Psychology 410: Sensory Processes & Perception Lecture Notes PDF
Document Details
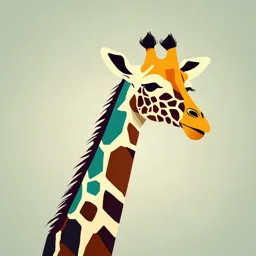
Uploaded by CooperativeHeliotrope1657
University of Tennessee, Knoxville
Daniela Corbetta, Ph.D.
Tags
Related
- Molecular and Cellular Physiology of Neurons (2nd Edition) PDF
- 6th Lecture PDF - Synaptic Transmission & Neurophysiology
- Fondements neurophysiologiques et neuropsychologiques (2020-2021) - PDF
- APK3110 Chapter 7: The Nervous System PDF
- PHY261 Electrical Properties of Multipolar Neuron Lecture Notes PDF
- Neurophysiology of Nerve Impulses PDF
Summary
These lecture notes cover Week 2 of Psychology 410: Sensory Processes & Perception, focusing on the physiological approach to understanding sensory processes. The notes detail fundamental concepts like neurophysiology, electrical signaling in neurons, and measuring brain activity, along with visual elements such as diagrams of neuronal structures.
Full Transcript
WEEK 2: Lecture outlines Reading: Goldstein Chapter 2 THE PHYSIOLOGICAL APPROACH 1. Foundations of neurophysiology - The neurons/receptors and their components/morphology - Neuron and nerves - terminology FUNDAMENTALS OF NEURAL COMMUNICATION AND PROCESSING 1. Electrical signals in neurons - Ions - P...
WEEK 2: Lecture outlines Reading: Goldstein Chapter 2 THE PHYSIOLOGICAL APPROACH 1. Foundations of neurophysiology - The neurons/receptors and their components/morphology - Neuron and nerves - terminology FUNDAMENTALS OF NEURAL COMMUNICATION AND PROCESSING 1. Electrical signals in neurons - Ions - Permeability in neurons membranes - Action potentials - Ion pumps and ion channels 2. Recording electrical signals in neurons - Phases of neural activation - Depolarization - Repolarization - Hyperpolarization - Refractory period 3. Properties of action potentials 4. Transmission from neuron to neuron MEASURING BRAIN ACTIVITY 1. The cerebral cortex - Lobes and modular organization - Primary receiving areas 2. Measuring activity in the brain - ElectroEncephaloGram (EEG) - Evoked potentials (EPs) - Positron Emission Tomography (PET) - Functional Magnetic Resonance Imaging (fMRI) - Functional Near-Infrared Spectroscopy (NIRS) - Connectomics LIGHT: THE STIMULUS FOR VISION 1. Seeing the light (physical energy) - The electromagnetic spectrum - Wavelength visible to the human eye - Short and long wavelengths (range in nanometers) - How animals see the world THE VISUAL SYSTEM AND ITS RECEPTORS 1. A dynamic system 2. Major connections in the visual system 3. Anatomy of the eye 4. The eye focusing power - Normal vision - Visual accommodation 5. What makes us see the light? 6. How is the light captured 7. Major types of cones and rods 8. Four kinds of visual receptors and their light absorption characteristics 9. Distribution of cones across the retina 10. Major connections in the visual system of the brain - Visual hemifields PSYC410_CH02-WK2_1_PhysiolofPerception ====== The presented content includes two slides from a PowerPoint presentation focused on the subject of "Psychology 410: Sensory Processes & Perception." The slides are formatted in a professional manner, likely intended for an academic audience. The first slide, labeled as slide 7, contains a brief copyright notice from the instructor, Daniela Corbetta, Ph.D. This notice reinforces the importance of intellectual property rights, indicating that the material is intended solely for the use of registered students and cannot be distributed or sold without authorization. The background of the slide is plain, emphasizing the text. The second slide, labeled as slide 8, introduces "UNIT 2" with the title "The Physiological Approach." This slide features a visually striking background, possibly depicting a top-down view of a forest or trees, which is relevant to psychological topics regarding nature and sensory experiences. Below the title, there is a courteous reminder to students to turn off their cell phones or set them to airplane mode, suggesting an expectation of focus and attention during the presentation. Both slides contain consistent formatting, utilizing the same header for the course title and maintaining a formal tone throughout. ``` Psychology 410 Sensory Processes & Perception UNIT 2 The Physiological Approach ``` ====== In the first section displayed, titled "The Physiological Approach," a flowchart illustrates the relationship between a stimulus and the subsequent physiological and perceptual responses. At the top, "stimulus" is referenced as the initiating factor. Following this, a pathway leads to "physiological response," suggesting a biological reaction to the stimulus. This response then connects to "perceptual response," indicating how the physiological reaction is interpreted at a cognitive level. Accompanying this text is a circular diagram that represents various cognitive processes, including "Knowledge," "Processing," "Perception," "Recognition," and "Action," indicating a cycle of interaction between stimuli and processed responses. The diagram also includes terms like "Environmental stimulus" and "Attended stimulus," highlighting the focus of perception toward certain stimuli. The second section, titled "The components of a vertebrate motor neuron," features an illustration of a neuron, clearly labeled to show its various parts. The diagram includes "Dendrite," "Nucleus," "Soma," "Axon hillock," "Axon," "Myelin sheath," "Presynaptic terminal," and "Muscle fiber." Each labeled component is color-coded and distinctly visualized to indicate the structure of a motor neuron which plays a crucial role in transmitting signals within the nervous system. ``` The Physiological Approach stimulus physiological response perceptual response The components of a vertebrate motor neuron Dendrite Nucleus Soma Axon hillock Axon Myelin sheath Presynaptic terminal Muscle fiber ``` ====== The visual consists of two main sections, each featuring detailed diagrams relevant to the study of nerve cells. The first section illustrates a neuron and its components, specifically highlighting the relationship between a touch receptor and its corresponding nerve fiber. It depicts the process of transduction, which involves the conversion of a stimulus into an electrical signal within the nerve fiber. Key parts included are the touch receptor, nerve fiber, dendrites, the cell body, and an axon. Arrows indicate the flow of information, while questions prompt further consideration of where transduction and processing occur in the overall function of the neuron. The second section focuses on the morphologies of various nerve cells. Multiple types of neurons are illustrated, showing the distinct features and structures that characterize different nerve cell types. This variety emphasizes the complexity and specialization of neurons within the nervous system, showcasing their various forms and adaptations suited for specific functions. ``` Receptor and Neuron Where does transduction occur? Where does processing occur? Figure 2.18 p.33 Nerve cell morphologies Figure 1.2. Examples of different types of nerve cells. 1 a. Atypical axon; b. Cerebellar Purkinje cell; c. Bipolar neuron; d. Motor neuron; e. Spine-associated cell. ``` ====== The content consists of two distinct slides from a presentation likely related to psychology, specifically focusing on the fundamentals of neuronal signaling. The first slide presents a definition of key terminologies associated with neurons. It explains the structure and function of a neuron, defining terms such as "neuron," "axon," "nerve," "electrical signal," and "propagation." Each term is succinctly described, providing clarity on the anatomy and physiology of nerve cells. The slide uses bullet points to streamline the information for easier reading, indicating that it is educational material intended for students or individuals learning about sensory processes and perception. The second slide introduces the topic of the week (Week 2) and indicates that the focus will be on the "Fundamentals of Electrical Signaling in Neurons." This presents the audience with the overarching theme of the lecture or discussion. The background consists of an overhead view of what appears to be trees or foliage, possibly symbolizing natural processes or connections, which complements the theme of signaling in the nervous system. The overall layout of both slides employs a clean, academic format, with titles, bullet points, and accompanying visuals to enhance understanding. ``` Defining Terminology A neuron (= nerve cell) has cell body, axon, synapses, dendrites The axon = nerve fiber A nerve → many nerve fibers/axons’ bundle projecting to parts of brain or body (e.g. optic nerve, nerves to muscles) Electrical signal = nerve impulse = action potential Propagation: When electrical signal travels down axons and from neurons to neurons Psychology 410 Sensory Processes & Perception WEEK 2 Fundamentals of Electrical Signaling in Neurons Please note: Daniela Corbetta, Ph.D., retains copyright of this licensed PowerPoint and of all of her instructional materials. This recording is strictly intended for a collaborative learning experience and may not be shared, duplicated, or disseminated in any way, without the express written consent of the author. Any unauthorized use of this PowerPoint presentation or its content may be subject to the user for criminal or civil actions or a violation of the Resource Policy and Procedures. ``` ====== The content primarily focuses on the topic of electrical signals in neurons, highlighting the significance of ions in neural signaling. In the upper section, key points emphasize that electrical signaling occurs in a "wet" environment, designated as cerebrospinal fluid, where neurons are immersed in a solution rich in ions. These ions, defined as molecules carrying an electrical charge that can gain or lose electrons, are crucial for the functionalities of neurons. The lower section elaborates on the neuron's membrane and its selective permeability during different states, particularly in its resting state where no action is propagated. It notes that outside the axon fiber, the solution is rich in sodium (Na⁺) ions, and it provides a depiction of the neuron's electrical dynamics with respect to the concentration of ions, particularly sodium and potassium (K⁺). The active state of the neuron is illustrated with a voltage range, with values labeled as +40 mV and -70 mV (millivolts). Overall, the content serves as an educational overview of how neurons communicate through electrical signals facilitated by ionic movement across their membranes. ``` Electrical Signals in Neurons - Electrical signaling occurs in “wet” environment (cerebrospinal fluid) - Neurons bath in a solution rich in ions - Ions: molecules carrying an electrical charge (electrons attach to them) - Ions gain or lose electrons The membrane of a neuron can change its permeability selectively Resting state (no propagation/no activity) Outside of axon fiber, solution rich in ions positively charged with sodium (Na⁺) Electrical signal occurs when ions flow across the neural membrane (active state) Inside of axon fiber, solution rich in ions positively charged with potassium (K⁺) +40 mV -70 mV (millivolts) ``` ====== The visual includes two distinct sections focusing on the role of ion channels and ion pumps in neurons. The first section illustrates a diagram that describes how ion exchange takes place during the active state of a neuron. It explains the movement of ions across the neuron's membrane, showing sodium (Na⁺) ions moving from outside the neuron to the inside through specific pathways, and potassium (K⁺) ions moving from inside the neuron to outside. The diagram labels the states of the ions involved in this exchange and indicates the direction of ion flow using colored arrows and numbers. The second section presents a more straightforward schematic representation of ion pumps and ion channels. It provides a comparative illustration, highlighting that while ion pumps move ions against their concentration gradient, ion channels allow ions to diffuse according to their gradients. There are arrows illustrating the inward movement of sodium ions through the ion channels, while the ion pump is depicted actively transporting ions. This section emphasizes how these mechanisms generate cellular electricity, essential for neuronal action potentials. The background of each section appears to be a plain light color, allowing the diagrams and text to stand out clearly, aiding comprehension of the complex biological processes. ``` Ion channels in the membrane of a neuron: a 3-step exchange Active state (propagation occurs) GREEN: Outside sodium ions (Na⁺) flow inside the axon fiber (diffuse through channels) 1 2 RED: Inside potassium ions (K⁺) flow outside axon fiber Ion pumps and ion channels Figure 2.2 Ion pumps and ion channels are responsible for ionic movements across neuronal membranes. Pumps can be considered active transports against their chemical gradients. Channels allow for passive diffusion, allowing selected ions to move via diffusion, down their electrochemical gradients. Pumps and channels work against each other, in doing so, they generate cellular electricity (action potential) ``` ====== The content illustrates two main sections focused on the electrical signaling process in neurons, including methods for recording these signals and the physiological changes occurring during action potentials. In the first section, the left side displays a diagram outlining methods to record electrical signals from neurons. It shows an axon connected to a recording apparatus via an intracellular microelectrode and an amplifier connected to a computer. The right side appears to include a visual element, perhaps depicting a natural scene with branches against a background of light, possibly symbolizing the complexity of neuronal networks. The second section comprises a series of illustrations depicting the action potential phases: 1. "Potential at rest," which describes the resting membrane potential of a neuron. 