Genetics – An Introduction PDF
Document Details
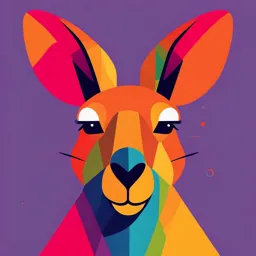
Uploaded by NonViolentGreekArt7258
TCD
Matthew Campbell
Tags
Related
Summary
This document provides an introduction to genetics, covering its history, key concepts, and applications. It details Mendelian genetics, DNA structure, and genomics. The document also touches upon genetic disorders and diseases. It's intended for an undergraduate biology course.
Full Transcript
Genetics – An Introduction Professor Matthew Campbell School of Genetics & Microbiology, TCD. Biology - A Global Approach (11th edition, Pearson) Campbell, Urry, Cain, Wasserman, Minorsky & Reece Biological Information: Genetics, Heredity and DNA Key concepts that will be covered: * Introduction to...
Genetics – An Introduction Professor Matthew Campbell School of Genetics & Microbiology, TCD. Biology - A Global Approach (11th edition, Pearson) Campbell, Urry, Cain, Wasserman, Minorsky & Reece Biological Information: Genetics, Heredity and DNA Key concepts that will be covered: * Introduction to genetics: history, overview, applications * Mendelian genetics * Linkage and recombination * Identification of DNA as the hereditary material * Quantitative genetics * DNA, structure and function * DNA – mutation, recombination and repair * The central dogma MCQ evaluation A Whistle-Stop Tour of 150 years of Genetics – History 1860s Mendel published his research on inheritance of unit factors. Cytologists describe chromosomes & their behaviour during mitosis & meiosis. 1900s Rediscovery of Mendel’s work. Chromosomes behave like unit factors. Theterm“Gene” proposedtoreplaceunitfactors.Genetics becomesa discipline in itself. 1905 William Bateson first uses the term ‘genetics’ to describe the study of inheritance 1940s Confirmation that the genetic material is DNA not protein. 1950s Watson and Crick describe double-helical structure of DNA - Molecular Biology Era Begins 1960s Cracking the triplet ‘code’ and defining the pathway of information flow: ‘DNA makes RNA makes protein’ James Watson Francis Crick Rosalind Franklin DNA structure 1953 1970s Discovery of restriction enzymes: Recombinant DNA technology becomes possible. Expression of human growth hormone gene in E. coli; Discovery of split genes in eukaryotes - introns & exons; methods for sequencing DNA. 1980s Commercialization of Recombinant DNA technology. Methods for making transgenic plants & animals. 1990s Genome sequencing: Human, plant, drosophila, nematode, microbial genomes & many other genomes sequenced. 2000s First complete human genome sequence 2003. Cost approx. $3 billion! 2000s Technology for expression profiling of the entire gene complement in a genome. 2000s RNA interference; genome editing; induced pluripotent stem (iPS) cells & many others - some of which we will cover during the lecture course 2018. 2018 Whole human genome sequence (WGS) costs less than $1,000! 2019. 2019 Era of genome editing and gene therapy takes off ! Life’s processes involve the Expression & Transmission of Genetic Information Within cells, structures called chromosomes contain genetic material in the form of DNA (deoxyribonucleic acid) Each chromosome contains one long DNA molecule with hundreds or thousands of genes Genes are the units of inheritance - they encode information for building the molecules synthesized within the cell The genetic information encoded by DNA directs the development of an organism and the maintenance of cells in the organism Nuclei containing DNA Sperm cell Egg cell Fertilized egg with DNA from both parents Embryo’s cells with copies of inherited DNA © 2018 Pearson Education Ltd. Offspring with traits inherited from both parents The molecular structure of DNA accounts for its ability to store information Each DNA molecule is made up of two long chains arranged in a double helix Each chain is made up of four kinds of chemical building blocks called nucleotides and abbreviated A, Nucleus DNA Nucleotide ACTATACC GTAGTA G, C, and T © 2018 Pearson Education Ltd. Cell Adenine Guanine Thymine Cytosine (a) DNA double helix (b) Single strand of DNA Chromatin 3 x 109 bp of DNA in the human genome – 3 billion bases. If there were 3,000 letters on a typed page that would be 1 million pages of text in our genomes – the same 1 million pages of text in every nucleus of every cell in the human body. Approx. 1 in 1,000 bases varies between individuals. Humans have 10-100 trillions of cells, each cell with approx. 2 meters of DNA packaged into the nucleus of the cell. There are over 3,000 genetic diseases in humans which are termed ‘Mendelian’ – due to a mutation in a single gene 3 billlion nucleotides of DNA code in each cell.... so tightly packaged.... 2 meters of DNA inside the nucleus of each cell Male Female Human Karyotype Traditional methods of identifying chromosomes involve staining with dyes that produced banding patterns. These chromosomes are stained with Giemsa (G- banded). Another form of staining is Q-banding. What is this karyotype representative of...Male or Female? The same 3 billion base pairs of DNA are present in every cell of your body. Approx. 20,000 genes in 23 pairs of human chromosomes in 3 billion DNA base pairs. How does each cell function so differently? Not all genes are active in all cell types. Genes make RNA which is translated into proteins, the building blocks required for each cell to function. Different cell types need different proteins to function. Mutations in the DNA sequence can result in no protein or incorrect proteins being formed giving rise to genetic disorders. Mutations in a single gene that give rise to a disease are called single gene defects (or Mendelian disorders after Gregor Mendel) e.g. Cystic fibrosis etc DNA Genes –> RNA Messages –> Proteins The Central Dogma The Central Dogma of Molecular Biology For many genes, the sequence provides the blueprint for making a protein. Protein-encoding genes control protein production indirectly. DNA is transcribed into RNA, which is then translated into a protein. Gene expression is the process of converting information from gene to cellular product. It turns out that only about 1% of our genome codes for proteins. Much of our genome makes RNA but does not code for protein – termed non-coding RNAs.. © 2018 Pearson Education Ltd. Genomics: Large-Scale Analysis of DNA Sequences An organism’s genome is its entire “library” of genetic instructions Genomics is the study of sets of genes in one or more species Proteomics is the study of whole sets of proteins and their properties The entire set of proteins expressed by a given cell, tissue, or organ is called a proteome The genomics approach depends on: “High-throughput” technology, which yields enormous amounts of data Bioinformatics, which is the use of computational tools to process a large volume of data Interdisciplinary research teams Human Genome Sequencing Project: 3 billion nucleotides of DNA code in the human genome - 1st human complete genome sequence published in 2003 Human Genome Sequencing 1st human genome sequence – estimated cost $2-3 billion (2003) Whole human genome sequence (WGS) costs less than $1,000 – 2019! Over a million fold price drop! We are in an era of big data (Moore's law is the observation that the number of transistors in a dense integrated circuit doubles approx. every two years). Genes and Diseases...... Inheritance patterns - Mendelian diseases (single gene defects), quantitative traits Identifying disease genes and associated mutations Moving towards rational therapies based on genetic cause Achondroplasia Affects 1 in 15,000 to 1 in 40,000 newborns. Most cases are sporadic – parents are not affected and these cases are caused by de novo (spontaneous, new) mutations in the germ line. However, an affected individual will transmit the condition as an autosomal dominant disease – 50% of offspring on average will pick up the gene. Features: Short stature (av. 4 feet 1 inch – 124 cm); average sized trunk; but arms and legs short; disproportionately large head with prominent forehead; spinal curvature which can cause back pain in older subjects; short fingers; normal intelligence. Gene: a dominant mutation within the FGFR3 gene (encodes fibroblast growth factor receptor 3). The growth factor receptor becomes hyperactive – the mutant protein dominates the normal allele, negatively affecting bone development. http://www.google.ie/images?hl=en&q=achondroplasia Autosomal dominant On average 50% of children are affected with the disease and 50% are unaffected Mendelian Disorders These are single gene defects - diseases due to a mutation in a single gene gig.org.uk/education2.htm Retinitis pigmentosa (recessive form) Consanguineous pedigree Autosomal recessive Mendelian Disorders Single gene defects - diseases due to a mutation in a single gene gig.org.uk/education2.htm On average 25% of children are normal, 50% are carriers and 25% are affected with the disease An X linked recessive Gene -Haemophilia X-linked / Sex linked XY XX XY XX Mendelian disorders – single gene defects - diseases due to a mutation in a sinlge gene All female children from an affected male will be carriers of the disease On average 50% of male children from a carrier female will have the disease & 50% of female children will be carriers X-linked health.allrefer.com/pictures-images/x-linked-recessive-genetic- defects.html Over 3,000 single gene disorders / Mendelian disorders in humans Disorder Frequency per1000 births Examples of autosomal dominant diseases: Familial combined hyperlipidaemia 5.0 Familial hypercholsterolaemia 2.0 Dominant otosclerosis 1.0 Adult polycystic kidney disease 0.8 Multiple exostoses 0.5 Huntington's disease 0.5 Neurofibromatosis0.4 Myotonic Dystrophy 0.2 Congenital spherocytosis 0.2 Polyposis coli 0.1 Over 3,000 single gene disorders / Mendelian disorders in humans Disorder Frequency per 1000 births Examples of autosomal recessive diseases: Cystic fibrosis 0.4 alpha-1-antitrypsin deficiency 0.2 Phenylketonuria 0.1 Congenital adrenal hyperplasia 0.1 Spinal muscular atrophy 0.1 Sickle cell anaemia 0.1 beta-Thalassaemia 0.05 Over 3,000 single gene disorders / Mendelian disorders in humans Disorder Frequency per 1000 births Examples of x-linked recessive diseases: Fragile X syndrome 0.5 Duchenne muscular dystrophy 0.3 X- linked ichthyosis 0.2 Haemophilia A 0.1 Becker muscular dystrophy 0.05 Haemophilia B 0.03 Gene-based medicines Knowledge of the genes causing genetic conditions enables the development of methods of intervention Dominant diseases: The strategy may require suppression of expression of the mutant gene (& thereby the mutant protein) Recessive diseases: The strategy involves supply of the wild type / normal copy of the gene to supply the wild type protein Gene Replacement - see video LCA (vision loss - RPE65 gene) Drug development Using knowledge from the human genome project and tools such a viral vectors and cell and animal models, the development of therapies for many human genetic disorders becomes a realistic aspiration. Knowledge of the underlying genetic basis of a condition enables the development of designer therapies targeted towards the cause of the disease, as opposed to the somewhat random approach (serendipity) at times used to develop drugs in the past. Inherited Retinal Disorders Leber Congenital Amaurosis (LCA) – an autosomal recessive eye disorder. The RPE65 gene is one of the causes of LCA and also some other types of recessive eye disease. The RPE65 gene encodes an important enzyme involved in regenerating light sensitive molecules in the retina. Gene Medicines In Action for a Recessive Eye Disorder – RPE65 Let’s look at a very short video of a gene medicine at work in the eye..... one of the first ever patients treated with this gene medicine in the world by Professors Jean Bennett, Albert McGuire & Kathy High in the University of Pennslyvania and now being developed by Spark Therapeutics Inc (Philadelphia). FDA approved - Dec 2017. LCA/RPE65 – Gene Therapy Video Leber’s Congenital Amaurosis (LCA) is a recessive genetic eye disorder where often children early in life have significant visual loss. It is recessive & so in principle delivery of the normal (wild type) gene