entities alone that were not logically true. The same argument can be used to directly motivate Principle C , thereby obviating the need of the compactness assumption: if mathematics together with a body N∗ of nominalistic assertions implied an assertion A∗ which wasn’t a logical consequence of N∗ alone, then the truth of the mathematical theory would hinge on the logically consistent body of assertions N∗ + ¬A∗ not being true. But it would seem that it must be possible, and/or not a priori false, that such a consistent body of assertions about concrete objects alone is true; if so, simply that it not overlap in non-logical vocabulary with the mathematical theory to be introduced. (Recall that ‘=’ counts as logical.)” This obviously conflicts with the remarks above on the need of impure mathematics. For discussion, see the end of subsection . of the new Preface.] Proof: Suppose N∗ + S + ‘∃x¬M(x)’ implies A∗. Then N∗ + S implies A∗ ∨ ∀x(¬M(x) → x = x); that is, it implies B∗ where B is A ∨ ∀x(x = x). Applying Principle C , we get that N∗ implies B∗ , and consequently that N∗ + ‘∃x¬M(x)’ implies A∗. From this it clearly follows that N implies A. Principle C does not quite follow from Principle C, for a theory S could imply that there are non-mathematical objects but not imply anything else about the non-mathematical realm (in particular, not imply that there are at least two mathematical objects—the latter would violate Principle C as well as Principle C ). OUP CORRECTED PROOF – FINAL, //, SPi m at h e m at i c a l e n t i t i e s an d t h e oret i c a l e n t i t i e s then the failure of Principle C would show that mathematics couldn’t be ‘true in all possible worlds’ and/or ‘a priori true’. The fact that so many people think it does have these characteristics seems like some evidence that it does indeed satisfy Principle C and therefore Principle C. This argument isn’t conclusive: standard mathematics might turn out not to be conservative (i.e. not to satisfy Principle C), for it might conceiv- ably turn out to be inconsistent, and if it is inconsistent it certainly isn’t conservative. We would however regard a proof that standard mathemat- ics was inconsistent as extremely surprising, and as showing that standard mathematics needed revision. Equally, it would be extremely surprising if it were to be discovered that standard mathematics implied that there are at least non-mathematical objects in the universe, or that the Paris Commune was defeated; and were such a discovery to be made, all but the most unregenerate rationalist would take this as showing that standard mathematics needed revision. Good mathematics is conservative; a dis- covery that accepted mathematics isn’t conservative would be a discovery that it isn’t good. Indeed, as some of the mathematical arguments in the Appendix to this chapter show, the gap between the claim of consistency and the full claim of conservativeness is, in the case of mathematics, a very tiny one. In fact, for pure set theory, or for set theory that allows for impure sets but doesn’t allow empirical vocabulary to appear in the comprehension axioms, the conservativeness of the theory follows from its consistency alone. For full set theory this is not quite true; but a large part of the content of the conservativeness claim for full set theory (probably the only part of the content that is important in application) follows from the consistency of set theory alone (and still more of the content follows from slightly stronger assumptions, like that full set theory is ω-consistent). These claims are demonstrated in the Appendix to this chapter. In any case, I think that the two previous paragraphs show that the same sort of quasi-inductive grounds we have for believing in the consistency of mathematics extend to its conservativeness as well. As we saw earlier, this means that there is a marked disanalogy between mathematical theories and physical theories about unobservable entities: physical theories about unobservables are certainly not conservative, they give rise to genuinely new conclusions about observables. What the facts about mathematics I have been emphasizing here show is that even someone who doesn’t believe in mathematical entities is free OUP CORRECTED PROOF – FINAL, //, SPi m at h e m at i c a l e n t i t i e s an d t h e oret i c a l e n t i t i e s to use mathematical existence-assertions in a certain limited context: he can use them freely in deducing nominalistically stated consequences from nominalistically stated premises. And he can do this not because he thinks those intervening premises are true, but because he knows that they preserve truth among nominalistically stated claims. In what sense does he know this? At the very least, he knows it in the sense that a platonist mathematician who proves a result in recursive function theory by means of Church’s thesis knows that he could construct a proof that didn’t invoke Church’s thesis. The platonist mathematician hasn’t proved using the basic forms of argument that he accepts that such a proof is possible, for he hasn’t proved Church’s thesis. (Nor can he even