2. "Depolarization," illustrating the influx of sodium ions (Na+) into the axon, which causes the membrane potential to become less negative. 3. "Repolarization," showing potassium ions (K+) exiting the axon, working to return the membrane potential towards its resting state. 4. Finally, there is a note on the role of ion pumps in restoring sodium and potassium gradients across the membrane and reaching the resting potential after an action potential. The diagrams systematically progress to illustrate how the action potential changes over time, marked by specific graphical representations showing voltage changes corresponding to these phases. ``` Methods for recording electrical signals in neuron Potential at rest Depolarization Repolarization Ion pump restores densities of sodium/potassium in and out of the membrane to reach resting potential refractory period ``` ====== The visual consists of two main sections, each depicting concepts related to nerve impulses and action potentials. The first section (labelled as "21") illustrates the process of a nerve impulse traveling, displaying different phases of an action potential. It features a diagram with a cylindrical representation likely signifying an axon, along with labeled parts: - Potential at rest: This indicates the resting membrane potential, represented as a static state before an impulse occurs. - Depolarization (→): This phase is illustrated with the influx of sodium ions (Na+), which leads to a change in the membrane potential. - Repolarization (→): This shows potassium ions (K+) leaving the neuron, marking the restoration of the membrane potential. - Ion pump restores densities of sodium/potassium in and out of the membrane to reach resting potential → refractory period: This part highlights the action of ion pumps in resetting ion concentrations after an action potential, briefly blocking further impulses during the refractory period. Each phase is supported by corresponding graphs on the right side, depicting the change in membrane potential (y-axis) over time (x-axis). The shape of these graphs illustrates the rise and fall of the action potentials during the processes depicted in the diagram. The second section (labelled as "22") is simpler and consists of a title and instructions for a demonstration about phases of action potentials. It emphasizes the educational aspect, suggesting that the audience will engage with the material further through a demo. ``` Nerve impulse travelling Potential at rest Depolarization → Repolarization → Ion pump restores densities of sodium/potassium in and out of the membrane to reach resting potential → refractory period Illustrate with DEMO #6 Phases of action potentials ``` ====== The content depicts a comprehensive overview of electrical signals in neurons, illustrating fundamental principles relevant to action potentials. The first section outlines how electrical activity in neurons operates on the all-or-none principle. There are visual elements showing a neuron, its components, and a graph representing action potentials. The neuron graphic highlights key features such as the soma, axon, and dendrites. The graph illustrates the relationship between depolarization and action potentials, with markings that differentiate between various states of the neuron: resting potential, depolarization (induced by the influx of sodium ions), and hyperpolarization. It states that the potential at rest is less than 70 mV, indicating a polarized state. A reference to the action potentials indicates that they lead to a shift towards depolarization, appearing as spikes on the timeline. The lower section, titled "All-or-none principle," features separate graphs for depolarization and hyperpolarization. The graphs depict a change in membrane potential, emphasizing that a threshold must be surpassed for an action potential to occur and that hyperpolarization can inhibit neural firing. The visuals effectively summarize the dynamics of nerve signal propagation and the essential nature of excitatory versus inhibitory responses. ``` Recording Electrical Signals in Neurons All or none response Action potentials => depolarization potential in membrane > potential at rest (=diffusion of Na+ through the cell membrane) All-or-none principle (a) Change in membrane potential Depolarization (Excitatory) (b) Change in membrane potential (c) Change in membrane potential Hyperpolarization (Inhibitory) Level of depolarization needed to trigger an action potential Level to trigger action potential ``` ====== The content presents information about action potentials, their sequence of activation, and their characteristics. The visuals include diagrams and graphs that illustrate the process of action potentials in neurons. In the upper section, titled "Sequence of activation," there's a diagram that likely represents the cellular processes during action potential generation. It showcases the permeability of sodium (Na⁺) and potassium (K⁺) ions, indicating how the distribution of these ions influences the action potential. The associated text states that the typical duration of an action potential in nerves is around 1 millisecond, while in skeletal muscle nerves it can last between 2 to 5 milliseconds. The lower section, titled "Properties of action potentials (AP)," outlines key features of action potentials. It explains that once initiated, action potentials propagate along the axon over long distances. The amplitude remains constant, regardless of variations in stimulus intensity. Additionally, the text notes that variations in stimulus intensity result in differences in firing frequencies, which leads to higher firing rates with increased stimulus intensities. However, there is a limit to the firing rate, cited as 500-800 pulses per second, linked to the refractory period—the time needed for the ion pumps to reset before a new action potential can occur. Overall, the content is educational and appears to be aimed at an audience studying neuroscience or physiology, focusing on neurophysiological mechanisms. ``` Sequence of activation 1 msec duration of action potential in typical nerve In skeletal muscle nerves, an action potential can last 2-5 msec Properties of action potentials (AP) - Once triggered, APs travel all the way down the axon → propagation over long distances - Amplitude of APs is always identical (+40mV) throughout propagation, even if intensity of stimulus changes - Variations in stimulus intensities → variations in firing frequencies → greater stimulus intensity = increased firing rate BUT - Limit to the firing rate: 500-800 pulses per second. Why? - APs last 1msec (activity of ion pump) before nerve cell can fire again → refractory period ``` ====== The content consists of two main sections displayed in a structured format. The first section illustrates a graph and discusses the action potential in the context of neuronal physiology. The x-axis of the graph is labeled "Time (ms)" and ranges from 0 to 5 milliseconds, while the y-axis shows "Voltage (mV)", ranging from -70 to +40 millivolts. Key features of the graph include annotations indicating significant points such as "Resting state," "Threshold," "Action potential," "Repolarization," "Refractory period," "Stimulus," and "Failed initiations." The graph highlights the all-or-none principle of action potential triggering. The second section provides an illustration geared towards explaining the process of transmitting information across a synaptic gap in the nervous system. It includes sequentially labeled diagrams or steps (a to c). These depict components such as receptors and neurotransmitter molecules, indicating how a stimulus leads to neurotransmitter release, binding to receptors, and the resulting signal transmission between neurons. Each part of this educational content focuses on understanding the dynamics of nerve signal transmission, emphasizing both the electrical and chemical components involved. ``` http://en.wikipedia.org/wiki/Refractory_period_%28physiology%29 AP triggering: All-or-none principle Illustrated with DEMO Transmitting Information Across a Gap ``` ====== The content displays two slides focused on the transmission process of signals between neurons. Each slide contains both text and illustrations that visualize the concept of synaptic transmission. The first slide outlines the fundamental aspects of synaptic transmission. It emphasizes that neurons communicate through a synapse, which is characterized as a small gap where axon terminals of one neuron connect to other neurons, dendrites, or even organs. The illustration depicts synapses and indicates that the process of transmission is chemical in nature. The second slide expands on the mechanisms involved in synaptic transmission. It discusses postsynaptic receptors and neurotransmitter molecules, explaining that these receptors come in various shapes. Importantly, it highlights that a voltage change in the postsynaptic neuron occurs only when a neurotransmitter matches the receptor's shape, leading to either excitation (increased firing rate) or inhibition (decreased firing rate). This slide visually contrasts the presynaptic neuron (the sending neuron) with the postsynaptic neuron (the receiving neuron) while using arrows to indicate the flow of information. Here is the extracted text: ``` Transmission From Neuron to Neuron Occurs through synaptic transmission Synapse → small space between neurons where the axon terminals connect to new cell bodies, dendrites, or organs Transmission is a chemical process (Fornito et al. (2012) Neuroanatomy, 99, 1001-1007. Elsevier) Transmission From Neuron to Neuron Postsynaptic receptors & neurotransmitter molecules exist in variety of shapes Voltage change in postsynaptic neuron occurs only when receptor site matches shape of neurotransmitter Match can create excitation (increase rate of firing) or inhibition (decrease rate of firing) → both are needed for processing ``` ====== The content consists of two main sections. The first section is a title slide for a psychology course, specifically focusing on sensory processes and perception. It prominently displays the week number ("WEEK 2") and the topic, "Measuring Brain Activity." The slide has a dark background featuring a top-down view of a forested area, which adds a naturalistic element that contrasts with the scientific topic. Below the title, there is a note addressing the copyright information, including the name of the instructor, who is likely responsible for the content. In the second section, a diagram illustrates the cerebral cortex and its modular organization. This visual aids in understanding how different areas of the cortex serve specific functions related to sensory processing. The diagram includes labeled areas such as the parietal lobe (which contains the somatosensory cortex), occipital lobe (visual cortex), temporal lobe (auditory cortex), and frontal lobe. The primary receiving areas are noted, as well as descriptions of various brain functions, emphasizing