should provide benefit. There are some human clinical trials for LCA. Here is a video from a University of Pennsylvania trial (Prof Jean Bennett, Albert Maguire, Kathy High & colleagues), now being developed by Spark Therapeutics (Philadelphia). Patients in the U Penn/Spark trials have been evaluated in Phase I/II & III trials. The therapy, Luxturna, provided benefit & as of December 2017 has been approved by the US regulatory body, the FDA. In this video the patient is injected in one eye with a virus (AAV) that carries the normal gene (RPE65 gene). The other eye is untreated. Knowledge of the cause of a genetic disease together with a method to get the normal gene into the target cell type represents a powerful therapeutic approach for many genetic disorders. To gain an understanding of a gene therapy like Luxturna, some background information about genetics is required, which will be presented over the course of the next few lectures. Genetics has many applications – here are just a few examples relating to human health 1. Production of safer vaccines: recombinant single subunit vaccines e.g. hepatitis B vaccine 2. Production of recombinant human “therapeutic” proteins e.g. insulin, growth hormone, clot dissolving proteins 3. Inherited disorders can be diagnosed prenatally 4. Prenatal genotyping – in vitro fertilisation & pre implantation diagnosis 5. Pharmacogenomics: using genomics to genotype populations & individuals for alleles that determine responsiveness to drug therapies.....and many others Let’s quickly look at 2 examples... Individualisation of medicines - Pharmacogenomics Research is on-going into understanding differences in drug response that exist between patients – Pharmacogenomics. What genes influence drug response? In liver & intestine a large number of genes are expressed into drug metabolising enzymes (DMEs). Allelic variants of the genes encoding DMEs exist in human populations resulting in heritable differences in DMEs between people. These genetic variants are in part responsible for the differences between people in response to drugs including chemotherapeutics, immuno-suppressants, anti- depressants, pain killers etc. For example, TPMT is a DME that is involved in the metabolism of chemotherapeutic drugs. 1 in 300 people are deficient in this enzyme (homozygous mutant) due to mutations in the gene encoding the enzyme. 1 in 10 people have lower levels of the enzyme (heterozygotes). Homozygotes can experience extreme toxicity to chemotherapeutic drugs which can at times be fatal. Adverse drug responses account for approx. 100,000 deaths per year in the US alone. Pharmcogenomics should enable the identification of such people prior to adminstering a drug thereby prevention such adverse events. CYP2D6 and Codeine The prodrug codeine is metabolised to the active drug morphine by an enzyme termed CYP2D6 (involving O- demethylation of codeine into morphine). The metabolism of codeine to morphine is undertaken almost exclusively by CYP2D6. Codeine has a 200x lower affinity for mu opioid receptors than morphine. Plasma morphine concentrations in patients after codeine administration is influenced by genotype at CYP2D6 (with almost no morphine present in some poor metabolisers). CYP2D6 poor metabolisers encode either dysfunctional or partially inactive CYP2D6 enzyme due to variants in the CYP2D6 gene - approximately 10% of Caucasians. In such individuals codeine may be an ineffective analgesic as the active drug is not formed or is formed at low levels. For patients reporting lack of pain relief from analgesics based on codeine, in many cases it may be that CYP2D6 genotype is responsible. Codeine is an active ingredient in a lot of non- prescription analgesic medications, e.g. Solpadeine etc Biological Information: Genetics, Heredity and DNA Lectures 26-37 Key concepts that will be covered: * Introduction to genetics: history, overview, applications * Mendelian genetics * Linkage and recombination * Identification of DNA as the hereditary material * Quantitative genetics * DNA, structure and function * DNA – mutation, recombination and repair * The central dogma MCQ evaluation Matthew Campbell [email protected] Genetics – An Introduction Professor Matthew Campbell School of Genetics & Microbiology, TCD. Biology - A Global Approach (11th edition, Pearson) Campbell, Urry, Cain, Wasserman, Minorsky & Reece Biological Information: Genetics, Heredity and DNA Key concepts that will be covered: * Introduction to genetics: history, overview, applications * Mendelian genetics * Linkage and recombination * Identification of DNA as the hereditary material * Quantitative genetics * DNA, structure and function * DNA – mutation, recombination and repair * The central dogma MCQ evaluation A Whistle-Stop Tour of 150 years of Genetics – History 1860s Mendel published his research on inheritance of unit factors. Cytologists describe chromosomes & their behaviour during mitosis & meiosis. 1900s Rediscovery of Mendel’s work. Chromosomes behave like unit factors. Theterm“Gene”proposedtoreplaceunitfactors.Genetics becomesa discipline in itself. 1905 William Bateson first uses the term ‘genetics’ to describe the study of inheritance 1940s Confirmation that the genetic material is DNA not protein. 1950s Watson and Crick describe double-helical structure of DNA - Molecular Biology Era Begins 1960s Cracking the triplet ‘code’ and defining the pathway of information flow: ‘DNA makes RNA makes protein’ James Watson Francis Crick Rosalind Franklin DNA structure 1953 1970s Discovery of restriction enzymes: Recombinant DNA technology becomes possible. Expression of human growth hormone gene in E. coli; Discovery of split genes in eukaryotes - introns & exons; methods for sequencing DNA. 1980s Commercialization of Recombinant DNA technology. Methods for making transgenic plants & animals. 1990s Genome sequencing: Human, plant, drosophila, nematode, microbial genomes & many other genomes sequenced. 2000s First complete human genome sequence 2003. Cost approx. $3 billion! 2000s Technology for expression profiling of the entire gene complement in a genome. 2000s RNA interference; genome editing; induced pluripotent stem (iPS) cells & many others - some of which we will cover during the lecture course 2018 Whole human genome sequence (WGS) costs less than $1,000! 2019 Era of genome editing and gene therapy takes off ! Life’s processes involve the Expression & Transmission of Genetic Information Within cells, structures called chromosomes contain genetic material in the form of DNA (deoxyribonucleic acid) Each chromosome contains one long DNA molecule with hundreds or thousands of genes Genes are the units of inheritance - they encode information for building the molecules synthesized within the cell The genetic information encoded by DNA directs the development of an organism and the maintenance of cells in the organism Nuclei containing DNA Sperm cell Egg cell Fertilized egg with DNA from both parents Embryo’s cells with copies of inherited DNA © 2018 Pearson Education Ltd. Offspring with traits inherited from both parents The molecular structure of DNA accounts for its ability to store information Each DNA molecule is made up of two long chains arranged in a double helix Each chain is made up of four kinds of chemical building blocks called nucleotides and abbreviated A, Nucleus DNA Nucleotide ACTATACC GTAGTA G, C, and T © 2018 Pearson Education Ltd. Cell Adenine Guanine Thymine Cytosine (a) DNA double helix (b) Single strand of DNA Chromatin 3 x 109 bp of DNA in the human genome – 3 billion bases. If there were 3,000 letters on a typed page that would be 1 million pages of text in our genomes – the same 1 million pages of text in every nucleus of every cell in the human body. Approx. 1 in 1,000 bases varies between individuals. Humans have 10-100 trillions of cells, each cell with approx. 2 meters of DNA packaged into the nucleus of the cell. There are over 3,000 genetic diseases in humans which are termed ‘Mendelian’ – due to a mutation in a single gene 3 billlion nucleotides of DNA code in each cell.... so tightly packaged.... 2 meters of DNA inside the nucleus of each cell Male Female Human Karyotype Traditional methods of identifying chromosomes involve staining with dyes that produced banding patterns. These chromosomes are stained with Giemsa (G-banded). Another form of staining is Q-banding. What is this karyotype representative of...Male or Female? The same 3 billion base pairs of DNA are present in every cell of your body. Approx. 20,000 genes in 23 pairs of human chromosomes in 3 billion DNA base pairs. How does each cell function so differently? Not all genes are active in all cell types. Genes make RNA which is translated into proteins, the building blocks required for each cell to function. Different cell types need different proteins to function. Mutations in the DNA sequence can result in no protein or incorrect proteins being formed giving rise to genetic disorders. Mutations in a single gene that give rise to a disease are called single gene defects (or Mendelian disorders after Gregor Mendel) e.g. Cystic fibrosis etc DNA Genes –> RNA Messages –> Proteins The Central Dogma The Central Dogma of Molecular Biology For many genes, the sequence provides the blueprint for making a protein. Protein-encoding genes control protein production indirectly. DNA is transcribed into RNA, which is then translated into a protein. Gene expression is the process of converting information from gene to cellular product. It turns out that only about 1% of our genome codes for proteins. Much of our genome makes RNA but does not code for protein – termed non-coding RNAs.. © 2018 Pearson Education Ltd. Genomics: Large-Scale Analysis of DNA Sequences An organism’s genome is its entire “library” of genetic instructions Genomics is the study of sets of genes in one or more species Proteomics is the study of whole sets of proteins and their properties The entire set of proteins expressed by a given cell, tissue, or organ is called a proteome The genomics approach depends on: “High-throughput” technology, which yields enormous amounts of data Bioinformatics, which is the use of computational tools to process a large volume of data Interdisciplinary research teams Human Genome Sequencing Project: 3 billion nucleotides of DNA code in the human genome - 1st human complete genome sequence published in 2003 Human Genome Sequencing 1st human genome sequence – estimated cost $2-3 billion (2003) Whole human genome sequence (WGS) costs less than $1,000 – 2019! Over a million fold price drop! We are in an era of big data (Moore's law is the observation that the number of transistors in a dense integrated circuit doubles approx. every two years). Genes and Diseases...... Inheritance patterns - Mendelian diseases (single gene defects), quantitative traits Identifying disease genes and associated mutations Moving towards rational therapies based on genetic cause Achondroplasia Affects 1 in 15,000 to 1 in 40,000 newborns. Most cases are sporadic – parents are not affected and these cases are caused by de novo (spontaneous, new) mutations in the germ line. However, an affected individual will transmit the condition as an autosomal dominant disease – 50% of offspring on average will pick up the gene. Features: Short stature (av. 4 feet 1 inch – 124 cm); average sized trunk; but arms and legs short; disproportionately large head with prominent forehead; spinal curvature which can cause back pain in older subjects; short fingers; normal intelligence. Gene: a dominant mutation within the FGFR3 gene (encodes fibroblast growth factor receptor 3). The growth factor receptor becomes hyperactive – the mutant protein dominates the normal allele, negatively affecting bone development. http://www.google.ie/images?hl=en&q=achondroplasia Autosomal dominant On average 50% of children are affected with the disease and 50% are unaffected Mendelian Disorders These are single gene defects - diseases due to a mutation in a single gene gig.org.uk/education2.htm Retinitis pigmentosa (recessive form) Consanguineous pedigree Autosomal recessive Mendelian Disorders Single gene defects - diseases due to a mutation