state Church’s thesis except by vague terms like ‘intuitively computable’.) Still, there is a perfectly good sense in which our platonist mathematician does know that a proof without Church’s thesis is possible—after all, he could probably come up with Turing machine programs at each point where Church’s thesis was invoked, if given sufficient incentive to do so. In precisely the same sense, the nominalist knows that for any platonist proof of a nominalistically stated conclusion from nominalistically stated premises there is a nominalistic proof of the same thing. Just what this sense of ‘know’ is (or, just what kind of knowledge is involved) is a difficult matter: it doesn’t seem to me quite right to call it ‘inductive’ knowledge. But however this may be, it is a kind of knowledge (or justification) whose strength can be increased by inductive considerations: in the recursive function case, by knowledge that in the past one had been able to transform proofs involving the imprecise notion of ‘intuitively computable’ to proofs not involving it when one has tried (or by knowledge that others have been able to effect such transformations, and that one’s own judgements of intuitive computability tend to coincide with theirs). In the conservativeness case, the kind of inductive considerations that are relevant are the knowledge that in the past no one has found counterexamples to conservativeness, and also the knowledge that in many actual cases where platonistic devices are used in proofs of nominalistic conclusions from nominalistic premises (such as the cases discussed in Chapters and ), these devices are eliminable in what seems to be a more or less systematic way. These remarks suggest that the nominalistic position concerning the use of platonistic proofs is about comparable to the platonist’s position concerning proofs that use Church’s thesis. Actually I think that the nominalist’s position is in one respect even better, for he can rely on something that the platonistic recursion theorist has no analog of: viz., the mathematical arguments for conservativeness given in the Appendix. Of course, these arguments don’t raise the claim that mathematics is conservative to complete certainty, for two reasons. One reason is that something at least as strong as the consistency of set theory is assumed in them, and no one (platonist or nominalist) can be completely sure of that. The other reason is that these proofs (at least the first, and both if one is sufficiently strict about what counts as nominalist) are platonistic, and so some story has to be told about how the nominalist is justified in appealing to them outside the context of a reductio. I think some such story can be told, but it would be a long one. (An essential idea of the story would be that we use conservativeness to argue for conservativeness: we’ve seen that the nominalist has various initial quasi-inductive arguments which support the conclusion that it is safe to use mathematics in certain contexts; if he then using mathematics in one of those contexts can prove that it is safe to use mathematics in those contexts, this can raise the support of the initial conclusion quite substantially.) OUP CORRECTED PROOF – FINAL, //, SPi m at h e m at i c a l e n t i t i e s an d t h e oret i c a l e n t i t i e s This point is not of course intended to license the use of mathematical existence assertions in axiom systems for the particular sciences: such a use of mathematics remains, for the nominalist, illegitimate. (Or more accurately, a nominalist should treat such a use of mathematics as a temporary expedient that we indulge in when we don’t know how to axiomatize the science properly, and that we ought to try to eliminate.) The point I am making, however, does have the consequence that once such a nominalistic axiom system is available, the nominalist is free to use any mathematics he likes for deducing consequences, as long as the mathematics he uses satisfies Principle C. So if we ignore for the moment the role of mathematics in axiomatizing the sciences, then it looks as if the satisfaction of Principle C is the really essential property of mathematical theories. The fact that mathematical theories have this property is doubtless one motivation for the platonist’s assertion that such theories are ‘true in all possible worlds’. It does not appear to me, however, that the satisfaction of Principle C provides reason for regarding a theory as true at all (even in the actual world). Certainly such speculations, typical of extreme platonism, as to for instance whether the continuum hypothesis is ‘really true’, seem to lose their point once one recognizes conservativeness as the essential requirement of mathematical theories: for the usual Gödel and Cohen relative consistency proofs about set theory plus the continuum hypothesis and set theory plus its denial are easily modified into relative conservativeness proofs. In other words, assuming that standard set theory satisfies Principle C, so does standard set theory plus the continuum hypothesis and standard set theory plus its denial; so it follows that either