that the cortex is approximately 2mm thick and contains around 100 billion densely interconnected neurons. The spinal cord is also depicted, showing its connection to the brain. The overall design balances visual appeal with educational content, effectively combining imagery and diagrammatic representation to facilitate comprehension of the brain's structure and its functional assignments. ``` Psychology 410 Sensory Processes & Perception WEEK 2 Measuring Brain Activity Please note: Daniela C. Cardella, PhD., retains copyright of this recorded PowerPoint and all of her instructional materials. This recording study materials is a derivative of her instructional materials and may not be reproduced or distributed in any form without her express written permission. Figure 1.24, page 26 The Cerebral Cortex and its Modular Organization Cortex is about 2mm thick and contains 100 billion neurons richly interconnected Frontal lobe Parietal lobe (somatosensory cortex) Occipital lobe (visual cortex) Temporal lobe (auditory cortex) Olfaction Olfaction Central fissure Spinal cord Modular organization = specific functions are served by specific areas of the cortex ``` ====== The visual content showcases two separate diagrams related to human brain anatomy, specifically focusing on the cerebral cortex and its functional subdivisions. The first diagram illustrates various sensory cortices located in the cerebral cortex. Each labeled section highlights a specific sensory function: - Vestibular cortex which is associated with balance. - Somatosensory cortex responsible for touch. - Gustatory cortex linked to the sense of taste. - Visual cortex pertaining to sight. - Olfactory cortex for smell. - Auditory cortex associated with hearing. Additionally, there is a note indicating that smell and taste are collectively referred to as chemical senses, emphasizing their interconnected nature in sensory perception. The second diagram presents an overview of the major subdivisions of the human cerebral cortex. It illustrates a lateral view of the brain with color-coded sections denoting different areas, though the specific subdivisions themselves are not identified in the diagram. This serves to provide a basic understanding of the organizational structure of the cerebral cortex. Overall, both diagrams serve educational purposes, providing valuable insight into the functional anatomy of the brain. ``` 33 Vestibular cortex (balance) Somatosensory cortex (touch) Gustatory cortex (taste) Visual cortex (sight) Olfactory cortex (smell) Auditory cortex (hearing) Smell and taste also called chemical senses 34 Some major subdivisions of the Human cerebral cortex ``` ====== The visual consists of two sections, each presenting topics related to EEG (Electroencephalography) and different methods for measuring brain activity. In the first section, titled "Measuring activity in the brain (1)," the content details various techniques for assessing brain function. Key points include: - Evoked potentials (EPs), EEG: This technique involves surface electrodes that record changes in voltage from a large population of neurons, approximately a thousand. - PET (Positron Emission Tomography): It highlights that a small amount of radioactive tracer is injected into the subject. PET scans track changes in blood flow revealed by the tracer while the subject engages in a perceptual task. - fMRI (functional Magnetic Resonance Imaging): This method is described as measuring blood flow changes, emphasizing that there is no need for a tracer. Instead, it utilizes a magnetic field to detect ferrous molecules in hemoglobin. This section is attributed to Daniela Corbetta and marked with the number 35, likely indicating a slide number or reference within a larger presentation. In the second section, labeled with the number 36, the focus shifts to EEG, specifically the “ElectroCap 10-20 system.” The visuals include two images of children wearing EEG caps, which are specifically designed for positioning electrodes according to the 10-20 system. The caps appear to be white and yellow, and the children are depicted with varied expressions, likely during a study or testing scenario. The background seems neutral, focusing on the subjects and their headgear, which is crucial for the EEG process. ``` Measuring activity in the brain (1) Evoked potentials (EPs), EEG – Surface electrodes record change in voltage over a large group of neurons (~1000) PET (Positron Emission Tomography) – Low dose of radioactive tracer is injected to the person. PET tracks changes in blood flow revealed by the tracer as person performs a perceptual task fMRI (functional Magnetic Resonance Imaging) – Measures changes in blood flow as well. No need of tracer. Uses magnetic field to capture ferrous molecules in hemoglobin EEG ElectroCap 10-20 system ``` ====== The content consists of two slides, likely from a presentation regarding EEG (electroencephalography) systems. The first slide features two images. On the left is a woman wearing a headgear designed for EEG recording, with a mesh covering and electrodes positioned on her scalp. She appears engaged, possibly ready for a recording session, dressed in a dark top against a neutral backdrop. On the right, a child is shown wearing a similar EEG cap, looking straight ahead with a calm expression. The setting appears clinical, suggesting that both subjects are involved in a study or assessment utilizing the EEG technology. The second slide shows a setup for EEG data collection. In the foreground, a computer monitor displays waveforms typical of EEG output, indicating brain activity. Below the monitor, a keyboard rests on a wooden surface, while various electronic devices are seen in the background. The equipment suggests a research or clinical environment, equipped for detailed analysis of brain patterns. The presence of a person, whose hand is visible reaching towards the monitor, indicates active monitoring or data collection. The surrounding equipment implies a sophisticated setup potentially used for various neurological assessments. ``` EEG 128 channel recording system Figure 2.2 p.15 ``` ====== The content depicts a presentation related to brain imaging methods, specifically focusing on the subtraction method used in neuroimaging. The visual part is divided into two sections, reflecting different angles of information concerning brain activity. On the left side, there are three brain diagrams labeled sequentially as (a), (b), and (c). These illustrations represent different conditions of brain activity. - Diagram (a) is labeled "Control," indicating a baseline state without any stimulation. - Diagram (b) is labeled "Stimulation," where some form of stimulus is introduced. - Diagram (c) is labeled "Activity due to stimulation," showing the active responses from the brain because of the introduced stimulus. The stimulated areas appear marked or highlighted, contrasting with the control conditions. Below this graphic, the content shifts focus to methods of measuring brain activity, presented in bulleted format: - The first bullet point outlines fNIRS (Functional Near-Infrared Spectroscopy), a technique for measuring brain activity linked to hemodynamic responses. It provides a brief explanation of how light is shone through the skull and notes that hemoglobin absorbs light differently than other tissues. - The second bullet point introduces connectomics, specifically diffusion MRI tractography. It describes a "connectome" as a comprehensive map of neural connections within the brain, likening it to a wiring diagram. The text is clear and well-structured, providing an overview of these neuroimaging techniques. ``` Brain imaging, subtraction method (a) Control (b) Stimulation (c) Activity due to stimulation Measuring activity in the brain (2) fNIRS (Functional Near-Infrared Spectroscopy) – Measures brain activity through hemodynamic (blood flow) responses associated with neuron behavior. Light is shined through the scull. Hemoglobin has high light absorption properties, while other tissues have low light absorption properties. Connectomics (diffusion MRI tractography) – A connectome is a comprehensive map of neural connections in the brain, and may be thought of as its “wiring diagram” ``` ====== The content comprises two slides, each presenting distinct scientific concepts related to neuroscience. The first slide is labeled "fNIRS," which stands for functional Near-Infrared Spectroscopy, a technology used to monitor brain activity. In this visual, two individuals, identified as children, are interacting with a table filled with colorful pieces, likely a puzzle or a game. Both individuals are wearing specialized headgear that includes sensors connected by wires to a larger unit situated behind them. This setup suggests that they may be participating in an activity while their brain activity is being monitored by the fNIRS system. The second slide is titled "Connectomes," indicating a focus on the interconnected networks of neurons in the brain. It contains two colorful illustrations depicting intricate neural pathways within the brain. The visuals use bright colors such as pink, blue, green, and yellow, exhibiting the complex structure of the connectome, which represents the connections between different brain areas. The illustrations are likely designed to showcase the complexity and beauty of brain connectivity in a visually stimulating way. ``` fNIRS Connectomes ``` PSYC410_CH02-WK2_2_VisualSystem ====== The content presents a PowerPoint presentation designed for a course titled "Psychology 410: Sensory Processes & Perception." The first slide, serving as a cover page, features a simple, white background with text stating the course title. Below that, there is a note about copyright, highlighting that the materials are owned by Daniela Corbetta, Ph.D. It emphasizes that the content is intended solely for enrolled students and warns against unauthorized distribution or sharing of the materials electronically. The second slide, with a darkened visual of dense foliage or treetops, includes the course title again, along with the phrase "WEEK 2: Light: The Stimulus for Vision." This suggests that the week's focus will be on the topic of light in the context of sensory perception. The overall aesthetic combines a straightforward slideshow format with a focus on educational content, specifically within the realm of psychology. ``` Psychology 410 Sensory Processes & Perception WEEK 2 Light: The Stimulus for Vision ``` ====== The content presents an overview of the mechanism of seeing, emphasizing the role of light as both a stimulus and a critical component of the visual system. The first section highlights the relationship between light and the visual mechanism, indicating that seeing requires both the presence of light and the ability of the visual system to respond to it. The second section delves into the electromagnetic spectrum, illustrating the continuum of electromagnetic energy. It notably specifies that the wavelengths of visible light for humans range from 400 to 700 nanometers. This part includes a graphic that categorizes different wavelengths, showing the spectrum from gamma rays, which are on the short wavelength end, through to infrared rays and alternating current circuits at the long wavelengths. Additionally, the graphic indicates harmful UV radiation from the sun, namely UVB and UVA. The description stresses that electric charges radiate as waves and provides a brief explanation about the applicability of wavelengths. The visual elements such as the gradient representing different wavelengths are color-coded, providing an intuitive understanding of how these wavelengths are perceived, ranging from purple (short wavelengths) through green and yellow, to red (long wavelengths). ``` Seeing the light Seeing involves a stimulus (light) AND Mechanism that reacts to light (visual system) , 2005 to 2021 Figure 2.2, page 23 Electromagnetic spectrum → continuum of electromagnetic energy Humans visible light has wavelengths that range from 400 to 700 nanometers 400 500 600 700 Short wavelengths Long wavelengths Ultraviolet Visible Infrared Gamma rays X-rays Ultraviolet Radar FM TV AM AC circuits: alternating current electricity Electric charges radiate as waves Length of waves indicate distance between peaks in nanometers (nm) UVB/UVA: Sun damaging UV Gamma rays: form of hazardous radiation, decay of atomic nuclei ``` ====== The content consists of two slides likely from a presentation focused on the differences