Chapter 5: Fundamental (Astro) Physics Breakthroughs Enabled by Space PDF
Document Details
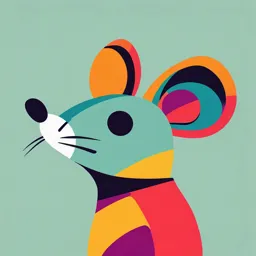
Uploaded by ImportantAltoFlute1375
Tags
Summary
This document details advancements in astrophysics research, focusing on breakthroughs enabled by space-based observations, including the cosmic microwave background radiation and gravitational waves. The content also explores the concept of cosmological redshift and the role of space missions in these advancements.
Full Transcript
# Chapter 5: Fundamental (Astro) Physics Breakthroughs Enabled by Space This chapter shows how observations from space have pushed forward our understanding of astrophysical problems. ## 5.1 The Cosmic Microwave Background: An Evidence of the Big Bang - The Cosmic Microwave Background (CMB) is a...
# Chapter 5: Fundamental (Astro) Physics Breakthroughs Enabled by Space This chapter shows how observations from space have pushed forward our understanding of astrophysical problems. ## 5.1 The Cosmic Microwave Background: An Evidence of the Big Bang - The Cosmic Microwave Background (CMB) is a nearly uniform glow of radiation in the microwaves that pervades the Universe. - It corresponds to a blackbody emission at a temperature of 2.7 K. - It is a fingerprint of the primordial Universe, as it is thought to be the afterglow of the Big Bang. - The Big Bang theory suggests that the Universe began with an extremely hot and dense point that exploded and started to expand. - The Universe went through a phase of accelerated expansion of the space itself, the so-called inflation phase. - At this point, the temperature was so high - billions of degrees Kelvin - that all the matter was in a state of hot, ionized plasma composed only of the fundamental particles of the standard model in a highly energetic and chaotic state, including quarks, electrons, and photons. - As the Universe expanded, it also started to cool down, until about 3000 K, when it became cool enough for hydrogen to recombine and the photons to escape the plasma state. - The Universe became transparent and released these almost homogeneous fingerprints of hot photons that compose the CMB. - With time the Universe kept expanding, and so did the wavelength associated with it. - That is why the measured temperature is much lower than what was initially. - Before talking about how the CMB was discovered and how space missions allowed to really connect the gap between theory and observations in the cosmology field, we need to define a fundamental concept: ### Cosmological Redshift - It is defined as the observed elongation of the wavelengths of the photons emitted by an astronomical object, resulting in "redder wavelengths". - It is caused by a more general physical effect, the Doppler effect. - It was observed (by mistake) for the first time by Penzias and Wilson, in 1964 while performing telecommunications experiments. - This mistake granted them the Nobel Prize in 1978. - Three space missions have significantly enhanced our comprehension of the CMB: - **COBE (COsmic Background Explorer, 1992)** - Measured the spectrum of the CMB (radiation intensity per unit of wavelength) and found two groundbreaking results: - The measured spectrum is an almost perfect black body at the temperature of 2.74 K (Fixsen et al., 1994). - The spatial distribution of the intensity has very small deviations from isotropy (the intensity is the same in all directions). - **WMAP (Wilkinson Microwave Anisotropy Probe, 2002)** - Provided the scientific community with measurements of the CMB temperature fluctuations with an increased spatial resolution. - **Planck (2009)** - Provided an even better angular resolution to map the CMB. - Measurements allowed to put constraints on fundamental cosmological parameters, such as: - Density of Dark Energy to Dark Matter: ~ 7:3 - Density of Dark Energy / Cold Dark matter / Baryonic matter: ~ 25:5:1 - Redshift at decoupling: 1060 ## 5.2 Gravitational Waves - Gravitational waves are ripples in space-time caused by some of the most violent and energetic processes in the Universe. - Albert Einstein first predicted the existence of gravitational waves in 1916 in his general theory of relativity. - Einstein's mathematics showed that massive accelerating objects (things like neutron stars or black holes orbiting each other) would disrupt space-time in such a way that waves of undulating space-time would propagate in all directions away from the source. - These cosmic ripples would travel at the speed of light, carrying information about their origins, as well as clues to the nature of gravity itself. - The strongest gravitational waves are produced by cataclysmic events such as merging black holes, neutron stars, SN (massive stars exploding at the end of their lifetimes), etc. - Other gravitational waves are predicted to be caused by the rotation of neutron stars that are not perfect spheres, and possibly even the remnants of background gravitational waves created by the Big Bang. ### Observations of Gravitational Waves - Direct observation of gravitational waves was not possible for the many decades after they were predicted due to the minuscule effect that would need to be detected and separated from the background of vibrations present everywhere on Earth. - A technique called interferometry was suggested in the 1960s and eventually, technology developed sufficiently for this technique to become feasible. ## 5.3 Dark matter, Exotic Particles - For a long time, astronomers thought that dark matter might be hidden in objects that appear dark because they emit no light (e.g., black holes) or that are too faint to be observed at large distances (e.g., planets or white dwarfs). - However, these objects would be made of ordinary matter. - The deuterium