theory could be used without harm in deducing consequences about concrete entities from nominalistic theories. The same point made about the continuum hypothesis holds as well for less recherché mathematical assertions. Even standard axioms of number theory can be modified without endangering Principle C; similarly for standard axioms of analysis. What makes the mathematical theories we A platonist might be inclined to dismiss the sort of quasi-inductive knowledge discussed in this note. But to do so would be to pay a high price: most of mathematics is known only in this quasi-inductive sort of way. For most of it is proved by rather informal proofs; and though we all do in an important sense know that we could reconstruct such proofs formally if forced to do so, still the principle that formal proofs are always possible when we have an intuitively acceptable proof is, like Church’s thesis, a principle that we haven’t proved and have no prospect of proving. OUP CORRECTED PROOF – FINAL, //, SPi m at h e m at i c a l e n t i t i e s an d t h e oret i c a l e n t i t i e s accept better than these alternatives to them is not that they are true and the modifications not true, but rather that they are more useful: they are more of an aid to us in drawing consequences from those nominalistic theories that we are interested in. If the world were different, we would be interested in different nominalistic theories, and in that case some of the alternatives to some of our favorite mathematical theories might be of more use than the theories we now accept. Thus mathematics is in a sense empirical, but only in the rather Pickwickian sense that is an empirical question as to which mathematical theory is useful. It is in an equally Pickwickian sense, however, that mathematical axioms are a priori: they are not a priori true, for they are not true at all. The view put forward here has considerable resemblance to the logical positivist view of mathematics. One difference that is probably mostly verbal is that the positivists usually described pure mathematics as ana- lytically true, whereas I have described it as not true at all; this difference is probably mostly verbal, given their construal of ‘analytic’ as ‘lacking factual content’. A much more fundamental difference is that what wor- ried the positivists about mathematics was not so much its postulation of entities as its apparently non-empirical character, and this was a problem not only for mathematics, but for logic as well. Hence they regarded logic as analytic or contentless in the same sense that mathematics was. I believe that this prevented them from giving any clear explanation of what the ‘contentlessness’ of mathematics (or of that part of mathematics that quantifies over abstract entities) consists in. The idea of calling a logical or mathematical assertion ‘contentless’ was supposed to be that a conclu- sion arrived at by a logical or mathematical argument was in some sense ‘implicitly contained in’ the premises: in this way, the conclusion of such an argument was ‘not genuinely new’. Unfortunately, no clear explanation of the idea that the conclusion was ‘implicitly contained in’ the premises was ever given, and I do not believe that any clear explanation is possible. What I have tried to do in this chapter is to show how by giving up (or saving for separate explication) the claim that logic (and that part of math that doesn’t make reference to abstract entities) doesn’t yield genuinely new conclusions, we can give a clear and precise sense to the idea that mathematics doesn’t yield genuinely new conclusions: more precisely, we We will see, however, that the utility of number theory is less subject to such empirical vicissitudes than are theories about say the real numbers. OUP CORRECTED PROOF – FINAL, //, SPi a ppe n di x : on c on se rvat i v e n e s s can show that the part of math that does make reference to mathematical entities can be applied without yielding any genuinely new conclusions about non-mathematical entities. Appendix: On Conservativeness It may be illuminating to give two mathematical arguments for the con- servativeness of mathematics. The first argument proves, from a set- theoretic perspective (more specifically, from the perspective of ordinary set theory plus the axiom of inaccessible cardinals) that ordinary set theory (and hence standard mathematics, which is reducible to ordinary set theory) is definitely conservative. The second argument is a purely proof-theoretic one: it establishes a slightly restricted form of the conser- vativeness claim on the basis merely of the assumption that standard set theory is consistent. This is illuminating in showing that the assumption of the conservativeness of set theory is much ‘closer to’ the assumption that set theory is consistent than to the assumption that it is true. As a preliminary, let’s introduce some notation. Let ZF be standard Zermelo-Fraenkel set theory (including the axiom of choice); let restricted ZFU be ZF modified to allow for the existence of urelements, but not allowing for any non-set-theoretic vocabulary to appear in the