in visual perception among dogs, humans, and cats. The first slide is titled "How the spectrum looks to dogs and people" and includes a visual illustration comparing the wavelength perception of dogs and humans. There is a gradient color scale showing different wavelengths, with the dog's view represented in tones of yellow, dark blue, and other muted colors, indicating a restricted color range compared to humans, who see a broader spectrum that includes vibrant colors. The dog’s view section has a more limited representation of the color spectrum, suggesting they perceive less color variety than humans. Below the color scales, there are photographs featuring flowers arranged in different colors, with side-by-side comparisons to provide a visual representation of how each species would perceive the same scene. The second slide is titled "How Cats See the World" and discusses cat vision, highlighting that cats see colors in a more muted way but possess a wider visual field of 200 degrees compared to the 180 degrees of humans. This section is divided into images illustrating various aspects of cat vision. These images typically reflect scenes with distorted clarity: "Great night vision" is described alongside a darkened image to represent their superior night vision capabilities. It also includes a statement defining the cat's "Poor distance vision" with accompanying imagery that appears fuzzy, indicating the limitations in their ability to focus on distant objects, quantified as 20/100-200 vision. The overall tone and content suggest an educational approach to understanding animal vision and provide visual aids to enhance the comprehension of differences in color perception and field of vision between humans, dogs, and cats. ``` How the spectrum looks to dogs and people The Dog's View The Human's View "We perceive what is out there as filtered through the properties of the visual system" (Goldstein, 2002, p. 36) How Cats See the World See colors more attenuated but have 200° visual field as opposed to 180° in humans Great night vision Poor distance vision 20/100-200 ``` ====== The visual presentation consists of two primary sections: one focusing on the spectrum of light and its wavelengths, and the other on a psychology course titled "The Visual System." In the first section, a colorful spectrum of light is illustrated, indicating different wavelengths measured in nanometers (nm) ranging from ultraviolet on the left (below 400 nm) through the visible spectrum (approximately 400 to 700 nm) to infrared on the right (above 700 nm). The visible light section is centrally located and is highlighted to indicate its significance to human vision. Additionally, there are annotations pointing out the specific visibility of light for various creatures, such as bees and birds for ultraviolet and certain species of snakes for infrared. Links to YouTube videos are provided, suggesting further exploration of how animals perceive the world through the variations in light wavelengths. The second section presents a title slide for a psychology course labeled "Psychology 410," detailing the topic of sensory processes and perception focusing specifically on the visual system during week two of the course. The background is a scenic aerial view of a dense forest, which reinforces the theme of nature and visual perception. Overall, the content emphasizes the importance of understanding the visual spectrum not only for humans but for various animal species, and it establishes a foundation for studying sensory processes in a psychological context. ``` A beam of light separated into its Wavelengths "We perceive what is out there as filtered through the properties of the visual system" (Goldstein, 2002, p. 36) Visible to many insects (bees) and birds (paridae) Visible light to human eye Visible to some species of snakes Psychology 410 Sensory Processes & Perception WEEK 2 The Visual System ``` ====== The visual representation consists of two main sections dedicated to understanding the visual system and its connections within the brain. The first section outlines key concepts related to the visual system, emphasizing its dynamic nature and the various components involved in the act of seeing. It highlights the role of light-sensitive receptors and neurons, along with the importance of pigment molecules and cognitive processes in interpreting visual stimuli. The second section features an illustration of the brain, detailing the major connections in the visual system. It includes labeled areas such as the eye, optic nerve, and visual cortex, showing how light energy travels from the eye to the brain. Additionally, a visual pathway is demonstrated, indicating the lateral geniculate nucleus and the connections it has with the visual receiving area of the brain, which plays a critical role in processing visual information. Overall, the materials provide an educational overview of how visual perception works, from the initial capture of light by the eyes to the complex neural pathways leading to cognitive interpretation in the brain. ``` The Visual System Dynamic system at work Seeing involves: – Large number of – Light-sensitive receptors – Neurons – Various – Pigment molecules – Combinations of neural activity – Cognitive ability – Knowing/interpreting what we see , 2005 to 2021 Major connections in the visual system of the brain Lateral geniculate nucleus in thalamus Visual receiving area (cortex) Eye – Light energy Optic nerve Superior colliculus Optic chiasm Rentina © Copyright 2005 Daniela Corbetta, visual system adapted from Kosslyn and Koenig 1992 ``` ====== The content consists of two slides detailing aspects of the vertebrate eye, with illustrations and textual explanations about its anatomy and focusing power. The first slide presents a cross-section of a vertebrate eye. Key structures are labeled, including: - Iris: Controls the size of the pupil. - Pupil: The opening allowing light to enter the eye. - Cornea: Contributes to the majority of the eye's focusing power. - Lens: Adjusts shape to help focus light on the retina. - Ciliary muscle: Controls the shape of the lens. - Vitreous humor: The gel-like substance filling the eye. - Macula: The central region of the retina responsible for sharp vision. - Retina (rods and cones): Sensory cells that convert light into neural signals. - Optic nerve: Transmits visual information to the brain. - Blind spot: Area with no photoreceptors, where the optic nerve exits. The second slide focuses on the eye's focusing power, highlighting key points such as: - The cornea accounts for over 80% of the eye's focusing power, while the lens provides the remaining 20%. - It explains how light rays entering the eye from a distance (20 feet) are parallel and in focus, while closer distances result in rays that diverge and require accommodation to maintain focus. - It also mentions different visual conditions: myopia (nearsightedness), hyperopia (farsightedness), and presbyopia (age-related loss of focusing ability). The artwork enhances the descriptions, showing diagrams of the eye's anatomy and how various visual conditions affect focus. ``` Cross-section of the vertebrate eye , 2005 to 2021 The eye focusing power Cornea ➔ 80% of the eye focusing power Lens ➔ remaining 20% 20 feet ➔ light rays enter the eye in parallel < 20 feet, light rays no longer parallel ➔ image out of focus ➔ accommodation Accommodation possible up to near point (point of max accommodation) Presbyopia = product of aging , 2005 to 2021 ``` ====== The content consists of two main sections related to the anatomy of the eye and visual acuity measurement. The first section illustrates various optical scenarios within the eye, displayed through diagrams. There are five labeled illustrations depicting how the eye focuses on an object depending on its distance. 1. The first image demonstrates focusing on a distant object where the focus point lands on the retina. 2. The second image details a moving object, explaining how the focus point moves back. 3. The third image shows a nearby object where the focus point falls in front of the retina, indicating accommodation. 4. The fourth image presents a scenario labeled "Accommodation point reached," where the focus is on the retina. 5. The fifth diagram features corrective lenses that adjust the focus point appropriately. In the second section, the Snellen chart details visual acuity measurement. It provides information on how different lines indicate the clarity of vision: - Line 7 illustrates the standard 20/20 vision. - Line 5 represents a person with 20/40 vision when viewed at a distance of 40 feet. - It mentions that blurred vision reading below line 5 indicates nearsightedness. The right side presents a Snellen chart with letters arranged in decreasing size. The top row starts with an "E" and the letters decrease in size down to the last row. The background is primarily white with clear, concise illustrations in red and black. The visual elements are educational, aimed at conveying the mechanisms of vision and their measurement. ``` Snellen Charts to Measure Acuity Line 7 - 20/20 Line 5 - 20/40 person with normal vision see line at 40 ft Blurry vision below line 5 indicates nearsighted E M F D P T Z F D U E Z D F U M P T Z F D U D E M , 2005 to 2021 ``` ====== The visual content is split into two upper and lower sections, presenting detailed illustrations related to the anatomy and function of the eye, specifically focusing on the receptors involved in vision. In the upper section, there are two diagrams labeled (a) and (b). Diagram (a) illustrates the basic structure of the eye, including the pathway of light as it enters. It features elements like the pupil, cornea, lens, and retina. The diagram highlights the "Direction of light" with an arrow, demonstrating how light reflects off objects and enters the eye, ultimately stimulating the rod and cone receptors in the retina. The presence of labels like "Optic nerve fibers," "Rod," "Cone," and "Pigment epithelium" indicates the different components involved in light perception. In the lower section, the focus shifts to the structural details of rod and cone cells, which are critical for vision. An illustration reviews the segmented structure of these photoreceptor cells, depicting their outer and inner segments, as well as the receptor cell body. This part also includes a microscopic image on the right side, showing the fine details of visual pigment molecules, possibly emphasizing the intricate structure that enables these cells to function effectively in light detection. The overall layout is educational, aiming to provide insight into how light interacts with ocular components and how visual information is processed at the cellular level, enhancing an understanding of both anatomy and physiology of vision. ``` Figure 2.2, page 23 Eye cross-section and how is light captured Light reflects from objects Light reflection stimulates rod and cone receptors in retina (a) (b) Structure of rod and cone Visual pigment molecules Receptor outer segment Receptor inner segment Receptor cell body ``` ====== The content depicts two diagrams related to the structure of the vertebrate retina, highlighting different aspects of retinal anatomy. The first diagram illustrates the arrangement of neurons within the retina. It features a detailed layout of the retinal cell types, including photoreceptors (rods and cones), bipolar cells, and ganglion cells. The diagram emphasizes the pigment epithelium, which is situated at the back of the retina. This layer is noted for containing vital nutrients and enzymes necessary for the proper functioning of visual receptors. Arrows are used to indicate the direction of visual signal processing, with an emphasis on how these cells are interconnected to facilitate visual input. The second diagram presents a cross-sectional view of the retina, showcasing various layers, including the photoreceptors (the outermost layer), bipolar cells, and ganglion cells at different depths. This cross-section provides insight into the cellular composition and organization of the retina, illustrating how these cells interact and contribute to visual processing. This view highlights the intricacy of retinal structure, revealing how these different cell types are arranged in close proximity to each other. Overall, the diagrams serve a complex educational purpose, conveying the intricate relationships within the retina's cellular architecture and the fundamental role each cell plays in vision. ``` The