abundance tells us that no more than 5% of the critical density consists of ordinary matter. - Another possible form that dark matter can take is some type of elementary particle that we have not yet detected here on Earth - a particle that has mass and exists in sufficient abundance to contribute 25% of the critical density. - Some physics theories predict the existence of such particles. - One class of these particles has been given the name WIMPS, which stands for weakly interacting massive particles. - Since these particles do not participate in nuclear reactions leading to the production of deuterium, the deuterium abundance puts no limits on how many WIMPS might be in the Universe. - If large numbers of WIMPs do exist, then some of them should be passing through us right now. - Since by definition they interact only weakly (or infrequently) with other matter, the chances that they will have a measurable effect are small. - Many experiments designed to detect WIMPs are in operation or in the planning stages. ## 5.4 Matter and Energy in Our Universe - By making accurate measurements of the cosmic microwave background fluctuations, the Wilkinson Microwave Anisotropy Probe (WMAP) measured the basic parameters of the Big Bang model including the density and composition of the Universe. - WMAP measured the relative density of baryonic and non-baryonic matter. - The composition of the Universe is as follows: - Luminous matter such as stars, etc. (1%) - Free hydrogen and helium in interstellar and intergalactic space (4%) - Dark matter (25%): Dark matter is likely to be composed of one or more species of sub-atomic particles that interact very weakly with ordinary matter. Particle physicists have many plausible candidates for the dark matter (such as WIMPs), and new particle accelerator experiments are likely to bring new insight in the coming years. - Dark Energy (70%): The first observational hints of dark energy in the Universe date back to the 1980's when astronomers were trying to understand how clusters of galaxies were formed. In the 1990's, observations of supernovas were used to trace the expansion history of the Universe, and the big surprise was that the expansion appeared to be speeding up, rather than slowing down. If 70% of the energy density in the Universe is in the form of dark energy, which has a gravitationally repulsive effect, it is just the right amount to explain both the flatness of the Universe and the observed accelerated expansion. Thus dark energy explains many cosmological observations at once. ## 5.5 Hubble Space Telescope - The Hubble Space Telescope (HST) is a unique space-based observatory, born from a collaboration between ESA and NASA. - It is important to highlight that it is one of its kind mission since it was the only satellite that has been upgrading instrumentation while operating, and there are no plans of replicating this on future missions. - HST has 4 instruments on board - 2 spectrographs in the ultra-violet, optical, and infrared, and 2 cameras in the optical and infrared. - It was launched in 1990 and it is still operational as of September 2024. - Its wavelength coverage spans from UV to infrared. - It provided the astronomy community with 1.5 million observations that led to groundbreaking discoveries. ### 5.5.1 The HST Deep Fields - The science case for HST was to enable observations deeper into the Universe, in order to allow observations further back in time than what was possible from ground-based telescopes. - A Deep Field (DF) observation is a very long observation - order of days - of the same place in the Universe, possibly an "empty" spot, to be able to look far away in time. - The first HST DF observation lasted 10 days, with the goal of mapping the shape of the galaxies as a function of their distance from Earth. - The majority of these distant galaxies are located so far away that their light is redshifted towards the infrared, and as a consequence they appear very faint in the optical. - The DF observations were repeated three times, with better instruments, to allow to go further back in time: Hubble Deep Field, Hubble Ultra Deep Field, and Hubble Ultra Deep Field-IR. - These long exposures allowed to go further away in time then expected. ## 5.6 High-Energy Astronomy - High-energy astronomy explores the most extreme and dynamic phenomena in the Universe, focusing on objects emitting radiation in X-rays, gamma rays, and cosmic rays. - By studying such high-energy emissions, we can understand violent cosmic phenomena such as binary systems, black holes, neutron stars, supernovae, and active galactic nuclei. ### 5.6.1 X-ray Sources - X-rays, a high-energy form of electromagnetic radiation, are emitted by various sources throughout the Universe. - Some significant X-ray sources are: - **Black holes:** They can emit X-rays when they accrete matter from nearby stars or gas clouds. As material spirals into a black hole's event horizon, it heats up and emits X-rays before disappearing beyond the point of no return. - **Neutron Stars and Pulsars:** Neutron stars, incredibly dense remnants of massive stars, emit X-rays due to their strong magnetic fields and rapid rotation. Pulsars, a type of neutron star, emit regular pulses of X-rays as their magnetic poles sweep across our line of sight. - **Supernova remnants:** When massive stars reach the end of their lives and explode in supernovae, they leave behind expanding remnants. These remnants, filled with high-energy particles, emit X-rays as they interact with surrounding interstellar material. - **Galaxy Clusters:** Galaxy clusters, collections of galaxies held together by gravity, contain hot gas at temperatures of millions of degrees Celsius. This superheated gas emits X-rays through a process