compre- hension axioms (for definiteness, we may stipulate that it contains as an axiom that there is a set of all non-sets). [Added in this edition: In accor- dance with the discussion in the text, we take ZFU to include the assertion that ∀x(Set(x) → M(x)), leaving open whether some urelements (e.g. numbers conceived as non-sets) are also mathematical.] If V is a class of expressions, let ZFUV be restricted ZFU together with any instances of the comprehension schemas in which the vocabulary in V as well as the set-theoretic vocabulary is allowed to appear. What I earlier called ‘full set theory’ isn’t really a single theory: rather, to ‘apply full set theory’ in the context of a theory T is to apply ZFUV(T) , where V(T) is the vocabulary of T. Consequently, what we want to prove is that for any theory T, ZFUV(T) applies conservatively to T. That is, we want to prove (C ) If T is any consistent body of assertions not containing ‘Math’ or ‘∈’ or ‘Set’, then ZFUV(T) + T∗ is also consistent. [Changed from st edition: I’ve added “not containing ‘Math’ or ‘∈’ or ‘Set’ ”] OUP CORRECTED PROOF – FINAL, //, SPi m at h e m at i c a l e n t i t i e s an d t h e oret i c a l e n t i t i e s (The T here is the N + ¬A of Principle C.) This in fact will suffice for proving the conservativeness of ZFUV(T) + S, for any standard math- ematical theory S: for standard mathematical theories are embeddable in ZF. So much for preliminaries. How then do we prove that (C ) holds? The obvious set-theoretic line of proof is this: Suppose T is consistent; then it has a model M of accessible cardinality, say with domain D. Pick any entity e not in D. (e is to be thought of as the empty set.) Let D be D ∪ {e}; Let D consist of all non-empty subsets of D ; let D consist of all non-empty subsets of D ∪ D ; and so on. Let Dω be D ∪ D ∪ D ∪... ; let Dω+ consist of all non-empty subsets of Dω ; and so on. Continuing in this way until you reach an inaccessible cardinal, you get—if certain initial precautions are taken on the choice of D and e—a model of ZFUV(T) + T∗. (It is a model of ZFUV(T) + T∗ rather than merely of ZFU + T∗ because at each stage you’ve added every set of things available at previous stages.) So ZFUV(T) + T∗ is consistent. Q.E.D. Now let us turn to the proof-theoretic line of argument for conserva- tiveness; the point of doing this is to make clear how narrow the gap is between the consistency of mathematics and its conservativeness. Indeed, in the case of mathematical theories which don’t allow for impure abstract entities (e.g. number theory by itself, or ZF), consistency implies conservativeness: this is an obvious consequence of the Robinson joint consistency theorem. The same result holds also in the more D should either be taken to consist entirely of non-sets, in which case e should be taken to be the empty set (or another non-set); or D should be taken to consist entirely of sets of the same rank and e should be another set of that rank. Given any model of a theory, there is no difficulty in getting another model whose domain meets these conditions. Suppose S + T∗ is inconsistent; the Robinson theorem says that there is a sentence B in the language common to S and T∗ such that S B and T∗ ¬B. Clearly if S and T are both consistent, then B can’t be either a logical truth or a contradiction. The language common to S and T∗ consists, in the case of a ‘pure’ mathematical theory, of ‘M’ (the predicate ‘mathematical’ discussed prior to the formulation of Principle C) and ‘=’, and nothing else. The only statements in this language other than logical truths or contradictions are statements saying how many mathematical objects there are and/or how many non- mathematical objects there are. But since all statements in T∗ are explicitly restricted to non-mathematical objects, T∗ can’t imply anything about how many mathematical objects there are, and since the mathematical theory is assumed to be a pure one it can’t imply anything about how many non-mathematical objects there are. So there can be no such B; that is, the supposition that S and T are consistent but S + T∗ is inconsistent has been reduced to absurdity. OUP CORRECTED PROOF – FINAL, //, SPi a ppe n di x : on c on se rvat i v e n e s s interesting case of restricted ZFU: here one needs, in addition to the Robinson theorem, the well-known fact that if ZFU is consistent then one can’t prove any result about how many non-sets there are. But in the really interesting case of full ZFU, this whole line of argument via the joint consistency theorem is blocked by the fact that the empirical vocabulary that appears in the theory T also appears in set-theoretic comprehension axioms. The simplest thing to do in this case is to mimic proof-theoretically the set-theoretic argument given three paragraphs back: doing so, it becomes an argument that under certain conditions ZFUV(T) + T∗ is interpretable within ZFUV(T) , and in fact within ZF. (We don’t need the inaccessible cardinal assumption anymore.) If the ‘certain conditions’ were merely that T is consistent, then we’d know that (C ) holds as long as ZF is consistent, and this is what we