vertebrate retina (diagram of the neurons of the retina) Pigment epithelium Contains vital nutrients and chemicals (enzymes) needed for the functioning of the visual receptors The vertebrate retina (cross-section through the retina) Receptors Bipolar cells Ganglion cells Axons from ganglion cells ``` ====== The content features two slides discussing the properties of rods and cones, which are types of photoreceptor cells located in the retina of the eye. The first slide contains an electron microscope image showing the shapes of rods and cones, with arrows pointing to each type. The slide is titled "Rods and cones varying properties" and includes a caption indicating the labeling of rods and cones. The second slide elaborates on the characteristics of rods and cones in a bullet-point format. The section for rods highlights key features: they are responsible for black and white perception, function in low illumination, detect motion from the periphery, and are poor at resolving details. The cone section notes that cones enable color perception, operate under high illumination, and are effective for detecting details on objects, contributing to acute (foveal) vision. The background is minimalistic, focusing attention on the text and the scientific image. The formatting is clear, making it easy to read and understand, reinforcing the complex biological functions of the eye's photoreceptors. ``` Rods and cones varying properties Rods: – B/W perception (process light - not colors) – Low illumination – Detect motion from periphery – Poor at resolving details Cones: – Color perception – High illumination – Detect details on objects (acuity → foveal vision) , 2005 to 2021 ``` ====== The content is divided into two sections, each featuring distinct visual elements and information about photoreceptors and color vision. The first section presents a list titled "Four kinds of Photoreceptors," highlighting the different types of photoreceptors in the visual system. It indicates that there are rods, which are responsible for black and white vision, and three types of cones that detect color: long-wavelength light (red), middle-wavelength light (green), and short-wavelength light (blue). Each type of cone is associated with a specific wavelength in nanometers (nm). The section concludes with a note that these three cone types contribute to the perception of the entire color spectrum. The second section features an illustration that appears to be a scientific diagram pertaining to color vision in fish. On the left side, there is a close-up depiction of cone cells, with indications of their shapes and arrangements—possibly illustrating the diversity among species. On the right side, another diagram showcases multiple shapes resembling cones colored in blue, green, and yellow. The overall layout alternates between informative text about photoreceptors and illustrative visuals, establishing a connection between theoretical concepts and biological structures relevant to color vision. ``` Four kinds of Photoreceptors Rods (B/W) Three types of cones: – long-wavelength light (red) (~559 nm) – middle-wavelength light (green) (~531 nm) – short-wavelength light (blue) (~419 nm) 3 cone types → perception of entire color spectrum , 2005 to 2021 LAYNE and MACNICHOL. 1. COLOR VISION IN FISHES CONES CELLS of the fish retina have mapped 500 receptors, differ according to shape and arrangement. ``` ====== The visual consists of two sections focusing on the anatomy of the human retina, specifically comparing the distribution of rods and cones. The upper section features a detailed diagrammatic cross-section of the human fovea, displaying various layers of retinal cells and emphasizing the blind spot. It illustrates that rods outnumber cones by a significant margin (20 times) as one moves away from the fovea. A side note indicates that only 1% of the total number of cones are concentrated in the fovea itself. In the lower section, a graph depicts the number of rods and cones as a function of the angle of visual perception, illustrating that cones are densely packed in the fovea and sharply decline in number as one moves toward the periphery, where rods are predominant. The caption highlights that this distribution affects ambient and peripheral vision. The color scheme includes shades of red and blue for the graphs, while the diagrams use a neutral palette with labels in black and gray to make the content clear and readable. ``` Away from fovea, rods outnumber cones 20x 1% of total number of cones are concentrated in fovea Rods and cones are not distributed the same across retina Ambient/peripheral vision Fovea ``` ====== The first segment depicts a stylized representation of the eye's anatomy, focusing on the optic nerve and receptor cells. The color scheme employs gradients of red and yellow, suggesting a biological context. The region labeled "Blind spot" indicates an area where there are no photoreceptor cells, highlighting an important aspect of vision. The "Receptors" are depicted along the edges, emphasizing their role in image processing before transmitting signals via the optic nerve. The diagram's intricate details appear to showcase the cellular structure relevant to visual perception. The second segment illustrates the distribution and types of cone cells in the human fovea. The image uses a dense arrangement of colored cells to represent cones sensitive to different wavelengths of light: short (blue), medium (green), and long (red). The text below the visual clearly identifies these wavelengths, identifying their significance in color perception. The overall aesthetic communicates a life sciences theme, with a focus on ocular biology. ``` 26 Blind spot Receptors Optic nerve © Cengage Learning Figure 2-6 p.25 27 Cones in the human fovea Short wavelength (419 nm) Medium wavelength (531 nm) Long wavelength (559 nm) ``` ====== The content consists of two sections focusing on vision, particularly the roles of rods and cones in the retina, and the connections within the visual system of the brain. The first section features a visual representation of a monkey's retina, illustrating the distribution of rods (small dots) and cones (large dots). Here, it is emphasized that rods outnumber cones significantly, highlighting their importance in peripheral vision and low-light conditions. The background is filled with green and yellow dots representing the photoreceptor cells, with a focus on the spatial relationship between these two types of cells. The second section provides a labeled diagram depicting major connections in the visual system of the brain. It illustrates the pathways from the retina through the optic chiasm to the visual cortex. This portion identifies the right and left visual fields and states that visual perception is divided into two hemifields, noting that the left hemifield projects to the right visual cortex, while the right hemifield projects to the left visual cortex. The diagram is anatomically oriented, showing the major components like the superior colliculus, lateral geniculate nucleus of the thalamus, and the optic nerve, all crucial for visual processing. ``` Rods and cones in the periphery Rods (small dots) outnumber the cones (large dots) (picture from a monkey's retina) Major connections in the visual system of the brain RIGHT LEFT Visual cortex (also known as striate cortex) Retina Optic chiasm Superior colliculus Visual perception divided into 2 space hemifields Left hemifield projects into right visual cortex Right hemifield projects into left visual cortex ``` Book Chapter C H A P T E R 2 The Beginning of the Perceptual Process CHAPTER CONTENTS Perception Starting at the Beginning Light, the Eye, and the Visual Receptors Light: The Stimulus for Vision The Eye Adapting to the Dark Spectral Sensitivity Electrical Signals in Neurons Recording Electrical Signals in Neurons Convergence Causes the Rods to Be More Sensitive Than the Cones Lack of Convergence Causes the Cones to Have Better Acuity Than the Rods SOMETHING TO CONSIDER: Early Events Focusing Light Onto the Receptors Receptors and Perception Transforming Light Energy Into Electrical Energy Basic Properties of Action Potentials Chemical Basis of Action Potentials Transmitting Information Across a Gap Neural Convergence and Are Powerful DEVELOPMENTAL DIMENSION: Infant Visual Acuity THINK ABOUT IT Some Questions We Will Consider: How does the focusing system at the front of our eye affect our perception? (p. 25) How do chemicals in the eye called visual pigments affect our perception? (p. 30) How can the way neurons are “wired up” affect perception? (p.!39) How does a tree become a perception of a tree? One way to answer this question is to refer back to the percep- tual process shown in Figure 1.1: Information about the tree (distal stimulus) is carried in light re!ected from the tree and into the eye. When this light reaches the receptors in the retina, creating the proximal stimulus, it becomes transformed into electrical signals that contain information about the tree, which are transmitted to the brain, where eventually these elec- trical signals become transformed into a perception of the tree. In this chapter we will focus on the beginning of the per- ceptual process. Although we will use visual examples to de- scribe the initial processes of the perceptual process, many of the principles we will be describing hold for the other senses as well. Just as the person from Chapter 1 sees the tree because light is re!ected from it into his eyes, he hears the rustle of its branches because sound energy in the form of pressure changes in the air enters his ears. In both cases, stimuli trigger a process that ends up with perception occurring as a result of activity in the brain. Similar events occur for feeling the texture of the tree’s bark, smelling its blossoms, and tasting its fruit. By the time you "nish this book, you will see that although there are numerous differences between the senses, they all operate ac- cording to similar principles. Starting at the Beginning The idea that perception starts at the beginning of the percep- tual process may sound obvious. But as we will see, there is enough going on right at the beginning of the perceptual pro- cess to "ll a whole chapter and more, and most of what goes on can affect perception. So, the "rst step in understanding per- ception is to take a close look at the processes that begin, in the case of vision, with light re!ected from an object into the eye. Figure 2.1 shows the "rst four steps of the visual process, which starts on the right and moves to the left to match the perceptual process in Figure 1.1. Following the sequence of the physical events in the process, shown in black along the bottom of the "gure, we begin with Step 1, the distal stimulus (the tree); then move to Step 2, in which light is re!ected from the tree and enters the eye to create the proximal stimulus on 21 Copyright 2017 Cengage Learning. All Rights Reserved. May not be copied, scanned, or duplicated, in whole or in part. Due to electronic rights, some third party content may be suppressed from the eBook and/or eChapter(s). Editorial review has deemed that any suppressed content does not materially affect the overall learning experience. Cengage Learning reserves the right to remove additional content at any time if subsequent rights restrictions require it.Rod Cone Seeing fine details Seeing in dim light Seeing in focus STEP 4 Neural processing: Signals travel in a network of neurons. STEP 3 Receptor processes: Receptors transform light into electricity. STEP 2 Light is reflected and focused to create an image of the tree on the retina. STEP 1 Distal stimulus: The tree Figure 2.1 Chapter preview. This chapter will describe the !rst three steps of the perceptual process for vision and will introduce Step 4. Physical processes are indicated in black; the perceptual outcomes of these processes are indicated in blue. the visual receptors; then to Step 3, in which receptors trans- form light into electrical signals; and "nally to Step 4, in which electrical signals are “processed” as they travel through a net- work of neurons. Our goal in this chapter is to show how these physical events in!uence the following aspects of perception, shown in blue in Figure 2.1: (1) seeing in focus, (2) seeing in dim light, and (3) seeing "ne details. We begin by describing light, the eye, and the receptors in the retina that line the back of the eye. Visible light, the energy within the electromagnetic spectrum that humans can perceive, has wavelengths rang- ing from about 400 to 700 nanometers (nm), where 1 nano- meter 5 1029 meters, which means that the longest visible wavelengths are slightly less than one-thousandth of a millimeter long. For humans and some other animals, the wavelength of visible light is associated with the different colors of the spectrum, with short wavelengths appearing blue, middle wavelengths green, and long wavelengths yel- low, orange, and red. Light, the Eye, and the Visual Receptors The ability to see a tree, or any other object, depends on light being re!ected from that object into the#eye. Light: The Stimulus for Vision Vision is based on visible light, which is a band of energy within the electromagnetic spectrum. The electromagnetic spectrum is a continuum of electromagnetic energy that is produced by electric charges and is radiated as waves (see Figure# 1.21, page# 18). The energy in this spectrum can be described by its wavelength—the distance between the peaks of the elec- tromagnetic waves. The wavelengths in the electromagnetic spectrum range from extremely short-wavelength gamma rays (wavelength 5 about 10212 meters, or one ten-billionth of a meter) to long-wavelength radio waves (wavelength 5 about 104 meters, or 10,000 meters). 