The Working Cell: Cellular Functions, Nanotechnology and Energy - PDF
Document Details
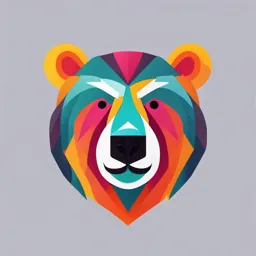
Uploaded by HardyPeninsula9403
BITS Pilani Hyderabad Campus
Tags
Summary
This document is a chapter from a biology textbook, "The Working Cell". It covers core concepts of cellular functions, energy transformations (ATP), and introduces aspects of nanotechnology mimicking natural processes. The chapter examines how cellular structures work, including cellular metabolism and enzymes.
Full Transcript
Okay, here is the converted text from the document or image into a structured markdown format. ### 5 The Working Cell #### Why Cellular Functions Matter * Both nerve gas and insecticides work by crippling a vital enzyme. * For thousands of years, people have used osmosis to preserve food throu...
Okay, here is the converted text from the document or image into a structured markdown format. ### 5 The Working Cell #### Why Cellular Functions Matter * Both nerve gas and insecticides work by crippling a vital enzyme. * For thousands of years, people have used osmosis to preserve food through salt and sugar curing. * You'd have to walk more than 2 hours to burn the calories in half a pepperoni pizza. --- #### CHAPTER CONTENTS * Some Basic Energy Concepts 110 * Energy Transformations: ATP and Cellular Work 113 * Enzymes 114 * Membrane Function 117 #### CHAPTER THREAD * Nanotechnology * BIOLOGY AND SOCIETY Harnessing Cellular Structures 109 * THE PROCESS OF SCIENCE Can Enzymes Be Engineered? 115 * EVOLUTION CONNECTION The Origin of Membranes 121 ### Nanotechnology BIOLOGY AND SOCIETY #### Harnessing Cellular Structures Imagine a tiny movable "car" with balls of carbon atoms for wheels, or a three-dimensional relief map of the world carved onto an object 1,000 times smaller than a grain of sand. These are real-world examples of nanotechnology, the manipulation of materials at the molecular scale. When designing devices of such small size, researchers often turn to living cells for inspiration. After all, you can think of a cell as a machine that continuously and efficiently performs a variety of functions, such as movement, energy processing, and production of various products. Let's consider one example of cell-based nanotechnology and see how it relates to working cells. Researchers at Cornell University are attempting to harvest the energy-producing capability of human sperm cells. Like other cells, a sperm cell generates energy by breaking down sugars and other molecules that pass through its plasma membrane. Enzymes within the cell carry out a process called glycolysis. During glycolysis, the energy released from the breakdown of glucose is used to produce molecules of ATP. Within a living sperm, the ATP produced during glycolysis and other processes provides the energy that propels the sperm through the female reproductive tract. In an attempt to harness this energy-producing system, the Cornell researchers attached three glycolysis enzymes to a computer chip. The enzymes continued to function in this artificial system, producing energy from sugar. The hope is that a larger set of enzymes can eventually be used to power microscopic robots. Such nanorobots could use glucose from the bloodstream to power the delivery of drugs to body tissues, among many other possible tasks. This example is only a glimpse into the incredible potential of new technologies inspired by working cells. *** **Cellular structures.** Even the smallest cell, such as this one from a human pancreas, is a miniature machine of startling complexity. *** In this chapter, we'll explore three processes common to all living cells: energy metabolism, the use of enzymes to speed chemical reactions, and transport regulation by the plasma membrane. Along the way, we'll further consider nanotechnologies that mimic the natural activities of living cells. --- ### Some Basic Energy Concepts Energy makes the world go round-both on a planetary scale and on a cellular scale. But what exactly is energy? Our first step in understanding the working cell is to learn a few basic concepts about energy. #### Conservation of Energy Energy is defined as the capacity to cause change. Some forms of energy are used to perform work, such as moving an object against an opposing force-for example, lifting a barbell against the force of gravity. Imagine a diver climbing to the top of a platform and then diving off (Figure 5.1). To get to the top of the platform, the diver must perform work to overcome the opposing force of gravity. Specifically, chemical energy from food is converted to kinetic energy, the energy of motion. In this case, the kinetic energy takes the form of muscle movement propelling the diver to the top of the platform. What happens to the kinetic energy when the diver reaches the top of the platform? Does