in a single gene gig.org.uk/education2.htm On average 25% of children are normal, 50% are carriers and 25% are affected with the disease An X linked recessive Gene -Haemophilia X-linked / Sex linked XY XX XY XX Mendelian disorders – single gene defects - diseases due to a mutation in a sinlge gene All female children from an affected male will be carriers of the disease On average 50% of male children from a carrier female will have the disease & 50% of female children will be carriers X-linked health.allrefer.com/pictures-images/x-linked-recessive-genetic-defects.html Over 3,000 single gene disorders / Mendelian disorders in humans Disorder Frequency per1000 births Examples of autosomal dominant diseases: Familial combined hyperlipidaemia 5.0 Familial hypercholsterolaemia 2.0 Dominant otosclerosis 1.0 Adult polycystic kidney disease 0.8 Multiple exostoses 0.5 Huntington's disease 0.5 Neurofibromatosis0.4 Myotonic Dystrophy 0.2 Congenital spherocytosis 0.2 Polyposis coli 0.1 Over 3,000 single gene disorders / Mendelian disorders in humans Disorder Frequency per 1000 births Examples of autosomal recessive diseases: Cystic fibrosis 0.4 alpha-1-antitrypsin deficiency 0.2 Phenylketonuria 0.1 Congenital adrenal hyperplasia 0.1 Spinal muscular atrophy 0.1 Sickle cell anaemia 0.1 beta-Thalassaemia 0.05 Over 3,000 single gene disorders / Mendelian disorders in humans Disorder Frequency per 1000 births Examples of x-linked recessive diseases: Fragile X syndrome 0.5 Duchenne muscular dystrophy 0.3 X-linked ichthyosis 0.2 Haemophilia A 0.1 Becker muscular dystrophy 0.05 Haemophilia B 0.03 Gene-based medicines Knowledge of the genes causing genetic conditions enables the development of methods of intervention Dominant diseases: The strategy may require suppression of expression of the mutant gene (& thereby the mutant protein) Recessive diseases: The strategy involves supply of the wild type / normal copy of the gene to supply the wild type protein Gene Replacement - see video LCA (vision loss - RPE65 gene) Drug development Using knowledge from the human genome project and tools such a viral vectors and cell and animal models, the development of therapies for many human genetic disorders becomes a realistic aspiration. Knowledge of the underlying genetic basis of a condition enables the development of designer therapies targeted towards the cause of the disease, as opposed to the somewhat random approach (serendipity) at times used to develop drugs in the past. Inherited Retinal Disorders Leber Congenital Amaurosis (LCA) – an autosomal recessive eye disorder. The RPE65 gene is one of the causes of LCA and also some other types of recessive eye disease. The RPE65 gene encodes an important enzyme involved in regenerating light sensitive molecules in the retina. Gene Medicines In Action for a Recessive Eye Disorder – RPE65 Let’s look at a very short video of a gene medicine at work in the eye..... one of the first ever patients treated with this gene medicine in the world by Professors Jean Bennett, Albert McGuire & Kathy High in the University of Pennslyvania and now being developed by Spark Therapeutics Inc (Philadelphia). FDA approved - Dec 2017. LCA/RPE65 – Gene Therapy Video Leber’s Congenital Amaurosis (LCA) is a recessive genetic eye disorder where often children early in life have significant visual loss. It is recessive & so in principle delivery of the normal (wild type) gene should provide benefit. There are some human clinical trials for LCA. Here is a video from a University of Pennsylvania trial (Prof Jean Bennett, Albert Maguire, Kathy High & colleagues), now being developed by Spark Therapeutics (Philadelphia). Patients in the U Penn/Spark trials have been evaluated in Phase I/II & III trials. The therapy, Luxturna, provided benefit & as of December 2017 has been approved by the US regulatory body, the FDA. In this video the patient is injected in one eye with a virus (AAV) that carries the normal gene (RPE65 gene). The other eye is untreated. Knowledge of the cause of a genetic disease together with a method to get the normal gene into the target cell type represents a powerful therapeutic approach for many genetic disorders. To gain an understanding of a gene therapy like Luxturna, some background information about genetics is required, which will be presented over the course of the next few lectures. Genetics has many applications – here are just a few examples relating to human health 1. Production of safer vaccines: recombinant single subunit vaccines e.g. hepatitis B vaccine 2. Production of recombinant human “therapeutic” proteins e.g. insulin, growth hormone, clot dissolving proteins 3. Inherited disorders can be diagnosed prenatally 4. Prenatal genotyping – in vitro fertilisation & pre implantation diagnosis 5. Pharmacogenomics: using genomics to genotype populations & individuals for alleles that determine responsiveness to drug therapies.....and many others Let’s quickly look at 2 examples... Individualisation of medicines - Pharmacogenomics Research is on-going into understanding differences in drug response that exist between patients – Pharmacogenomics. What genes influence drug response? In liver & intestine a large number of genes are expressed into drug metabolising enzymes (DMEs). Allelic variants of the genes encoding DMEs exist in human populations resulting in heritable differences in DMEs between people. These genetic variants are in part responsible for the differences between people in response to drugs including chemotherapeutics, immuno-suppressants, anti-depressants, pain killers etc. For example, TPMT is a DME that is involved in the metabolism of chemotherapeutic drugs. 1 in 300 people are deficient in this enzyme (homozygous mutant) due to mutations in the gene encoding the enzyme. 1 in 10 people have lower levels of the enzyme (heterozygotes). Homozygotes can experience extreme toxicity to chemotherapeutic drugs which can at times be fatal. Adverse drug responses account for approx. 100,000 deaths per year in the US alone. Pharmcogenomics should enable the identification of such people prior to adminstering a drug thereby prevention such adverse events. CYP2D6 and Codeine The prodrug codeine is metabolised to the active drug morphine by an enzyme termed CYP2D6 (involving O-demethylation of codeine into morphine). The metabolism of codeine to morphine is undertaken almost exclusively by CYP2D6. Codeine has a 200x lower affinity for mu opioid receptors than morphine. Plasma morphine concentrations in patients after codeine administration is influenced by genotype at CYP2D6 (with almost no morphine present in some poor metabolisers). CYP2D6 poor metabolisers encode either dysfunctional or partially inactive CYP2D6 enzyme due to variants in the CYP2D6 gene - approximately 10% of Caucasians. In such individuals codeine may be an ineffective analgesic as the active drug is not formed or is formed at low levels. For patients reporting lack of pain relief from analgesics based on codeine, in many cases it may be that CYP2D6 genotype is responsible. Codeine is an active ingredient in a lot of non-prescription analgesic medications, e.g. Solpadeine etc Biological Information: Genetics, Heredity and DNA Lectures 26-37 Key concepts that will be covered: * Introduction to genetics: history, overview, applications * Mendelian genetics * Linkage and recombination * Identification of DNA as the hereditary material * Quantitative genetics * DNA, structure and function * DNA – mutation, recombination and repair * The central dogma MCQ evaluation Matthew Campbell [email protected] Lecture 2 (L27) 2018 Mendelian Genetics Biology - A Global Approach (11th edition, Pearson) Chapter 14 – Mendelian Genetics Campbell, Urry, Cain, Wasserman, Minorsky & Reece Genetics has many applications – here are just a few examples relating to human health (as per 1st Genetics lecture) 1. Production of safer vaccines: recombinant single subunit vaccines e.g. hepatitis B vaccine 2. Production of recombinant human “therapeutic” proteins e.g. insulin, growth hormone, clot dissolving proteins 3. Inherited disorders can be diagnosed prenatally 4. Prenatal genotyping – in vitro fertilisation & pre implantation diagnosis 5. Pharmacogenomics: using genomics to genotype populations & individuals for alleles that determine responsiveness to drug therapies. 6. Forensics - role of genetic information in resolving crimes, person identification etc....CSI of sorts....and many others Some Basics First! What is Genetics? - the study of inheritance - the flow of biological information from one generation to the next Information about what? - How to construct a living organism - How the organism’s physical characteristics are determined The Information Flow: Inheritance - Is it governed by rules? - If so, what are these rules? - How can the rules be discovered? Uncovering the Rules of Inheritance: A General Strategy Mate or “cross” 2 individuals that show different forms of the same physical trait Trait Parent 1 Height Tall Hair color Dark Eye color Blue Disease X Disease X X Parent 2 Short Blond Brown Healthy Ask: 1. How many distinct types of offspring? 2. How frequent is a given type? 3. Is there a predictable pattern of inheritance of the traits? (Refer to the dominant, recessive & sex-linked patterns of inheritance in humans outlined in Lecture 1 (L26)) In 1866 he published Experiments in Plant Hybridization, in which he established his three Principles of Inheritance Work was largely ignored for 34 years, until 1900, when 3 independent botanists rediscovered Mendel’s work. Mendel was the first biologist to use maths to explain his results quantitatively Mendel predicted the concept of genes Predicted that genes occur in pairs Predicted that one gene of each pair is present in the gametes TCD M - Rhodopsin gene - 3q Mendel studied 7 traits Gregor Mendel (1822 – 1884) Flower color Flower position Seed color Seed texture Pod texture Pod color Plant height Ref: You Tube Gregor Mendel Rap Before Mendel’s work (1860’s), no consistently predictable patterns of inheritance were observed. Mendel’s successful experimental strategy: 1. Choose an organism that is known to “breed true” i.e. when crossed with itself it gives only offspring that are the same as itself 2. Choice of experimental organism: pea (Pisum sativum) Why? a. Many physically distinct varieties available b. Varieties with alternative forms of a given trait Additional reasons for Mendel’s success 1. 2. He investigated the inheritance of only one or two traits at a time He kept accurate quantitative data about: (a) how many different types of offspring were obtained from a cross (b) the frequency of occurrence of the various types F1 Pp x Pp F2 PP Pp pP pp Mendel’s First Experiments Inheritance of a single trait = monohybrid cross Parental generation P1 purple F1 offspring generation Let F1 plants self-fertilize = F2 offspring generation P2 X white all purple F1 X F1 purple: 705 white: 224 Ratio: purple : white = 3 : 1 Each of the 7 individual traits that Mendel studied was inherited in the same way! P1 X P2 F1 offspring show one parent’s phenotype only said to be the DOMINANT phenotype F1 X F1 F2 reappearance of the second phenotype called the RECESSIVE phenotype Ratio of DOMINANT : RECESSIVE = 3 : 1 Mendel’s explanation – some lateral thinking! 1. Each trait is determined by a pair of unit factors which we now call genes Why a pair? Because each parent supplies one 2. There are two alternative forms = alleles of each gene that determines a given trait: a dominant allele a recessive allele 3. The two alleles segregate randomly during gamete formation i.e. the male and female gametes each contain one allele This is known as Mendel’s “law of segregation” 4. During fertilization, when an egg cell fuses with a sperm cell, the resulting zygote contains one allele from each parent 5. Since there are two alleles of each gene, there are three ways in which pairs of allele can be combined e.g. Flower colour: Dominant P allele + Recessive p allele GENOTYPE 1 PP homozygous Pp heterozygous Pp heterozygous PHENOTYPE Pp x Pp F1 PP Pp pP pp F2 3 2 1 pp homozygous 1 Alleles Allele for purple flowers Locus for flower- color gene Allele for white flowers Figure 14.4 CTAAATCGGT GATTTAGCCA CTAAATCGGT Pair of homologous chromosomes ATAAATCGGT TATTTAGCCA ATAAATCGGT Enzyme Enzyme that helps synthesize purple pigment Absence of enzyme One allele results in sufficient pigment. Alternative versions of genes account for variations in inherited characters. Alternative versions of a gene are termed alleles. 