22 CHAPTER 2 The Beginning of the Perceptual Process The Eye The eyes contain the receptors for vision. The "rst eyes, which appeared back in the Cambrian period (570–500 million years ago), were eyespots on primitive animals such as !at- worms that could distinguish light from dark but couldn’t detect features of the environment. Detecting an object’s details didn’t become possible until more sophisticated eyes evolved to include optical systems that could produce im- ages and therefore provide information about shapes and details of objects and the arrangement of objects within scenes (Fernald, 2006). Light re!ected from objects in the environment enters the eye through the pupil and is focused by the cornea and lens to form sharp images of the objects on the retina, the network of neurons that covers the back of the eye and that contains the receptors for vision (Figure 2.2a). There are two types of visual receptors, rods and cones, so called because of the rod- and cone-shaped outer segments (Figure 2.3). The outer seg- ments are the part of the receptor that contains light-sensitive Copyright 2017 Cengage Learning. All Rights Reserved. May not be copied, scanned, or duplicated, in whole or in part. Due to electronic rights, some third party content may be suppressed from the eBook and/or eChapter(s). Editorial review has deemed that any suppressed content does not materially affect the overall learning experience. Cengage Learning reserves the right to remove additional content at any time if subsequent rights restrictions require it.Optic nerve fibers Receptor cells (rods and cones) Back of eye Light Pupil Cornea Fovea (point of central focus) Optic nerve Retina Lens Retina (a) (b) Figure 2.2 An image of the tree is focused on the retina, which lines the back of the eye. The close-up of the retina on the right shows the receptors and other neurons that make up the retina. Rod Cone Pigment epithelium chemicals called visual pigments that react to light and trigger electrical signals. Signals from the receptors !ow through the network of neurons that make up the retina (Figure 2.2b) and emerge from the back of the eye in the optic nerve, which con- tains a million optic nerve "bers that conduct signals toward the brain. The rod and cone receptors not only have different shapes, they are also distributed differently across the retina. From Figure 2.4, which indicates the rod and cone distributions, we can conclude the following: 1. One small area, the fovea, contains only cones. When we look directly at an object, the object’s image falls on the fovea. 2. The peripheral retina, which includes all of the retina outside of the fovea, contains both rods and cones. It is important to note that although the fovea has only cones, there are also many cones in the peripheral retina. Rod The fovea is so small (about the size of this “o”) that it contains only about 1 percent, or 50,000, of the 6 mil- lion cones in the retina (Tyler, 1997a, 1997b). 3. The peripheral retina contains many more rods than cones because there are about 120 million rods and only 6 million cones in the retina. One way to appreciate the fact that the rods and cones are distributed differently in the retina is by considering what happens when functioning receptors are missing from one area of the retina. A condition called macular degenera- tion, which is most common in older people, destroys the cone-rich fovea and a small area that surrounds it. (Macula is a term usually associated with medical practice that in- cludes the fovea plus a small area surrounding the fovea.) This creates a blind region in central vision, so when a per- son looks directly at something, he or she loses sight of it (Figure 2.5a). Cone Outer segment Figure 2.3 (a) Scanning electromicrograph of the rod and cone receptors in the retina, showing the rod-shaped and cone-shaped receptor outer segments. (b) Rod and cone receptors, showing the inner and outer segments. The outer"segments contain the light-sensitive visual pigment. (From Lewis et al., 1969) Inner segment Rod Cone (a) (b) Light, the Eye, and the Visual Receptors 23 Copyright 2017 Cengage Learning. All Rights Reserved. May not be copied, scanned, or duplicated, in whole or in part. Due to electronic rights, some third party content may be suppressed from the eBook and/or eChapter(s). Editorial review has deemed that any suppressed content does not materially affect the overall learning experience. Cengage Learning reserves the right to remove additional content at any time if subsequent rights restrictions require it.Fovea Blind spot (no receptors) Cones Rods 808 608 408 808 608 408 208 Blind spot 08 Fovea 208 Number of receptors per square millimeter 180,000 160,000 140,000 120,000 100,000 80,000 60,000 40,000 20,000 Optic nerve 0 708 608 508 408 308 208 108 08 108 208 308 408 508 608 708 808 Angle (degree) Figure 2.4 The distribution of rods and cones in the retina. The eye on the left indicates locations in degrees relative to the fovea. These locations are repeated along the bottom of the chart on the right. The vertical brown bar near 20 degrees indicates the place on the retina where there are no receptors because this is where the ganglion cells leave the eye to form the optic nerve. (Adapted from Lindsay & Norman, 1977) © Bruce Goldstein (a) (b) Figure 2.5 (a) In a condition called macular degeneration, the fovea and surrounding area degenerate, so the person cannot see whatever he or she is looking at. (b) In retinitis pigmentosa, the peripheral retina initially degenerates and causes loss of vision in the periphery. The resulting condition is sometimes called “tunnel vision.” Another condition, called retinitis pigmentosa, is a degeneration of the retina that is passed from one genera- tion to the next (although not always affecting everyone in a family). This condition first attacks the peripheral rod receptors and results in poor vision in the peripheral vi- sual field (Figure 2.5b). Eventually, in severe cases, the fo- veal cone receptors are also attacked, resulting in complete blindness. Before leaving the rod–cone distribution shown in Figure# 2.4, note that there is one area in the retina, indi- cated by the vertical brown bar, where there are no receptors. Figure! 2.6 shows a close-up of the place where this occurs, which is where the nerve "bers that make up the optic nerve leave the eye. Because of the absence of receptors, this place is called the blind spot. Although you are not normally aware 24 CHAPTER 2 The Beginning of the Perceptual Process of the blind spot, you can become aware of it by doing the following demonstration. DEMONSTRATION Becoming Aware of the Blind Spot Place the book (or your electronic device if you are reading the ebook) on your desk. Close your right eye, and position yourself above the book/device so that the cross in Figure 2.7 is aligned with your left eye. Be sure the book page is "at and, while look- ing at the cross, slowly move closer. As you move closer, be sure not to move your eye from the cross, but at the same time keep noticing the circle off to the side. At some point, around 3!to 9 inches from the book/device, the circle should disappear. When this happens, the image of the circle is falling on your blind spot. Copyright 2017 Cengage Learning. All Rights Reserved. May not be copied, scanned, or duplicated, in whole or in part. Due to electronic rights, some third party content may be suppressed from the eBook and/or eChapter(s). Editorial review has deemed that any suppressed content does not materially affect the overall learning experience. Cengage Learning reserves the right to remove additional content at any time if subsequent rights restrictions require it.Receptors Blind spot Optic nerve Figure 2.8 View the pattern as described in the text, and observe what happens when the center of the wheel falls on your blind spot. (Adapted from Ramachandran, 1992) Figure 2.6 There are no receptors at the place where the optic nerve leaves the eye. This enables the receptor’s ganglion cell !bers to #ow into the optic nerve. The absence of receptors in this area creates the blind spot. Focusing Light Onto the!Receptors Figure 2.7 Blind spot demonstration. Why aren’t we usually aware of the blind spot? One reason is that the blind spot is located off to the side of our visual "eld, where objects are not in sharp focus. Because of this and because we don’t know exactly where to look for it (as opposed to the demonstration, in which we are focusing our attention on the circle), the blind spot is hard to detect. But the most important reason that we don’t see the blind spot is that some mechanism in the brain “"lls in” the place where the image disappears (Churchland & Ramachandran, 1996). The next demonstration illustrates an important prop- erty of this "lling-in process. DEMONSTRATION Filling In the Blind Spot Close your right eye and, with the cross in Figure 2.8 lined up with your left eye, move toward the “wheel”. When the center of the wheel falls on your blind spot, notice how the spokes of the wheel #ll in the hole (Ramachandran, 1992). These demonstrations show that the brain does not "ll in the area served by the blind spot with “nothing”; rather, it cre- ates a perception that matches the surrounding pattern—the white page in the "rst demonstration, and the spokes of the wheel in the second one. This “"lling in” is a preview of one of the themes of the book: how the brain creates a coherent per- ception of our world. For now, however, we return to the begin- ning of the perceptual process, as light re!ected from objects in the environment is focused onto the receptors. Light re!ected from an object into the eye is focused onto the# retina by a two-element optical system: the cornea and the lens. The cornea, the transparent covering of the front of the eye, accounts for about 80 percent of the eye’s focusing power, but like the lenses in eyeglasses, it is "xed in place so it can’t adjust its focus. The lens, which supplies the remaining 20#percent of the eye’s focusing power, can change its shape to adjust the eye’s focus for objects located at different distances. This change in shape is achieved by the action of ciliary muscles, which increase the focusing power of the lens (its ability to bend light) by increasing its curvature (compare Figure 2.9b and Figure 2.9c). We can understand why the eye needs to adjust its focus by "rst considering what happens when the eye is relaxed and a person with normal (20/20) vision views a small object that is far away. If the object is located more than about 20 feet away, the light rays that reach the eye are essentially parallel (Figure!2.9a), and the cornea–lens combination brings these parallel rays to a focus on the retina at point A. But if the ob- ject moves closer to the eye, the light rays re!ected from this object enter the eye at more of an angle, and this pushes the focus point back so if the back of the eye weren’t there, light would be focused at point B (Figure 2.9b). Because the light is stopped by the back of the eye before it reaches point B, the image on the retina is out of focus. If things remained in this state, the person would see the object as blurred. The adjustable lens, which controls a