called thermal bremsstrahlung as electrons interact with ions. - **Active Galactic Nuclei (AGN):** Supermassive black holes at the centers of galaxies often accrete large amounts of matter. The intense radiation emitted by these accretion disks, along with jets of high-speed particles, produces copious amounts of X-rays in AGN. - **X-ray Binaries:** These systems consist of a normal star and a compact object (like a neutron star or a black hole) orbiting each other. As matter from the normal star falls onto the compact object, it generates X-rays. - **X-ray Background Radiation:** The Universe itself emits a diffuse X-ray glow, often referred to as the cosmic X-ray background. This background radiation is a combination of emissions from various sources over cosmic history. - **Solar corona:** The solar corona is another significant source of X-rays in our Universe. It's the outermost layer of the Sun's atmosphere, extending millions of kilometers into space. The corona emits X-rays due to its incredibly high temperatures, reaching millions of degrees Celsius. ### 5.6.2 X-ray Binaries - Binary systems consist of two stars revolving around a common center of gravity. - A special class that emits X-rays are called X-ray binaries. - They are one of the brightest sources of X-rays in our galaxy. - Such a binary system comprises of a normal star and a compact stellar remnant (such as a white dwarf, neutron star, or black hole). - If the two stars of the binary are close enough, the material from the normal star (or the companion star) is pulled off which then spirals into the primary star (or the compact object) via an accretion disk. - As the material from the accretion disk falls onto the primary star, it can create shocks, heating the in-falling material to very high temperatures (orders of million kelvin) and thus producing vast amounts of X-rays. - X-ray binaries are further subdivided into different subclasses such as the High Mass X-ray Binary (HMXB) and Low Mass X-ray Binary (LMXB). Together they comprise 90% of the total X-ray binaries. ## 5.7 Gamma-ray Bursts - Gamma-ray bursts (GRBs) are bursts of gamma-ray radiation observed within a short time frame. - The first detection, shows two peaks of gamma-ray counts. - GRBs were first observed by the VELA satellites constellation launched in the 1960s. - These satellites operated synchronously and allowed to locate where the event was coming from thanks to a triangulation technique based on the timing at which the photon arrived and the satellite positions. - Over a decade, the VELA mission detected 73 events. - These measurements allowed to rule out that GRBs came from either Earth (at first they were though to be of human origin) or near solar neighbourhood. - There are two types of GRBs, long-duration bursts, linked with the explosion of massive stars, and short-duration bursts, linked to the presence of compact objects, i.e. the merging of two neutron stars. - As mentioned in Section 5.2, mergers of compact objects are a source of gravitational waves. - The first detection of gravitational waves by the collaboration of LIGO and VIRGO allowed astronomers to precisely locate mergers of compact objects. - In combination with the gamma-ray satellites, astronomers have recently been able to improve the localisation of these events. - A further improvement on this topic has been provided by observations in the optical band of the source after the gamma ray burst event, conducted by optical telescopes that can quickly identify (rapid response as mentioned in 5.5.2) a bright source within hours of a GRB detection, and compare this with previous images of the same object. - This is one of the many examples of how multi-messengers astronomy allows to improve our current understanding of the Universe. ## 5.8 Distance Measurements - The Gaia mission is a space observatory launched by the European Space Agency in 2013. - The scope of this mission is to create a precise 3D map of our galaxy. - GAIA was able to measure the distance and motion of a billion stars. - It works in the optical band. - Measured stars in the galactic centre (1 billion stars, 1% of the Milky Way content). - The mission was a success, and astronomers plan on building a similar mission that will cover the infrared region of the spectrum, to be able to observe past the gaseous and dust clouds that are opaque in the optical. ## 5.9 The James Webb Space Telescope - The James Webb Space Telescope (JWST) is a space telescope launched on the 25 December 2021. - Its scope is to investigate the origins of the Universe and our place in it. - It is observing multiple phases of our cosmic history, from the cosmic radiative background to the formation of galaxies, stars and planets, from our solar system and beyond. - JWST is having a big impact inside and outside of the astronomy community, by providing numerous breakthrough results every week that are carefully presented to the public in the form of pretty pictures. - It has dedicated teams for communication to the public, involving scientists, journalists, artists. ### WASP 39-b transit curve - The first image is a transit curve of the hot gas giant WASP 39-b, observed in three different photometric filters, covering a broad wavelength range of 0.5-5.5 μm. - This planet is a gas giant of 0.25 Jupiter masses with a radius of 1.28 Jupiter radii, making it a lighter, bigger Jupiter, i.e. a "puffy" gas giant. - This detection of CO is compatible with having a liquid water ocean around that planet. ## 5.10 Nobel Prizes From Space - The exploration of space carried out starting from last century has allowed numerous new discoveries and advancements in the scientific field. - Table 5.1 shows a list of Nobel prizes awarded to scientists for space-related subjects.