wanted. Unfortunately however we need the stronger assumption that T is provably consistent within ZF; that is, the best we can show is that if ZF is consistent, the following holds: (C ) If T is any body of assertions whose consistency is provable in ZF, then ZFUV(T) + T∗ is consistent. A sketch of the proof of the last fact is given in Jech : , problem . Using this fact, the proof that conservativeness implies consistency is just as in note . Proof: if ZF is consistent, and ZF ‘T is consistent’ (where ‘T is consistent’ abbreviates the formalization in ZF of the claim that T is syntactically consistent) then ZF + ‘T is consistent’ is certainly consistent. Since the Gödel completeness theorem (together with various more elementary facts) is provable in ZF, then so is ZF + ‘there is a model of T in which all elements of the domain have the same rank and such that there is a set of that rank that is not in the domain’. (Cf. note for the motivation of this.) If T has n primitive predicates, then a model of T consists of a domain together with n items each corresponding to one of the terms. Introducing new names b, c ,... , cn for these things, and a name d for the set of the right rank that isn’t in the domain of the model, we see that ZF + ‘ b, c ,... , cn is a model of T’ + ‘all members of b have the same rank’ + ‘d has the same rank as all members of b’ is also consistent. Call this theory ZFT. By the principle of transfinite recursion, there is a formula D(x) (in the language of ZF T ) such that ZFT (in fact, ZF) D(x) ↔ x ∈ b ∨ x = d ∨ (x = ∅ ∧ ∀y(y ∈ x →D(y))). If we translate statements of ZFUV(T) + T∗ into ZFT by using D(x) to restrict all variables, and translate ‘Set(x)’ as ‘x ∈ / b’, ‘∈’ as ‘∈’, ‘∅’ as ‘d’, and ‘Ai (x ,... , xk )’ where Ai is the ith predicate of T as ‘ x ,... , xk ∈ ci ’, then each of the translations of the axioms of ZFUV(T) + T∗ is a theorem of ZFT. Since ZFT is consistent (on the assumption that ZF is), so is ZFUV(T) + T∗. OUP CORRECTED PROOF – FINAL, //, SPi m at h e m at i c a l e n t i t i e s an d t h e oret i c a l e n t i t i e s This is a restricted version of conservativeness: it says that full set the- ory applies conservatively to theories which are modellable in ZF. In actual applications this is probably as much of the conservativeness claim as we ever need. For instance, later on in the book we will want to know that mathematics applies conservatively to a nominalistic ver- sion of Newtonian gravitation theory, N. But it is completely obvi- ous that if N is consistent then it is modellable in ZF (and the same would presumably be true for other nominalized physical theories); so the conservativeness result we actually need follows merely from the consistency of ZF. Scott Weinstein (besides clearing up a number of confusions I had gotten into concerning the issues of the last paragraph) pointed out to me that if you strengthen the consistency assumption about ZF slightly, to ω-consistency (or even something a bit weaker than that known as -consistency), you can strengthen (C ) in an attractive way: you can then prove (C ) If T is any consistent and recursively enumerable body of asser- tions, then ZFUV(T) + T∗ is consistent. It is all the more obvious that this would be sufficient for practical applications. Philosophers discussing set theory tend to discuss two of its properties: its consistency, and its (alleged) truth. The argument of this monograph is that the latter is completely irrelevant, and that the former is perhaps a bit too weak—it is too weak unless one is satisfied with (C ) instead of the full (C ). [Of course, for the kind of set theory philosophers tend to discuss— pure set theory, i.e. ZF—there is no difference at all between consistency and conservativeness (or rather, though they differ conceptually, they are provably equivalent). But pure set theory isn’t what is of interest, since To see this, observe first that the preceding note proved a slightly stronger result than was claimed: it proved that if ZF + ‘T is consistent’ is consistent, then ZFUV(T) + T∗ is consistent. So we now need only show that if ZF is ω-consistent and T is consistent and recursively enumerable, then ZF + ‘T is consistent’ is consistent. The reason for this is simple: if T is consistent, then nothing is a proof from T of ‘ = ’; and if T is also recursively enumerable, ZF is strong enough to prove ‘k is not the Gödel number of a proof from T of ‘ = ’ ’, for each numeral k. By the ω-consistency of ZF it follows that one cannot prove in ZF anything of the form ‘∃x(x is the Gödel number of a proof from T of ‘ = ’)’; so one can’t prove ‘T is not consistent’ from ZF, and so ZF + ‘T is consistent’ is consistent. OUP CORRECTED PROOF – FINAL, //, SPi a ppe n di x : on c on se rvat i v e n e s s as remarked before it can never be applied to the physical world, so this is not much of a justification for ignoring conservativeness.] But though we perhaps need to assume a bit more than consistency, we don’t need to assume all that much more; and in any case it seems pretty obvious that the stronger property of conservativeness does in fact obtain.