process called accommodation, comes to the rescue to help prevent blurring. Accommodation is the change in the lens’s shape that oc- curs when the ciliary muscles at the front of the eye tighten Focusing Light Onto the!Receptors 25 Copyright 2017 Cengage Learning. All Rights Reserved. May not be copied, scanned, or duplicated, in whole or in part. Due to electronic rights, some third party content may be suppressed from the eBook and/or eChapter(s). Editorial review has deemed that any suppressed content does not materially affect the overall learning experience. Cengage Learning reserves the right to remove additional content at any time if subsequent rights restrictions require it.and increase the curvature of the lens so that it gets thicker (Figure!2.9c). This increased curvature increases the bending of the light rays passing through the lens so the focus point is pulled back to A to create a sharp image on the retina. This means that as you look around at different objects, your eye is constantly adjusting its focus by accommodating, especially for nearby objects. The following demonstration shows that this is necessary because everything is not in focus at once. an object that is at least 20 feet away. As you stay focused on the faraway object, notice the pencil point without actually look- ing at it (be sure to stay focused on the far object). The point will probably appear slightly blurred. Then slowly move the pencil toward you while still looking at the far object. Notice that as the pencil moves closer, the point becomes more blurred. When the pencil is about 12 inches away, shift your focus to the pencil point. This shift in focus causes the pencil point to appear sharp, but the far object is now out of focus. DEMONSTRATION Becoming Aware of What Is in Focus Accommodation occurs unconsciously, so you are usually un- aware that the lens is constantly changing its focusing power to let you see clearly at different distances. This unconscious focusing process works so ef#ciently that most people assume that everything, near and far, is always in focus. You can demon- strate that this is not so by holding a pen or a pencil, point up, at arm’s length, closing one eye, and looking past the pencil at Lens When you changed focus from far away to the nearby pencil point during this demonstration, you were changing your ac- commodation. Either near objects or far objects can be in focus, but not both at the same time. Accommodation, therefore, makes it possible to adjust vision for different distances. However, as people get older, their ability to accommodate decreases due to hardening of the lens and weakening of the ciliary muscles, and Cornea Retina A (a) Object far— eye relaxed Focus on retina (d) Myopia— eye relaxed Focus in front of retina Moving object closer pushes focus point back B (b) Object near— eye relaxed Focus behind retina Accommodation brings focus point forward Corrective lens (e) Correction of myopia A (c) Object near— accommodation Focus on retina Figure 2.9 Focusing of light rays by the eye. (a) Rays of light coming from a small light source that is more than 20 feet away are approximately parallel. The focus point for parallel light is at A on the retina. (b) Moving an object closer to the relaxed eye pushes the focus point back. Here the focus point is at B, but light is stopped by the back of the eye, so the image on the retina is out of focus. (c) Accommodation of the eye (indicated by the fatter lens) increases the focusing power of the lens and brings the focus point for a near object back to A on the retina, so it is in focus. This accommodation is caused by the action of the ciliary muscles, which are not shown. (d) In the myopic (nearsighted) eye, parallel rays from a distant spot of light are brought to a focus in front of the retina, so distant objects appear blurred. (e) A corrective lens bends light so it is focused on the retina. 26 CHAPTER 2 The Beginning of the Perceptual Process Copyright 2017 Cengage Learning. All Rights Reserved. May not be copied, scanned, or duplicated, in whole or in part. Due to electronic rights, some third party content may be suppressed from the eBook and/or eChapter(s). Editorial review has deemed that any suppressed content does not materially affect the overall learning experience. Cengage Learning reserves the right to remove additional content at any time if subsequent rights restrictions require it.so they become unable to accommodate enough to see objects, or read, at close range. This loss of the ability to accommodate, called presbyopia (for “old eye”), can be dealt with by wearing reading glasses, which brings near objects into focus by replacing the focusing power that can no longer be provided by the lens. Another problem that can be solved by a corrective lens is myopia, or nearsightedness, an inability to see distant objects clearly. The reason for this dif"culty, which affects more than 70 million Americans, is illustrated in Figure 2.9d. Myopia oc- curs when the optical system brings parallel rays of light into focus at a point in front of the retina, so the image that reaches the retina is blurred. This problem can be caused by either of two factors: (1) refractive myopia, in which the cornea and/ or the lens bends the light too much, or (2) axial myopia, in which the eyeball is too long. Either way, images of faraway objects are not focused sharply, so objects look blurred. Cor- rective lenses can solve this problem, as shown in Figure 2.9e. Finally, people with hyperopia, or farsightedness, can see distant objects clearly but have trouble seeing nearby objects because the focus point for parallel rays of light is located be- hind the retina, usually because the eyeball is too short. Young people can bring the image forward onto the retina by accom- modating. However, older people, who have dif"culty accom- modating, often use corrective lenses that bring the focus point forward onto the retina. Focusing an image clearly onto the retina is the initial step in the process of vision, but although a sharp image on the ret- ina is essential for clear vision, we do not see the image on the retina. Vision occurs not in the retina but in the brain. Before the brain can create vision, the light on the retina must activate the visual receptors in the retina. Receptors and Perception Light entering visual receptors triggers electrical signals when the light is absorbed by light-sensitive visual pigment molecules in the receptors. This step is crucial for vision because it creates electrical signals that eventually signal the properties of the distal stimulus to the brain. But the importance of these visual pigments extends beyond triggering electrical signals. Visual pigments also shape our perceptions by determining our abil- ity to see dim lights and our ability to see light in different parts of the visual spectrum. In this section, we "rst describe transduction, and then how the receptors shape perception. Transforming Light Energy Into Electrical!Energy Transduction is the transformation of one form of energy into another form of energy (see Chapter 1, page 7). Visual trans- duction occurs in the rod and cone receptors, which transform light into electricity. The starting point for understanding how the rods and cones create electricity are the millions of mol- ecules of a light-sensitive visual pigment that are contained in the outer segments of the receptors (Figure 2.3). Visual pig- ments have two parts: a long protein called opsin and a much smaller light-sensitive component called retinal. Figure 2.10a shows a model of a retinal molecule attached to opsin (Wald, 1968). Note that only a small part of the opsin is shown here; it is actually hundreds of times longer than the retinal. Despite its small size compared to the opsin, retinal is the crucial part of the visual pigment molecule, because when the ret- inal and opsin are combined, the resulting molecule absorbs vis- ible light. When the retinal part of the visual pigment molecule absorbs light, the retinal changes its shape, from being bent, as shown in Figure 2.10a, to straight, as shown in Figure 2.10b. This change of shape, called isomerization, creates a chemical chain reaction, illustrated in Figure 2.11, that activates thousands of charged molecules to create electrical signals in receptors. What is important about the chain reaction that follows isomerization is that it ampli"es the effect of isomerization. Isomerizing one visual pigment molecule triggers a chain of chemical reactions that releases as many as a million charged molecules, which leads to activation of the receptor (Baylor, 1992; Hamer et al., 2005). Molecule in dark Retinal isomerized by light Retinal Opsin (a) (b) Figure 2.10 Model of a visual pigment molecule. The horizontal part of the model shows a tiny portion of the huge opsin molecule near where the retinal is attached. The smaller molecule on top of the opsin is the light-sensitive retinal. (a) The retinal molecule’s shape before it absorbs light. (b) The retinal molecule’s shape after it absorbs light. This change in shape, which is called isomerization, triggers a sequence of reactions that culminates in generation of an electrical response in the receptor. © Bruce Goldstein Receptors and Perception 27 Copyright 2017 Cengage Learning. All Rights Reserved. May not be copied, scanned, or duplicated, in whole or in part. Due to electronic rights, some third party content may be suppressed from the eBook and/or eChapter(s). Editorial review has deemed that any suppressed content does not materially affect the overall learning experience. Cengage Learning reserves the right to remove additional content at any time if subsequent rights restrictions require it.One visual pigment molecule Figure 2.11 This sequence symbolizes the chain reaction that is triggered when a single visual pigment molecule is isomerized by absorption of a single photon of light. In the actual sequence of events, each visual pigment molecule activates hundreds more molecules, which, in turn, each activate about a thousand molecules. Isomerization of just one visual pigment molecule activates about a million other molecules, which activates the receptor. METHOD Measuring the Dark Adaptation Curve The #rst step in measuring a dark adaption curve is to have the subject look at a small #xation point while paying attention to a "ashing test light that is off to the side (Figure 2.12). Because the subject is looking directly at the #xation point, its image falls on the fovea, so the image of the test light falls on the pe- ripheral retina, which contains both rods and cones. While still in the light, the subject turns a knob that adjusts the intensity of the "ashing light until it can just barely be seen. This threshold for seeing the light, the minimum amount of energy necessary to just barely see the light, is then converted to sensitivity. Be- cause sensitivity 5 1/threshold, this means that a high threshold corresponds to low sensitivity. The sensitivity measured in the light is called the light-adapted sensitivity, because it is mea- sured while the eyes are adapted to the light. Because the room (or adapting) lights are on, the intensity of the "ashing test light has to be high to be seen. At the beginning of the experiment, then, the threshold is high and the sensitivity is low. Once the light-adapted sensitivity to the "ashing test light is determined, the adapting light is extinguished so the subject is in the dark. The subject continues adjusting the intensity of the "ashing light so he or she can just barely see it, tracking the increase in sensitivity that occurs in the dark. As the subject be- comes more sensitive to the light, he or she must decrease the light’s intensity to keep it just barely visible. The result, shown as the red curve in Figure 2.13, is a dark adaptation curve. Visual pigments not only create electrical signals in the receptors, they also shape speci"c aspects of our perceptions. Next, we will demonstrate how properties of the pigments in- !uence perception. We do this by comparing the perceptions caused by the rod and cone receptors. As we will see, the visual pigments in these two types of receptors in!uence two aspects of visual perception: (1) how we adjust to darkness, and (2) how well we see light in different parts of the spectrum. The dark adaptation curve shows that as adaptation pro- ceeds, the subject becomes more sensitive to the light. Note that higher sensitivity is at the bottom of this graph, so movement of the dark adaptation curve downward means that the subject’s sensitivity is increasing. The red dark adaptation curve indicates that the subject’s sensitivity increases in two phases. It increases rapidly for the "rst 3 to 4 minutes after the light is extinguished and then levels off. At about 7 to 10 minutes, it begins increasing again and continues to do so until the subject has been in the dark for about 20 or 30 minutes (Figure 2.13). The sensitivity at the end of dark adaptation, labeled dark-adapted sensitivity, is about 100,000 times greater than the light-adapted sensitivity measured before dark adaptation began. Dark adaptation was involved in a 2007 episode of the Myth- busters program on the Discovery Channel, which was devoted Adapting to the Dark When we discussed measuring perception in Chapter 1, we noted that when a person goes from a lighted environment to a dark place, it may be dif"cult to see at "rst, but that after some time in the dark, the person becomes able to make out lights and objects that were invisible before (Figure 1.16, page #15). This process of increasing sensitivity in the dark, called dark adaptation, is measured by determining a dark adaptation curve. In this section we will show how the rod and cone receptors control an important aspect of vision: the ability of the visual system to adjust to dim levels of illumination. We will describe how the dark adaptation curve is measured, and how the increase in sensitivity that occurs in the dark has been linked to properties of the rod and cone visual pigments. Measuring the Dark Adaptation Curve The study of dark adaptation begins with measuring the dark adaptation curve, which is the function relating sensitivity to light to time in the dark, beginning when the lights are extinguished. 