3 Purple Purple Phenotype Purple Genotype PP (homozygous) Pp (heterozygous) Pp (heterozygous) pp (homozygous) Ratio 1:2:1 1 2 1 White Ratio 3:1 1 Figure 14.6 ©2018PearsonEducationLtd. Useful Genetic Vocabulary An organism with two identical alleles for a character is called a homozygote. It is said to be homozygous for the gene controlling that character. An organism with two different alleles for a gene is a heterozygote and is said to be heterozygous for the gene controlling that character. Unlike homozygotes, heterozygotes are not true-breeding. An organism’s traits do not always reveal its genetic composition. Therefore, we distinguish between an organism’s phenotype, or physical appearance, and its genotype, or genetic makeup. In the example of flower color in pea plants, PP and Pp plants have the same phenotype (purple) but different genotypes Figure 14.3 Experiment P Generation (true-breeding parents) × Purple flowers flowers F1 Generation (hybrids) All plants had purple flowers Self- or cross-pollination F2 Generation 705 purple-flowered 224 white-flowered plants plants White Mendel observed the same pattern of inheritance for all 7 characteristics. A 3:1 ratio was observed for each trait in the F2 generation. Mendel’s model: * Alternative versions of genes account for variation in inherited characteristics * For each character, an organism inherits two copies (two alleles) of a gene, one from each parent * If the two alleles at a locus (gene) differ then: a. the dominant allele determines the organism’s appearance b. the recessive allele has no noticeable effect on the organism’s appearance * The Law of Segregation: the two alleles for a heritable character segregate (separate from each other) during gamete formation and end up in different gametes (Mendel used terms such as unit factors rather than genes or alleles but the underlying principles remain the same) Figure 14.7 Technique TEST CROSS An individual with the dominant phenotype could be either homozygous dominant or heterozygous. To determine the genotype we can carry out a testcross: breeding the mystery individual with a homozygous recessive individual. If any offspring display the recessive phenotype, the mystery parent must be heterozygous. Dominant phenotype, unknown genotype: PP or Pp? Predictions If purple-flowered × Recessive phenotype, known genotype: Results Eggs Pp Pp Pp Pp Eggs Pp Pp pp pp parent is PP: Sperm PP P p All offspring purple 1 2 offspring purple and or pp If purple-flowered parent is Pp: Sperm pp pp or 1 2 offspring white What about the inheritance of two traits? A di-hybrid cross Will the inheritance of one influence the inheritance of the other? i.e. dependent or independent inheritance: which? Seed colour – yellow (Y) or green (y) Seed shape – Round (R) or wrinkled (r) This doesn’t happen! Just 2 sperm & ova types: YR and yr (Dependent inheritance) Mendel’s 2nd Law - The Law of Independent Assortment Mendel identified his second law of inheritance by following two characters at the same time Crossing two true-breeding parents differing in two characters produces dihybrids in the F1 generation, heterozygous for both characters A dihybrid cross, a cross between F1 dihybrids, can determine whether two characters are transmitted to offspring as a package or independently © 2018 Pearson Education Ltd. yellow (Y) green (y) Round (R) wrinkled (r) 4 types egg cells 4 types sperm Mendel’s second law: “Independent Assortment” Each trait is inherited independently of the other 9: 3: 3: 1 ratio © Solving Complex Genetics Problems with the Rules of Probability We can apply the rules of probability to predict the outcome of crosses involving multiple characters A multicharacter cross is equivalent to two or more independent monohybrid crosses occurring simultaneously In calculating the chances for various genotypes, each character is considered separately, and then the individual probabilities are multiplied 2 0 18PearsonEducationLtd. ØWhere does the 9:3:3:1 ratio come from? From the independent probabilities of inheriting a given allele Single probabilities: Seed color Seed shape probability of Yellow probability of green probability of Round probability of wrinkled 3/4 3:1 ratio 1/4 3/4 3:1 ratio 1/4 = 3/4 X 3/4 = 9/16 = 3/4 X 1/4 = 3/16 = 1/4 X 3/4 = 3/16 = 1/4 X 1/4 = 1/16 Combined probabilities: Probability of Yellow AND Round Yellow AND wrinkled green AND Round green AND wrinkled Experiment P Generation F1 Generation Predictions Predicted offspring of F2 generation YYRR yyrr Gametes YR × yr Hypothesis of dependent assortment YyRr Hypothesis of independent assortment Sperm 1 4 YR 1 4 Yr 1 4 yR 1 4 yr 1 4 YR YYRR YYRr YyRR YyRr Figure 14.8 12 YR Eggs 1 4 Yr Eggs 14 yR 14 yr 9331 16 16 16 16 Phenotypic ratio 9:3:3:1 or Sperm 12 YR 12 yr YYRR YyRr 12 yr YyRr yyrr 31 44 Phenotypic ratio 3:1 YYRr YYrr YyRR YyRr yyRR yyRr YyRr Yyrr yyRr yyrr YyRr Yyrr Results 315 108 101 32 Phenotypic ratio approximately 9:3:3:1 Mendelian Disorders Given the rules of inheritance established from Mendel’s work with pea plants, the way in which many human diseases are inherited has been termed Mendelian as outlined in Genetics Lecture 1 (L26). When mutations in a single gene cause a disease, the disease is said to be Mendelian. There are over 3,000 Mendelian disorders in humans. Examples of Mendelian disorders include: Cystic Fibrosis (CF) , Leber’s congenital amaurosis (LCA) – recessive disease. Huntington’s disease (HD) – dominant disease. Haemophilia – sex-linked disease Dominant Recessive http://ucsdnews.ucsd.edu/pressrelease/uc_san_diego_health_system_named_center_ http://learn.genetics.utah.edu/content/disorders/singlegene/hunt/ of_excellence_for_huntingtons_disease Huntington’s Disease – Autosomal Dominant Inheritance © 2018 Pearson Education Ltd. Inheritance patterns can be more complex than predicted by simple Mendelian genetics The relationship between genotype and phenotype at times is not as simple as in the pea plant characters Mendel studied Many heritable characters are not determined by only one gene with two alleles However, the basic principles of segregation and independent assortment apply even to more complex patterns of inheritance Inheritance of characters by a single gene may deviate from simple Mendelian patterns in the following situations: When alleles are not completely dominant or recessive When a gene has more than two alleles When a gene produces multiple phenotypes © 2018 Pearson Education Ltd. Degrees of Dominance Complete dominance occurs when phenotypes of the heterozygote and dominant homozygote are identical In incomplete dominance, the phenotype of F1 hybrids is somewhere between the phenotypes of the two parental varieties In codominance, two dominant alleles affect the phenotype in separate, distinguishable ways Multiple Alleles Most genes exist in populations in more than two allelic forms For example, the four phenotypes of the ABO blood group in humans are determined by three alleles for the enzyme that attaches A or B carbohydrates to red blood cells: I The enzyme encoded by the allele adds the A carbohydrate, whereas the enzyme encoded by A B , I , and I AI the carbohydrate; the enzyme encoded by the neither BI allele adds the B i allele adds Pleiotropy Most genes have multiple phenotype effects, a property termed pleiotropy This feature particularly obvious for syndromic diseases where a mutation in one gene can cause defects in multiple tissues. For example, Usher syndrome (hearing and vision loss), Bardet Biedl syndrome (mental retardation, vision loss and polydactyly & others. L2 (L27) Mendelian Genetics The laws of inheritance established by Gregor Mendel, the Father of Genetics, in the 1860s are relevant to the inheritance of genetic disorders today. All of us carry recessive alleles for some genetic disease & on average we carry X recessive disease alleles! Localising genes to chromosomes – let’s see how it is done! Linkage data from CF gene on Ch 7 in 1986 For example: The CF gene is located on human chromosome 7q31.2 How was this gene localised? Linkage & recombination is central to this. 10-100 trillion cells: To maintain the tissues which are being replaced daily, hundreds of billions of cells divide each day. DNA damage, due to environmental factors and normal metabolic processes occurs to cells each day. It is estimated that 10,000 - 1,000,000 molecular lesions occur per cell per day. These lesions / mistakes in DNA need continually to be fixed if we are to maintain the integrity of our genomes. It is incredible we are not disintegrating! Genome ‘spell check’ and DNA repair mechanisms are essential. Cell division involving somatic cells is termed Mitosis Cell division involving gametes (egg & sperm cells) is termed Meiosis The Cell Division Cycle Almost 90% of the cycle is taken up with Interphase during which DNA in the nucleus is replicated Mitosis and cytokinesis only take up 10% of the cycle There are trillions of cells in the human body. At least 5 trillion cell divisions required from fertilised eggàadult human. A copy of 3 x 109 bases of DNA is required for each daughter cell – each copy must be accurate. Some mistakes can lead to disease. The replication machinery is enormous – there are hundreds of proteins involved in replicating the genome. Some of the machinery involved in DNA replication & editing/repair (Kevin Devine will deal with DNA repair in later lectures) DNA ligase, an enzyme, encircles the DNA helix and repairs a broken strand of DNA (http://en.wikipedia.org/wiki/File:DNA_Repair.jpg It is believed that somewhere between 1,000 – 1,000,000 molecular lesions occur per cell per day. Efficient repair systems are essential! 14th May 2013 - Angelina Jolie Inherited form of breast cancer & the BRCA1 gene BRCA1 & BRCA2 proteins (encoded by the BRAC1 & BRAC2 genes) are normally expressed in breast cells & other tissues, where they help repair damaged DNA, or destroy cells if DNA cannot be repaired. They are involved in the repair of chromosomal damage with an important role in the error-free repair of DNA double-strand breaks. If BRCA1 or BRCA2 are mutant, damaged DNA is not repaired properly greatly increasing the risk of breast cancer. Recap: Mitosis & Meiosis – 2 types of cell division (Fred Sheedy’s lectures L4-6) Cell Division: MITOSIS MEIOSIS Meiosis: how the chromosome set is halved A diploid cell One pair of homologous chromosomes Replication of each chromosome two sister chromatids Meiosis I Each cell receives only one of the homologues Meiosis II Each cell receives only one sister chromatid The consequences of crossing over during meiosis: recombination Crossing over during Meiosis (during gamete formation) is central to the concepts of linkage and recombination that we are going to explore in this lecture. Crossing over in essence results in some random shuffling of genetic material during the process of gamete formation. During prophase I, homologous chromosomes pair up with each other. This pairing involves the synaptonemal complex (a protein scaffold) that joins the homologous chromosomes along their lengths. Cohesin crosslinking occurs between the homologous chromosomes & helps them resist being pulled apart until anaphase. click4biology.info/c4b/10/images/10.1/cross-over-main.gif Recombination/ crossing over is one of the key concepts in genome mapping including mapping human disease genes. Let’s look at why this is the case... Electron Micrograph (EM) of Chiasmata Crossing over at Chiasmata during Meiosis http://ib.bioninja.com.au/higher-level/topic-10-genetics-and-evolu/101- meiosis/chiasmata.html Linkage and Recombination (Understanding the basis of genome mapping) Finding Disease Genes Retinitis Pigmentosa (RP) – dominantly inherited eye disease Cause: Rhodopsin gene on chromosome 3q An Irish family with an autosomal dominant disease mutation causing blindness termed RP. A large family like this is powerful (statistically) & can be used to identify which human chromosome the disease gene is on. These 2 lectures are about the basis of genetic linkage mapping which allows us to localise disease genes. Central to this is the concept of crossing over / recombination during Prophase I of Meiosis as discussed in with Fred Sheedy. However let’s go thru’ some background first... How was it first established that genes are located on Chromosomes? Cytologists worked out the process of mitosis in 1875 & meiosis in the 1890s using improved techniques of microscopy. In the early 20th century, biologists realised that chromosomes behaved just like the hypothetical “unit factors” proposed by Mendel to explain inheritance. Around 1902, Sutton and Boveri and others independently noted these parallels and began to develop the chromosome theory of inheritance - they occur in pairs in diploid cells - their number is halved during gamete formation - fertilisation restores the diploid number They eventually proved that genetic information (genes) is located on the chromosomes Mendel’Studies Mendel investigated the inheritance of 7 traits Each trait showed “independent assortment” i.e. the inheritance of one trait did not influence the inheritance of the second trait Because of this, he observed the following ratios in the offspring 1. Monohybrid cross (inheritance of a single trait) 3 : 1 (dominant : recessive) 2. Dihybrid cross (inheritance of two traits) 9 :3 :3 :1 Figure 15.2b F1 Generation LAW OF SEGREGATION The two alleles for each gene separate. R R ry ry YY Meiosis Metaphase I All F1 plants produce yellow round seeds (YyRr). LAW OF INDEPENDENT ASSORTMENT Alleles of genes on nonhomologous chromosomes assort independently. R Anaphase I Yy Yy r Metaphase r R II RrYy rRYy 1r rR1 R 2Yyyy2 YYyyYYyy RRrrrrR R 1 4 YR 1 4 yr 1 4 Yr 1 4 yR Is “independent assortment” always the case? NO it depends on whether the genes are linked or not (a) Genes located on different chromosomes are NOT linked This allows independent assortment -in a di-hybrid cross the traits show the classic 9:3:3:1 inheritance pattern (b) Genes that are located very close together on the same chromosome may show complete linkage They may be so close to each other that they cannot be separated by recombination (crossing-over) during meiosis (c) Genes located far apart on the same chromosome typically show incomplete (partial) linkage because they are easily separated by recombination (crossing-over) Linkage Humans contain approximately 20,000 genes (haploid number) But there are only 23 chromosomes (diploid number 2n = 46) Clearly, there are hundreds / thousands of genes on a given chromosome All of the genes on a given chromosome are said to be linked or to belong to the same linkage group IMPLICATIONS OF LINKAGE Since chromosomes are the units that are distributed into gametes during meiosis, if 2 genes are located on the same chromosome they will tend to be inherited together whereas genes located on different chromosomes will show independent inheritance. © 2018 Pearson Education Ltd. Morgan showed that chromosomes are the location of Mendel’s heritable factors The first solid evidence associating a specific gene with a specific chromosome came in the early 1900s from the work of Thomas Hunt Morgan His early experiments provided convincing evidence that the chromosomes are the location of Mendel’s heritable factors Morgan chose to study Drosophila melanogaster, a common species of fruit fly. Several characteristics make fruit flies a convenient organism for genetic studies: They produce many offspring A generation can be bred every two weeks They have only four pairs of chromosomes Morgan & traits in Drosophila Wild type Mutant Thomas Hunt Morgan noted that wild type, or normal, phenotypes that were common in the fly populations Traits alternative to the wild type are called mutant phenotypes The first mutant Morgan discovered was a fly with white eyes instead of the wild-type red eyes Experiment Conclusion P × PXw+ Xw Generation Generation X Eggs Generation ×Y w+ w+ w w+ w+ w+ ww w+ F1 Generation Results F2 Generation All offspring had red eyes. + w Data from T. H. Morgan, Sex-limited inheritance in Drosophila, Science 32:120–122 (1910). Figure 15.4 F1 w+ Generation w F2 w w+ w+ Eggs Sperm Sperm Morgan’s discovery of a trait that correlated with the sex of flies was key to the development of the chromosome theory of inheritance © 2018 Pearson Education Ltd. The Chromosomal Basis of Sex We now know... Humans & other mammals have two types of sex chromosomes: a larger X chromosome & a smaller Y chromosome. A person with two X chromosomes develops as a female, while a male develops from a zygote with one X & one Y Short segments at the ends of the Y chromosomes are homologous with the X, allowing the two to behave like homologs during meiosis in males A gene on the Y chromosome called SRY (sex-determining region on the Y) is responsible for development of the testes in an embryo A gene that is located on either sex chromosome is called a sex-linked gene Genes on the Y chromosome are called Y-linked genes Only 78 genes, coding for about 25 proteins, have been identified on the human Y chromosome Genes on the X chromosome are called X-linked genes; the human X chromosome contains about 1,100 genes X Y Over 3,000 single gene disorders / Mendelian disorders in humans Disorder Frequency per 1000 births Examples of x-linked recessive diseases: Fragile X syndrome 0.5 Duchenne muscular dystrophy 0.3 X-linked ichthyosis 0.2 Haemophilia A 0.1 Becker muscular dystrophy 0.05 Haemophilia B 0.03 Also see Genetics lecture 1 (L26) These human disease traits are located on the X chromosome in the same way that Morgan’s eye colour trait mapped to the X chromosome in Drosophila As an aside: X Inactivation in Female Mammals In mammalian females, one of the two X chromosomes in each cell is randomly inactivated during embryonic development. The inactive X condenses into a Barr body. If a female is heterozygous for a particular gene located on the X chromosome, she will be a mosaic for that character. In the cat, the allele for black fur is on one X chromosome, while the allele for orange fur is on the other X chromosome. Due to X-inactivation a mixed coat colour is seen. Morgan showed that some traits locate to the X chromosome. How do we link/map genetic traits to any chromosome? Each chromosome has hundreds or thousands of genes (except the Y chromosome) – see slide 21. Genes that are located on the same chromosome tend to be inherited together and are called linked genes – see slide 21. Morgan did experiments with fruit flies to see how linkage affects inheritance of two characters. Morgan crossed flies that differed in traits of body color and wing size. The first cross was a P generation cross to generate F1 dihybrid flies. The second was a testcross. Morgan was working with Drosophila however the same methods are used to map human genes. Let’s look at this di-hybrid cross between 2 fruit flies - (Drosophila melanogaster) Morgan used the fruit fly, as a model system, however, the same rules apply to inheritance of two human traits. Trait 1 = body colour grey (wild-type) b+ black (mutant) b DOMINANT recessive Trait 2 = wing size large (wild-type) vg+ vestigial (mutant) vg DOMINANT recessive Expected if genes are NOT linked there are: 50% parental types 50% non-parental or recombinant types (Mendel’s 2nd law of independent assortment) If genes are linked: There are more parental types (greater than 50%) and less non-parental types (less than 50%) Morgan found that body color and wing size are usually inherited together in specific combinations (parental phenotypes). He noted that these genes do NOT assort independently & reasoned that they were on the same chromosome © 2018 Pearson Education Ltd. Summary Information I Offspring with a phenotype matching one of the parental (P) phenotypes are called parental types Offspring with nonparental phenotypes (new combinations of traits) are called recombinant types, or recombinants A 50% frequency of recombination is observed for any two genes on different chromosomes – independent assortment © 2018 Pearson Education Ltd. Summary Information II Morgan observed that although some genes are linked, at times nonparental allele combinations are still produced He proposed that some process must occasionally break the physical connection between genes on the same chromosome That mechanism was the crossing over of homologous chromosomes Experiment P Generation (homozygous) Wild type (gray body, normal wings) b+ b+ vg+ vg+ F1 dihybrid testcross Wild-type F1 dihybrid (gray body, normal wings) × Double mutant (black body, vestigial wings) b+ b vg+ vg Testcross offspring Eggs b+ vg + Wild type b vg b+ vg Gray- Black- b vg+ (gray-normal) vestigial vestigial normal × Homozygous recessive (black body, vestigial wings) b b vg vg bbvgvg PREDICTED RATIOS Genes on different chromosomes: Genes on the same chromosomes: Results b+ bvg+ vg b bvgvg b+ bvgvgb bvg+ vg 1:1:1:1 1:1:0:0 965 : 944 : 206 : 185 b vg Sperm Data from T. H. Morgan and C. J. Lynch, The linkage of two factors in Drosophila that are not sex-linked, Biological Bulletin 23:174–182 (1912). Figure 15.9 Black- Sometimes a crossing over event during meiosis will occur giving rise to new non- parental (recombinant) genotypes: b+ vg and b vg+ b+ vg+ b vg Data from T. H. Morgan and C. J. Lynch, The linkage of two factors in Drosophila that are not sex-linked, Biological Bulletin 23:174–182 (1912). Why so many parental types in the offspring? The 2 genes are not showing independent assortment because they are linked i.e. are on the same chromosome Mother Father Why are there any non-parental types (i.e. recombinants)? Answer: In this case because of recombination/crossing over in the female fly Meiosis II Cross overs between non-sister chromatids (crossover between homologous chromosomes) Types of gametes produced F1 dihybrid testcross b+ vg+ bvg b+ vg+ b+ vg+ b vg b vg b+ vg+ b+ vg b vg+ b vg b vg Wild-type F1 dihybrid (graybody, normal wings) Homozygous Meiosis I b vg b vg b vg b vg Meiosis II b+ vg+ Meiosis I and II Recombinant chromosomes recessive bvg (blackbody, vestigial wings) Eggs b vg b+ vg b vg+ b vg Figure 15.10b Sperm Meiosis II b+ vg+ Recombinant chromosomes Eggs Testcross offspring bvg 944 Black- vestigial b vg b vg b+ vg 206 Gray- vestigial b+ vg b vg bvg+ 185 Black- normal b vg+ b vg 965 Wild type (gray normal) b vg Sperm b+ vg+ b vg Parental-type offspring Recombinant offspring Recombination = 391 recombinants × 100 = 17% frequency 2,300 total offspring The same rules apply for two human traits on the same chromosome, e.g., Haemophilia & Glucose-6-Phosphate Dehydrogenase deficiency on the X chromosome – two X-linked recessive disorders A http://www.uic.edu/classes/bms/bms655/lesson12.html Haemophilia A (Factor VIII) – bleeding disorder & G6PD deficiency – hemolytic anemia A: Female heterozygous carrier of both Haemophilia A & G6PD deficiency. B: Chromosomes have paired & homologous chromosomes have exchanged sections (Meiosis Prophase I) Next lecture Recap from prior lectures Meiosis Prophase I (Crossing-over / recombination) Homologous chromosomes (each consisting of two sister chromatids) come together as pairs The structure formed is called a tetrad Chromosome segments are swapped between non-sister chromatids at cross-over points called chiasmata (= crossing-over) During prophase I, homologous chromosomes pair up with each other. This pairing involves the synaptonemal complex (a protein scaffold) that joins the homologous chromosomes along their lengths. Cohesin crosslinking occurs between the homologous chromosomes & helps them resist being pulled apart until anaphase. click4biology.info/c4b/10/images/10.1/cross-over-main.gif Meiosis Prophase I - Crossing-over / recombination www.phschool.com/science/biology_place/labbench/lab3/images/crossovr.gif Crossing over at Chiasmata http://o.quizlet.com/zdQlD.a-oL5oBu-x965H8w_m.jpg Recombination/ crossing over is one of the key concepts in genome mapping including mapping human disease genes. Let’s look at why this is the case... Electron Micrograph (EM) of Chiasmata Crossing over at Chiasmata during Meiosis http://ib.bioninja.com.au/higher-level/topic-10-genetics-and-evolu/101- meiosis/chiasmata.html click4biology.info/c4b/10/images/10.1/cross-over-main.gif Lots of genes & encoded proteins involved in control of recombination/crossing over in meiosis A large cellular machinery is involved in mediating the process of crossing over between homologous chromosomes Jillian L. Youds, and Simon J. Boulton J Cell Sci 2011;124:501-513 Genetic maps or linkage maps Alfred Sturtevant, one of Morgan’s students, proposed that recombination frequencies reflect the distances between genes on a chromosome It is assumed that the chances of crossing over is equal at all points on a chromosome If so, then the farther apart two genes are: (a) the higher the probability that a cross-over will occur between them and therefore (b) the higher the recombination frequency Why? Because the greater the distance between two genes the more points there are between them where crossing over can occur Sturtevant constructed a genetic map, an ordered list of the genetic loci along a particular chromosome Sturtevant predicted that the farther apart two genes are, the higher the probability that a crossover will occur between them and therefore the higher the recombination frequency A linkage map is a genetic map of a chromosome based on recombination frequencies Distances between genes can be expressed as map units; one map unit represents a 1% recombination frequency (approx. 