28 CHAPTER 2 The Beginning of the Perceptual Process Peripheral retina Fixation point Fovea Test light Figure 2.12 Viewing conditions for a dark adaptation experiment. In this example, the image of the !xation point falls on the fovea, and the image of the test light falls on the peripheral retina. Copyright 2017 Cengage Learning. All Rights Reserved. May not be copied, scanned, or duplicated, in whole or in part. Due to electronic rights, some third party content may be suppressed from the eBook and/or eChapter(s). Editorial review has deemed that any suppressed content does not materially affect the overall learning experience. Cengage Learning reserves the right to remove additional content at any time if subsequent rights restrictions require it.Pure cone curve Pure rod curve Both rods and cones Rod light-adapted sensitivity Low Cone light-adapted sensitivity Logarithm of sensitivity Figure 2.13 Three dark adaptation curves. The red line is the two-stage dark adaptation curve, with an initial cone branch and a later rod branch, which occurs when the test light is in the peripheral retina, as shown in Figure 2.12. The green line is the cone adaptation curve, which occurs when the test light falls on the fovea. The purple curve is the rod adaptation curve measured in a rod monochromat. Note that the downward movement of these curves represents an increase in sensitivity. The curves actually begin at the points indicating “light- adapted sensitivity,” but there is a slight delay between the time the lights are turned off and when measurement of the curves begins. C Maximum cone sensitivity Rod–cone break High Dark-adapted sensitivity R Maximum rod sensitivity 10 20 Time in dark (min) to investigating myths about pirates. One of the myths was that pirates wore eye patches to preserve night vision in one eye so that when they went from the bright light outside to the dark- ness below decks, removing the patch would enable them to see. To determine whether this would work, the Mythbusters car- ried out some tasks in a dark room just after both of their eyes had been in the light and did some different tasks with an eye that had previously been covered with a patch for 30#minutes. It isn’t surprising that they completed the tasks much more rap- idly when using the eye that had been patched. Anyone who has taken a course on sensation and perception could have told the Mythbusters that the eye patch would work because keeping an eye in the dark triggers the process of dark adaptation, which causes the eye to increase its sensitivity in the dark. Whether pirates actually used patches to help them see below decks remains an unproven hypothesis. One argument against the idea that pirates wore eye patches to keep their sen- sitivity high is that patching one eye causes a decrease in depth perception, which might be a serious disadvantage when the pirate is working on deck. We will discuss why two eyes are important for depth perception in Chapter 10. Although the Mythbusters showed that dark adapting one eye made it easier to see with that eye in the dark, we have a more speci"c goal. We are interested in showing that the "rst part of the dark adaptation curve is caused by the cones and the sec- ond part is caused by the rods. We will do this by running two additional dark adaptation experiments, one measuring adapta- tion of the cones and another measuring adaptation of the rods. Measuring Cone Adaptation The reason the red curve in Figure 2.13 has two phases is that the !ashing test light fell on the peripheral retina, which contains both rods and cones. To measure dark adaptation of the cones alone, we have to en- sure that the image of the test light falls only on cones. We achieve this by having the subject look directly at the test light so its image falls on the all-cone fovea, and by making the test light small enough so that its entire image falls within the fo- vea. The dark adaptation curve determined by this procedure is indicated by the green line in Figure 2.13. This curve, which measures only the activity of the cones, matches the initial phase of our original dark adaptation curve but does not in- clude the second phase. Does this mean that the second part of the curve is due to the rods? We can show that the answer to this question is “yes” by doing another experiment. Measuring Rod Adaptation We know that the green curve in Figure 2.13 is due only to cone adaptation because our test light was focused on the all-cone fovea. Because the cones are more sensitive to light at the beginning of dark adaptation, they control our vision during the early stages of adaptation, so we can’t see what the rods are doing. In order to reveal how the sensitivity of the rods is changing at the very beginning of Receptors and Perception 29 Copyright 2017 Cengage Learning. All Rights Reserved. May not be copied, scanned, or duplicated, in whole or in part. Due to electronic rights, some third party content may be suppressed from the eBook and/or eChapter(s). Editorial review has deemed that any suppressed content does not materially affect the overall learning experience. Cengage Learning reserves the right to remove additional content at any time if subsequent rights restrictions require it.dark adaptation, we need to measure dark adaptation in a per- son who has no cones. Such people, who have no cones because of a rare genetic defect, are called rod monochromats. Their all-rod retinas provide a way for us to study rod dark adap- tation without interference from the cones. (Students some- times wonder why we can’t simply present the test !ash to the peripheral retina, which contains mostly rods. The answer is that there are enough cones in the periphery to in!uence the beginning of the dark adaptation curve.) Because the rod monochromat has no cones, the light- adapted sensitivity we measure just before we turn off the lights is determined by the rods. The sensitivity we determine, which is labeled “rod light-adapted sensitivity” in Figure 2.13, indi- cates that the rods are much less sensitive than the cone light- adapted sensitivity we measured in our original experiment. We can also see that once dark adaptation begins, the rods increase their sensitivity, as indicated by the purple curve, and reach their "nal dark-adapted level in about 25 minutes (Rushton, 1961). The end of this rod adaptation measured in our monochromat matches the second part of the two-stage dark adaptation curve. Based on the results of our dark adaptation experiments, we can summarize the process of dark adaptation. As soon as the light is extinguished, the sensitivity of both the cones and the rods begins increasing. However, because the cones are much more sensitive than the rods at the beginning of dark ad- aptation, we see with our cones right after the lights are turned out. One way to think about this is that the cones have “cen- ter stage” at the beginning of dark adaptation, while the rods are working “behind the scenes.” However, after about 3 to 5 minutes in the dark, the cones have reached their maximum sensitivity, as indicated by the leveling off of the dark adapta- tion curve. Meanwhile, the rods are still adapting, behind the scenes, and by about 7 minutes in the dark, the rods’ sensitivity "nally catches up to the cones’. The rods then become more sensitive than the cones, and rod adaptation, indicated by the second branch of the dark adaptation curve, becomes visible. The place where the rods begin to determine the dark adapta- tion curve is called the rod–cone break. Why do the rods take about 20 to 30 minutes to reach their maximum sensitivity (point R on the curve) compared to only 3 to 4 minutes for the cones (point C)? The answer to this question involves a process called visual pigment regenera- tion, which occurs more rapidly in the cones than in the rods. Visual Pigment Regeneration From our descrip- tion of transduction earlier in the chapter, we know that light causes the retinal part of the visual pigment molecule, which is initially bent as shown in Figure 2.10a, to change its shape as in Figure 2.10b. This change from bent to straight is shown in the upper panels of Figure 2.14, which also shows how the retinal eventually separates from the opsin part of the molecule. This change in shape and separation from the opsin causes the molecule to become lighter in color, a process called visual pigment bleaching. This bleaching is shown in the lower pan- els of Figure 2.14. Figure 2.14a is a picture of a frog retina that was taken moments after it was illuminated with light. The red color is the visual pigment. As the light remains on, more and more of the pigment’s retinal is isomerized and breaks away from the opsin, so the retina’s color changes as shown in Figures 2.14b and 2.14c. When the pigments are in their lighter bleached state, they are no longer useful for vision. In order to do their job of changing light energy into electrical energy, the retinal needs to return to its bent shape and become reattached to the opsin. This process of reforming the visual pigment molecule is called visual pigment regeneration. Figure 2.14 A frog retina was dissected from the eye in the dark and then exposed to light. The top row shows how the relationship between retinal and opsin changes after the retinal absorbs light. Only a small part of the opsin molecule is shown. The photographs in the bottom row show how the color of the retina changes after it is exposed to light. (a) This picture of the retina was taken just after the light was turned on. The dark red color is caused by the high concentration of visual pigment in the receptors that are still in the unbleached state. (b,"c) After the retinal isomerizes, the"retinal and opsin break apart, and the retina becomes bleached, as indicated by the lighter color. Retinal Opsin Opsin Opsin © Bruce Goldstein (a) (b) (c) 30 CHAPTER 2 The Beginning of the Perceptual Process Copyright 2017 Cengage Learning. All Rights Reserved. May not be copied, scanned, or duplicated, in whole or in part. Due to electronic rights, some third party content may be suppressed from the eBook and/or eChapter(s). Editorial review has deemed that any suppressed content does not materially affect the overall learning experience. Cengage Learning reserves the right to remove additional content at any time if subsequent rights restrictions require it.When you are in the light, as you are now as you read this book, some of your visual pigment molecules are isomerizing and bleaching, as shown in Figure 2.14, while at the same time, others are regenerating. This means that in most normal light levels, your eye always contains some bleached visual pigment and some intact visual pigment. When you tur