1 million bases of DNA) Map units indicate relative distance and order, not precise locations of genes Recombination frequencies can be used to construct a genetic map Recombination frequencies are approximately additive Recombination frequency between the body colour gene black (b) and the eye colour gene cinnabar (cn) = 9% Recombination frequency between cinnabar and vestigial wing (vg) = 9.5% Recombination frequency between b and vg = 17% 1% recombination equates to approx. 1 million base pairs of DNA (also termed 1 centiMorgan (cM) after the scientist, Thomas Hunt Morgan) Sturtevant used recombination frequencies to make linkage maps of fruit fly genes He and his colleagues found that the genes clustered into four groups of linked genes (linkage groups) The linkage maps, combined with the fact that there are four chromosomes in Drosophila, provided additional evidence that genes are located on chromosomes © 2018 Pearson Education Ltd. Y II IV III I X Mutant phenotypes Maroon Black Cinnabar Vestigial Down- Brown Short aristae 0 eyes 16.5 body eyes wings curved eyes wings 48.5 57.5 67.0 75.5 104.5 Figure 15.12 Long aristae (appendages on head) Gray Red Normal body eyes wings Wild-type phenotypes Normal Red wings eyes Red eyes An example of gene identification by gene mapping. Cystic fibrosis: This is a genetic disease which has its world maximum prevalence in Ireland with 1/1800 births. It is recessive and involves chronic bacterial infection, inflammation of the lungs and high electrolyte level in sweat. The gene, which was unknown was known to influence chloride ion transport and mucus consistency on epithelial surfaces. This was the first gene identified through linkage analysis. www.cfireland.ie Symptoms of CF Very salty-tasting skin Persistent coughing, at times with phlegm; Frequent lung infections; Wheezing or shortness of breath; Poor growth/weight gain in spite of a good appetite; 1950s: Few children with CF lived to attend primary school. Today: Many people with the disease in Ireland can now expect to live into their 60s and well beyond. Orla Tinsley (@orlatinsley) · Twitter..TCGA.....AGCT....TCCA.....AGGT.. a) Cut DNA with restriction enzyme, eg TaqI b) Fragment size separation by gel electrophoresis. Fragment size is determined by cut/not cut of restriction enzyme. Individuals: 123 c) Visualisation of polymoprhism at particular gene by radioactive probing with specific marker DNA. Technique used is Southern blotting. First, linkage analysis using markers spread around the genome in many nuclear family pedigrees gave a region on chromosome 7 which was linked to the gene. This was bounded by recombinations with two markers: MET and D7S8. Allelic association 0.6 0.3 0.0 Then, researchers generated new markers within this 500 Kb segment and tested these for association with the disease. The association was strongest around a specific new gene. (this methodology is more successful with recessive diseases, dominant diseases are more likely to be heterogenous in causal mutations) Kerem et al. Science 1989 245:1073-80 3 RFLPs which showed strongest association, all within new cystic fibrosis transmembrane conductance regulator (CFTR) gene, close to a 3bp deletion on Chr 7. Detection of the delta F 308 mutation (deletion of codon for F) by oligo hybridisation It is only present in two copies in affected offspring, never in carrier parents. It is common, but not the only CF mutation. Sequencing of this gene revealed that it had deletions of 3 bp in some patients and analysis showed that it was involved in transmembrane ion transport. It was called the cystic fibrosis transmembrane conductance regulator (CFTR). CFTR regulates fluid flow within cells and affects the components of sweat, digestive fluids, and mucus. 68% of all chromosomes with a copy of the gene causing the disease show this same 3bp deletion. Its distribution is primarily western European. There is some evidence from the gene product’s involvement in ion transport and water retention that it may have been subject to natural selection in the past, with heterozygote advantage causing the high incidence of disease alleles in the present. Resistance to typhoid is one possibility (cells expressing wt CFTR internalise more S. typhi than those with two copies of the common mutant). Nearly 1 in 20 Irish people carry the CF gene!! There is potential for genetic therapy, but development of this is a slow process (especially for sufferers!). In principle an insertion of a wild type gene could correct the chloride conductance defect. Gene Therapy for CF? There is hope! A new agent, Ivacaftor (Kalydeco), was approved by the US Food and Drug Administration (FDA) in January 2012. Ivacaftor is a CFTR potentiator. It only works for the G551D mutation in which the protein is trafficked to the correct area, the epithelial cell surface, but once there the protein cannot transport chloride through the channel. Ivacaftor improves the transport of chloride through the ion channel by binding to the channels directly to induce a non-conventional mode of gating which in turn increases the probability that the channel is open Studies have shown lung function improvement at 2 weeks that was sustained through 48 weeks. The study also observed improvements in risk of pulmonary exacerbations, patient-reported respiratory symptoms, weight gain, and concentration of sweat chloride. The use of Ivacaftor for additional CFTR mutations is currently being studied as it only works for people with a mutation that accounts for 4–5% cases of cystic fibrosis. €234,000 per year!! Genes encoding the ABO blood group and Nail patella syndrome are localised to the same chromosome, 9. This one of the earliest documented chromosomal linkage identified in humans. The kids in the 3rd generation all are parental types except the individual identified with *, who is a recombinant. Human genome mapping The same approach has been used to generate maps of the human genome, i.e., to place one gene on a given chromosome relative to other genes on that chromosome. Mapping disease genes: To establish which chromosome a particular disease gene is on, such as the eye disease (RP) in the family below, one can look for co-inheritance or linkage between the disease gene & genes previously mapped to specific chromosomal regions. That is how in TCD we established that the rhodopsin (RHO) gene on chromosome 3 was the cause of some forms of RP. Genetic linkage map of human chromosome 3 - Genetic markers on chromosome 3. RHO is the Rhodopsin gene found to be causative of the eye disease in the Irish RP family in the prior slide. Somewhat similar information can now also be gained by analysising sequence data (from next Generation Sequencing (NGS)) Huntington’s Disease (HD) – Autosomal Dominant Neurodegenerative disease (recall from Genetics L2 (L27)) The HD gene is on human Chromosome 4 Woody Guthrie – died of HD. Father of Arlo Guthrie – ‘Alice’s Restaurant’ http://learn.genetics.utah.edu/content/disorders/singlegene/hunt/ http://ucsdnews.ucsd.edu/pressrelease/uc_san_diego_health_sy stem_named_center_of_excellence_for_huntingtons_disease Huntington disease gene on Chromosome 4p Huntington Disease (HD) - genetic linkage in a large family was used to locate the disease gene Part of a large Venezuelian HD family used to localise the HD disease gene to chromosome 4 by tracking on recombination / crossing over between the disease and known markers on chromosome 4 (published Nature 1983). http://ghr.nlm.nih.gov/dynamicImages/chromomap/HTT.jpeg Mutations in the Huntingtin gene causes an autosomal dominantly inherited fatal neurodegeneration called Huntington Disease (HD). A CAG expansion mutation in the gene causes the disease. Healthy people usually have between 10-35 CAG repeats in the HD gene. People with HD have between 36-120 CAG repeats. Each CAG encode for a glutamine amino acid in the Huntington protein. 1991 Locus segregating with familial AD mapped to chromosome 21 Families with late onset AD do not show linkage to chromosome 21 Some families with early onset AD show linkage while some don’t. Valine-isoleucine substitution Occurs within transmembrane domain of APP Makes transmembrane more hydrophobic Anchors it more firmly to the membrane Could impact its processing BUT! Because this gene/chromosome is not associated with other forms of AD, suggests that it is highly genetically heterogeneous. Alternating hemiplegia of childhood Rare neurological condition Recurrent episodes of temporary paralysis, often affecting one side of the body Paralysis can last minutes to days and begins in early childhood Mutations in the ATP1A3/ATP1A2 gene reduce the activity of Na+/K+ ATPase Defined as a channelopathy https://healthjade.net/alternating-hemiplegia-of-childhood/ Calcification in brain stem and basal ganglia Hashimoto et al., Brain, 2022 Arterial spin labelling Baseline Left hemiplegia Hashimoto et al., Brain, 2022 Dominant acting mutation in the coding sequence of CLDN5 Hashimoto et al., Brain, 2022 Tight junctions can form but electrical resistance is decreased Hashimoto et al., Brain, 2022 The barrier has formed an anion selective channel Hashimoto et al., Brain, 2022 Conclusions Mutation in the first extracellular loop of CLDN5 induces an anion selective BBB First description of a mutation in the coding sequence of CLDN5 Implications for drug delivery and treatment of hemiplegia A more complex genetic disease Somatic mutations Journey from single-celled zygote to adult human involves about 1014 mitotic cell divisions. Mutation is unavoidable...generally small number of cells If mutation can give rise to descendant cells that act abnormally. Glioblastoma multiforme Grade IV glioblastoma multiforme An attempt has been made (left) by Gerry Grant to totally resect this tumour – they always return. Glioblastoma multiforme: 20,000 deaths per annum in Europe/US combined Life expectancy from diagnosis, Approx. 12 months. Temozolomide (the current ʻwonder drugʼ) will increase survival by a couple of months. Tumours notoriously resistant to cytotoxic drug therapy because the drugs do not cross blood-tumor barrier. Summary Linkage and recombination during meiosis is central to mapping/localising genes. Genetic maps are based on tracking on meiotic recombination events between which occur in the generation of eggs and sperm and are evident from looking at parents and offspring. These methods have been used to localize many genes including many human disease genes. (1) Evidence that Genes are located on Chromosomes 1902 – McClung – a particular chromosome (X) determines sex in insects (XO = male; XX = female) 1903 – Sutton and Boveri – chromosomes behave just like the unit factors described by Mendel 1910 – Morgan – the white eye color gene of Drosophila is located on the X-chromosome - there are many other X-linked genes as discussed in Genetics L4(L29) (2) But what are Genes made of? 1869 – Meischer - he separated nuclei from cells - examined their chemical composition - extracted a phosphorus-rich polymer called nuclein - this was actually DNA 1910 – Morgan When Morgan’s group showed that genes are located on chromosomes, the two components of chromosomes - DNA and protein - became candidates for the genetic material 1912 – Feulgen - developed stains for detection of proteins - showed that the nucleus and chromosomes contained proteins as well as DNA (3) Problem: If chromosomes contain both DNA and proteins, which one is the genetic material (genes)? Arguments in favour of genes being made of proteins: Proteins are sufficiently complex to store genetic information but DNA is not Proteins - contain 20 different subunits (amino acids) and There are many different types of proteins DNA - contains only 4 different subunits The complexity found in proteins was considered sufficient to specify genetic complexity Arguments in favour of genes being made of DNA All cells of a given species contain a constant amount of DNA but the types and amounts of proteins differ in different cells The amount of DNA doubles in every cell just before it divides and an exactly equal amount is distributed to the two daughter cells Gametes contain half the number of chromosomes and half the amount of DNA Therefore, although DNA is a very simple molecule, it behaves in the way genetic material would be expected to behave During the 1940s the widely accepted conclusion was: § Genes are likely to be made of protein § DNA merely provides a structural framework in chromosomes Conclusive experimental evidence that genes are made of DNA came from studies of: (a) bacterial transformation and (b) virus replication Bacterial Transformation 1928 Griffith Streptococcus pneumoniae: two types S strains - virulent:caused lethal pneumonia in mice - can be isolated from blood of dead mice - bacterium has a polysaccharide coat - forms large, slimy colonies on agar plates R strains - non-virulent: doesn’t kill mice - doesn’t make a polysaccharide coat - grows as small, rough colonies on agar plates Griffiths experiments: inject mice with the different strain types observe effect on mice and analyse their blood for bacteria Strain injected Type II R Type III S killed Type III S killed Type III S + Type IIR Effect none dead all dead none dead all dead Blood analysis no bacteria present Type III S present no bacteria present Type III S present Pretty amazing idea to decide to test this last category! www.offresonance.com/wp-content/uploads/2008/05/450px-griffith_experimentsvg.png Explanation: Bacterial Transformation 1. The killed Type IIIS did NOT come back to life! 2. Genetic material contained in the Type IIIS cells has leaked out and entered the living Type IIR cells 3. The genetic material has converted (or transformed) cells of the Type IIR strain into Type III S cells 4. The genetic material from the Type III S contains the information for making the polysaccharide coat required for virulence – an example of horizontal gene transfer. 5. Griffith didn’t realise this! Horizontal gene transfer Horizontal gene transfer (HGT) is the movement of genetic material between unicellular and/or multicellular organisms other than by the vertical transmission of DNA from parent to offspring (reproduction). Transformation involves uptake of short fragments of naked DNA by naturally transformable bacteria. Transduction involves transfer of DNA from one bacterium into another via bacteriophages. Conjugation involves transfer of DNA via sexual pilus and requires cell-to-cell contact. Horizontal gene transfer Examples: * Griffith’s work (see prior slides). * Another example is the uptake of DNA fragments that contain resistance genes from resistant donors that can make previously susceptible bacteria express drug resistance encoded by the newly acquired resistance genes. Multidrug resistant (MDR) bacteria, e.g., multidrug resistant (methicillin resistant) Staphylococcus aureus (MRSA). MDR nosocomial (hospital acquired) infections represent a significant health risk. MRSA infection What is the transforming substance? Griffith’s discovery of bacterial transformation provided a way of identifying what chemical substance in the bacterium contained the genetic information i.e. the genes themselves 1944 Avery, McLeod and McCarthy Proved that the transforming substance = DNA 1. Made a soluble cell extract from Type IIIS cell cultures 2. Tested the cell extract for its ability to transform Type II R into Type IIIS. Before testing for transformation 3. Treated the extract with different enzymes i.e. (a) one that destroys proteins = protease or (b) one that destroys RNA = RNAase or (c) one that destroys DNA = DNAase Transformation Results A. Sextract alone B. S extract + protease C. Sextract+RNAase D. S extract + DNAase Transformation of TypeII R ? YES YES YES NO Transforming Substance ? NOT PROTEIN NOTRNA must be DNA Avery, McLeod and McCarthy www.visionlearning.com/library/modules/mid149/Image/VLObject-3756- 080922120939.jpg 1953 A second experiment confirms that DNA = The Genetic material The Hershey-Chase experiment: § Used a virus that infects and reproduces inside a bacterial cell = phage § The virus is made of 50% DNA and 50% protein § Question: which substance contains the genetic information? DNA contains phosphorus ( 32P) but no sulphur – can label the DNA with radioactive 32P Protein contains sulphur ( 35S) but no phosphorus can label protein with radioactive S Alfred Hershey and Martha Chase Experiment 1: The protein coat of the phage is labelled with 35S The DNA is not labelled. The protein coat stays on the surface of the bacterium and can be knocked off. After centrifugation (to pellet the cells) there is no 35S in the cells. Experiment 2: The DNA of the phage is labelled with 32P The protein is not labelled. The phage injects its DNA into the bacterial cell. When the cells are pelleted, the 32P is in the pellet. The progeny phage contain 32P Experiment 1 Labeled phages infect cells. 2 Agitation frees outside phage parts from cells. protein DNA 3 Centrifuged cells form a pellet. 4 Measured the radioactivity in the pellet and the liquid. Radioactive Empty Radioactivity (phage protein) found in liquid Phage Bacterial cell protein shell Batch 1: Radioactive sulfur (35S) in phage protein Phage DNA Centrifuge Batch 2: Radioactive phosphorus (32P) in phage DNA Radioactive DNA Pellet Centrifuge Radioactivity (phage DNA) found in pellet Pellet © 2018 Pearson Education Ltd. Figure 16.4 ©20 18P Additional Evidence That DNA Is the Genetic Material earsonEducationLtd. DNA is a polymer of nucleotides, each consisting of a nitrogenous base, a sugar, and a phosphate group The nitrogenous bases can be adenine (A), thymine (T), guanine (G), or cytosine (C) In 1950, Erwin Chargaff reported that DNA composition varies from one species to the next. This evidence of diversity made DNA a more credible candidate for the genetic material. Two findings became known as Chargaff’s rules: 1. The base composition of DNA varies between species 2. In any species the number of A and T bases is equal and the number of G and C bases is equal The basis for these rules was not understood until the discovery of the double helix. Figure 16.6 After DNA was accepted as the genetic material, the challenge was to determine how its structure accounts for its role in heredity. Maurice Wilkins & Rosalind Franklin were using a technique called X-ray crystallography to study molecular structure. Franklin produced a picture of the DNA molecule using this technique. Franklin’s X-ray crystallographic images of DNA enabled Watson to deduce that DNA was helical. The X-ray images also enabled deduction of the width of the helix & the spacing of the nitrogenous bases. The pattern in the photo suggested that the DNA molecule was made up of two strands, forming a double helix. © 2018 Pearson Education Ltd. Figure 16.5 DNA (deoxyribose) Nitrogenous base nucleotide © 2018 Pearson Education Ltd. Sugar–phosphate backbone 5′ end Nitrogenous bases Phosphate group Sugar 3′ end Thymine (T) Guanine (G) Cytosine (C) Adenine (A) DNA and RNA structures were discussed in L15 (Luke O’Neill) & will be discussed further in L32 (Kevin Devine) © 2018 Pearson Education Ltd. Sugar-phosphate backbone T CG GC G T Figure 16.UN03 GC CG AT A Nitrogenous bases CA Hydrogen bond © 2018 Pearson Education Ltd. A chromosome consists of a DNA molecule packed together with proteins The bacterial chromosome is a double-stranded, circular DNA molecule associated with a small amount of protein. In a bacterium, the DNA is “supercoiled” and found in a region of the cell called the nucleoid. Eukaryotic chromosomes have linear DNA molecules associated with a large amount of protein. In the eukaryotic cell, DNA is precisely combined with proteins in a complex called chromatin. Chromosomes fit into the nucleus through an elaborate, multilevel system of packing. Proteins called histones are responsible for the first level of packing in chromatin. Unfolded chromatin resembles beads on a string, with each “bead” being a nucleosome, the basic unit of DNA packaging. They are composed of two each of the four basic histone types, with DNA wrapped twice around the core of the eight histones. The N-termini (“tails”) of the histones protrude from the nucleosome. Nucleosomes, and especially their histone tails, are involved in the regulation of gene expression. Chemical modification to histones can change how tightly or loosely the DNA is packed and therefore can aid in controlling whether a gene will be expressed. Nucleosome (10 nm in diameter) H1 Figure 16.22 Chromatid (700 nm) DNA double helix (2 nm in diameter) HistonesHistone tail 30-nm fiber Looped Scaffold domain 300-nm fiber Replicated chromosome (1,400 nm) Figure 16.22 © 2018 Pearson Education Ltd. Chromatin ©2018Pear A chromosome consists of a DNA molecule packed together with proteins so nE du c at io nLt d. The N-termini (“tails”) of the histones protrude from the nucleosome. Nucleosomes, and especially their histone tails, are involved in the regulation of gene expression. Chemical modification to histones can change how tightly or loosely the DNA is packed and therefore can aid in controlling whether a gene will be expressed. Most chromatin is loosely packed in the nucleus during interphase and condenses prior to mitosis - loosely packed chromatin is called euchromatin. During interphase a few regions of chromatin (centromeres and telomeres) are highly condensed into heterochromatin. Dense packing of the heterochromatin makes it difficult for the cell to express genetic information coded in these regions ‘Epigenetics involves heritable changes in gene expression (active vs inactive genes) that are NOT due to changes to the DNA sequence - a change in phenotype without a change in genotype - which affects how cells read the genes’. https://www.whatisepigenetics.com/fundamentals/ When epigenetic modifications are dysregulated, it can result in disease, e.g., cancers, aging disorders etc. https://www.whatisepigenetics.com/fundamentals/ Epigenetics We need to consider not only the genetic code (the nucleotide sequence of DNA) but also the histone code. The chemical modifications to histone proteins & to DNA which influence this interaction & thereby can influence gene expression. www.naturalproductsok.com/sites/natural/uploads/images/header_images/epigenetic_markers. jpg Epigenetics & Disease The chemical modifications to DNA and histone proteins tend to show significant differences between healthy and diseased cells – see the example of cancer above. Abnormal epigenetic patterns are found in, e.g, ageing cells & many disease states. Lecture 28: DNA Structure and Replication Kevin Mitchell – Smurfit Institute of Genetics What’s different? 1 11/20/24 Organismal It has to be form is not recapitulated must contain some information directly replicated - some genetic material Properties required of the genetic material 1. It must contain the Information required to construct and maintain life forms 2. It must be Replicated with High Precision 3. Must be Inherited Intact through Generations 2 11/20/24 What is Life? An improbable pattern of matter – resistant to the 2nd Law of Thermodynamics: Must take in energy to stay organised Must have some information on how to be organised “Codescript” – an “aperiodic crystal” Stable, information-bearing Schrödinger’s codescript Must contain information on how the organism should be organised Must be chemically stable Must be replicable So that pattern of organisation can be recapitulated to create a new individual Bacteria can be transformed by some material from other strains Streptococcus pneumoniae (Griffiths, 1928) 3 11/20/24 DNA is the transforming (genetic) material (Avery, McCarty and MacLeod, 1944) Structure of the nucleotide subunits of DNA Alexander Todd (1907-1997) Nobel Prize, 1957 4 11/20/24 The sub-units of DNA: Nucleotides Nucleotide = Phosphate + Sugar + Base 4 kinds of Bases: PURINES: Adenine (A) Guanine (G) PYRIMIDINES: Cytosine (C) Thymine (T) Ø Structure of a nucleotide – key features 5’ phosphate 5’ 3’ hydroxyl 1’ 3’ 2’ Sugar = 2-deoxyribose 5’ 3’ 5 11/20/24 DNA is a polymer consisting of multiple ‘nucleotide’ subunits linked together This diagram shows only one of the two strands in a DNA molecule DNA is a polymer: The monomer is a nucleotide Four types of nucleotide: (d = deoxy) dAMP dGMP dCMP dTMP Monomer Base Phosphate Sugar The monomers link to each other to form a ‘Sugar-Phosphate’ backbone via phosphodiester bonds 6 11/20/24 Ø Only one kind of linkage is allowed between nucleotides 5’ phosphate Sugar 3’ hydroxyl Base Base Base Phosphodiester bond Sugar Phospho3d’iehsytedr rboxnydl Sugar 3’ hydroxyl Sugar Base 5’ Phosphate end The DNA strand has ‘directionality’ based on the different ‘end-groups’ 3’ Hydroxyl end OH What is the 3-D structure of DNA? What does it tell us about the molecular nature of life? 7 11/20/24 What is the 3-D Structure of DNA? Rosalind Franklin DNA X-Ray Diffraction Speculation & model building & Maurice Wilkins James Watson & Francis Crick The DNA structure as revealed by X-ray analysis and model building 1. DNA is a 2-stranded helical structure with the sugar-phosphate backbones on the outside 2. The bases are stacked on the inside, perpendicular to the helix axis 3. Helical repeat unit = 34oA (10 stacked base pairs per helix turn) The key question about the 3-D structure: How are the four kinds of bases arranged within the DNA double helix? 8 11/20/24 Ø A first clue concerning the arrangement of the bases Erwin Chargaff 1905-2002 Base composition and Base ratios in DNA Chargaff: DNA base composition & base ratios E. coli Diplococcus Mycobacterium Yeast Sea urchin Chargaff’s rules: A = T G=C Alternatively stated: A + G = T + C Key proposals of Watson & Crick: (1) The only base pairs permitted are: G : C and A : T (2) Base pairing, via hydrogen bonds, directs replication! GC GC A A TT 9 11/20/24 (3) Crick: The strands are in an anti-parallel orientation 5’ 3’ Hydrogen bonds 3’ 5’ DNA Different ways of depicting the double helix Vertical representations: Bases not shown Double-stranded DNA can also be represented horizontally: 5’ 3’ Bases not 5’ shown 3’ 5’ 3’ 5’ 3’ 5’ 3’ 5’ 3’ 10 11/20/24 Replication of DNA is tightly regulated The cell cycle Synthesis Mitosis Growth Replication of DNA The total DNA content (genome) of the cell must be: - Replicated with great precision (very few mistakes ! ) - A copy of all the genetic material must be be present in each daughter cell after cell division Major Questions: Replication – how is the DNA double helix replicated ? Genome duplication – how is an entire genome replicated ? MODELS OF DNA REPLICATION Hypothesis 1: Hypothesis 2: Hypothesis 3: Semi-conservative replication Conservative replication Dispersive replication Intermediate molecule 11 11/20/24 Hypothesis 1: Each strand acts as a template on which the other strand is synthesized The two strands separate Ø The Meselson-Stahl experiment 1. Grow E. coli cells for many generations in N-15 medium; N-15 N-14 (their DNA will be labelled with heavy N-15) 2. Centrifuge culture to obtain the cells 3. Transfer the N-15 cells into fresh N-14 growth medium The cells will replicate their DNA every 20 min New DNA strands will be labelled with light N-14 4. Extract DNA - investigate its density (banding position) in a CsCl gradient Ø Replication of DNA is semi-conservative Time: Replic: Replic: No DNA in the N-14 position DNA band at N-15 position No band in the N-14 position No band in the N-15 position Band in the N-14 position No band in the N-15 position N-15 N-15/N-14 hybrid DNA N-14 12 11/20/24 Role of DNA polymerase in replication Ø DNA polymerase can’t start a new DNA strand on its own Rule 1: DNA polymerase can only add nucleotides to a pre-existing strand (a primer strand) that is already base-paired with a template strand DNA pol adds nucleotides that extend the primer Conclusion: DNA synthesis occurs via ‘primer extension’ Template strand only: No replication 5’ 3’ RNA primer Template strand with RNA primer 5’ base-paired to it 5’ 3’ 3’ new DNA 5’ 3’ 5’ 3’ Rule 2: DNA polymerase can only add nucleotides to the 3’ end of a replicating strand 5 min 10 min 15 min 5’ 5’ 5’ 3’ 3’ new 5’ 3’ Template strand 3’ 5’ 3’ Template strand 5’ 3’ Template strand For this reason, DNA synthesis is said to occur exclusively in a 5’ to 3’ direction 13 11/20/24 Ø DNA replicates via ‘template-directed’ base-pairing of nucleotides and step-wise creation of phosphodiester bonds 5’ 3’ = template strand 3’ 5’ 3’ Replication as an in vivo process: a. The Replication Fork Replication occurs on both DNA strands The junction of the unwound molecules is a Replication Fork Each strand acts as a ‘template’ on which to build a new strand New strands are formed by pairing complementary bases with each pre-existing template strand Two molecules are made. Each has one new and one old strand Double-stranded DNA unwinds 14 11/20/24 Ø A MAJOR conceptual problem in DNA replication Consider one Replication Fork: 5’ Direction of unwinding 3’ 3’ 5’ 3’ Continuous replication on this strand How is this strand replicated? 5’ RNA Primer Proposal: the other strand is replicated “backwards and discontinuously” 5’ Direction of unwinding 3’ 5’ 3’ Continuous replication (primed by a single primer) Discontinuous replication (requiring multiple primers) 3’ 3’ 5’ RNA Primer 5’ 3’ 5’ Okazaki fragments ‘Leading’ and ‘Lagging’ strands during replication Leading strand 3’ Lagging strand 3’ 3’ 5’ RNA Primer 5’ Direction of unwinding Continuous replication (primed by a single primer) Discontinuous replication (requiring multiple primers) 5’ 3’ 5’ 3’ 5’ Okazaki fragments 15 RNA Primer RNA Primer RNA Primer RNA Primer 11/20/24 Ø Summary: Features of DNA Replication (1) DNA replication is semiconservative – Each strand of the template DNA is being copied (2) DNA replication is semi-discontinuous: – The Leading strand copies continuously in the direction of unwinding i.e. a single long molecule – The Lagging strand copies in segments (= Okazaki fragments) which must eventually be joined up! 5′ DNA pol III Primer Many proteins are involved in DNA Replication 3′ Parental DNA 5′ 3′ Primase 5′ Lagging strand Single-strand binding proteins 3′ Lagging strand Overall directions of replication Leading strand 3′ 5′ Helicase 3 1 5 DNA pol III Leading strand template Leading strand Lagging strand Leading strand 3 5′ ′ Lagging strand template DNA pol I 2 DNA ligase 3 ′ 5′ 4 Overview Origin of replication Table 16.1a © 2018 Pearson Education Ltd. 16 11/20/24 © 2018 Pearson Education Ltd. Table 16.1 SUMMARY: Replication Terminology Origin of Replication: The region of a chromosome at which DNA replication begins. In E. coli the origin of replications is known as oriC Primase: the enzyme that synthesizes the RNA primer Primer: DNA Polymerases can only extend a strand, so all DNA synthesis must begin with a Primer which is base-paired to the template Leading strand: the DNA strand that is made continuously (without interruption) Lagging strand: the DNA strand that is made discontinuously (interrupted) not each short newly synthesized strand begins with an RNA primer Okazaki Fragment: the short newly synthesized strands of RNA:DNA on the lagging strand are called Okazaki fragments (after the Japanese scientist who discovered them) Lecture 29 Information Flow in the Cell: RNA and Transcription Ø The Eukaryotic cell: nucleus and cytoplasm Genes code for proteins; and in eukaryotes nucleus cytoplasm genes are located in the nucleus But all proteins are synthesized in the cytoplasm What is the nature of the information that moves from the nucleus to the cytoplasm? Information flow: what is it that moves to the cytoplasm? 5’ 3’ ~ 1,000 bp DNA 3’ 5’ (Located in the Nucleus) ? What is the coding intermediate? (Produced in the Cytoplasm) 1 11/19/24 Ø Chemical nature of the intermediate that travels from the nucleus to cytoplasm A likely candidate = RNA Circumstantial evidence: 1. DNA is located in the nucleus (in chromosomes) But proteins are made in the cytoplasm 2. RNA is synthesized in the nucleus 3. RNA is transported to the cytoplasm where proteins are synthesized Information flows via mRNA 5’ ~ 1,000 bp DNA 3’ (In Nucleus) (Made in the cytoplasm) 3’ 5’ The intermediate = messenger RNA ? (mRNA) Ø Overview: Information flow in the cell Francis Crick’s ‘Central dogma’ of molecular biology: ‘DNA makes RNA makes protein’ DNA makes RNA makes protein this probably evolved first 2 11/19/24 Origins of life (Work by William Martin; Nick Lane and others) (Diagram from: Kevin Mitchell; Free Agents, 2023) Life goes on... Selection for persistence: Things that have the property of tending to persist, persist Things that don’t, don’t Things that out-persist other things come to predominate, especially if that persistence is achieved through replication The RNA World Hypothesis 3 11/19/24 The invention of genetic material (Kevin Mitchell; Free Agents, 2023) RNA RNA DNA mutation proteins mutation DNA Disrupted protein function Less robust persistence/reproduction Negative selection RNA X DNA proteins * * * * proteins * Improved/novel protein function More robust persistence/reproduction Positive selection Structure and properties of RNA 4 11/19/24 Ø Structure of RNA RNAisapolymerwithasugar-phosphatebackbone Bases(4kinds): Purines: Adenine, Guanine Pyrimidines: Uracil, Cytosine It is usually a single-stranded molecule (unlike DNA) But intramolecular base-pairing (via hydrogen bonds) may give rise to double-stranded regions RNA is a single-stranded 5’ polymer § Sugar-phosphate backbone One end has an exposed 5’ phosphate The other end an exposed 3’ hydroxyl 3’ RNA is made of nucleotide monomers Nucleotide = sugar, phosphate, base Phosphate Sugar 3’ 5’ Base 5 11/19/24 The key biochemical differences between RNA and DNA 1. The sugar: Ribose in RNA 1’ vs 2-deoxyribose in DNA 2’ 2’ 1’ 2- 2. Base difference: Uracil in RNA vs Thymine in DNA But Uracil (like Thymine) can ‘base pair’ to Adenine 6 11/19/24 Different types of RNA 1. Messenger RNA (mRNA) This is the RNA transcribed from ‘protein-coding’ genes mRNAs encode the information for specific proteins: Eukaryotic polyA tail UTR = Untranslated region Low abundance (< 10% of all types of RNA in a cell) Short half-life (i.e. subject to rapid turnover: minutes to hours) 2. Ribosomal RNA (rRNA) Is a structural & functional component of Ribosomes The most abundant RNA in a cell (>90% of total RNA) rRNA is very stable rRNA is a non-coding RNA rRNAs associate with ribosomal proteins in the large and small subunits of the ribosome Ribosome 7 11/19/24 3. Transfer RNA (tRNA) Very small - less than 100 bases long A non-coding RNA ‘Clover-leaf’ secondary structure due to internal (intramolecular) base-pairing Plays a key role in Translation (protein synthesis) Very abundant and very stable 4. ‘Non-coding’ regulatory RNAs a. RNAs involved in the regulation of gene expression MicroRNA (miRNA) Short interfering RNA (siRNA) Long non-coding RNA (lncRNA): 200 - 1000s bases b. RNAs involved in mRNA processing (splicin