it disappear at that point? In fact, it does not. A physical principle known as conservation of energy explains that it is not possible to destroy or create energy. Energy can only be converted from one form to another. A power plant, for example, does not make energy; it merely converts it from one form (such as energy stored in coal) to a more convenient form (such as electricity). That's what happens in the diner's climb up the steps. The kinetic energy of muscle movement is stored as potential energy, the energy an object has because of its location or structure. The energy contained by water behind a dam or by a compressed spring are examples of potential energy. In our example, the diver at the top of the platform has potential energy because of his elevated location. Then the act of diving off the platform into the water converts the potential energy back to kinetic energy. Life depends on countless similar conversions of energy from one form to another. *** **Figure 5.1 Energy conversions during a dive.** An illustration demonstrating all the energy conversions during a dive. * Climbing the steps converts kinetic energy of muscle movement to potential energy. * On the platform, the diver has more potential energy. * Diving converts potential energy to kinetic energy. * In the water, the diver has less potential energy. *** #### Heat If energy cannot be destroyed, where has the energy gone in our example when the diver hits the water? The energy has been converted to heat, a type of kinetic energy contained in the random motion of atoms and molecules. The friction between the body and its surroundings generated heat in the air and then in the water. All energy conversions generate some heat. Although releasing heat does not destroy energy, it does make it more difficult to harness for useful work. Heat is energy in its most disordered, chaotic form, the energy of aimless molecular movement. Entropy is a measure of the amount of disorder or randomness, in a system. Consider an analogy from your own room. It's easy to increase the chaos-in fact, it seems to happen spontaneously! But it requires the expenditure of significant energy to restore order once again. Every time energy is converted from one firm to another, entropy increases. The energy conversions during the climb up the ladder and the dive from the platform in- creased entropy as the diver emitted heat to the surroundings. To climb up the steps again for another dive, the diver must use additional stored food energy. This conversion will also create heat and therefore increase entropy. #### Chemical Energy How can molecules derived from the food we eat provide energy for our working cells? The molecules of food, gasoline, and other fuels have a form of potential energy called chemical energy, which arises from the arrangement of atoms and can be released by a chemical reaction. Carbohydrates, fats, and gasoline have structures that make them especially chemical in energy. Living cells and automobile engines use the same basic process to make the chemical energy stored in their fuels available for work (Figure 5.2). In both cases, this process breaks organic fuel into smaller waste molecules that have much less chemical energy than the fuel molecules did, thereby releasing energy that can be used to perform work. For example, the engine of an automobile mixes oxygen with gasoline (which is why all cars require an air intake system) in an explosive chemical reaction that breaks down the fuel molecules and pushes the pistons that eventually move the wheels. The waste products emitted from the car's exhaust pipe are mostly carbon dioxide and water. Only about 25% of the energy that an automobile engine extracts from its fuel is converted to the kinetic energy of the car's movement. Most of the rest is converted to heat - so much that the engine would melt if the car's radiator did not disperse heat into the atmosphere. That is why high-end performance cars need sophisticated airflow systems to avoid overheating. Cells also use oxygen in reactions that release energy from fuel molecules. As in a car engine, the "exhaust" from such reactions in cells is mostly carbon dioxide and water. The combustion of fuel in cells is called cellular respiration, which is a more gradual and efficient "burning" of fuel compared with the explosive combustion in an automobile engine. Cellular respiration is the energy-releasing chemical breakdown of fuel molecules and the storage of that energy in a form the cell can use to perform work. (We will discuss the details of cellular respiration in chapter 6.) You convert about 34% of your food energy to useful work, such as movement of your muscles. The rest of the energy released by the breakdown of fuel molecules generates body heat. Humans and many other animals can use this heat to keep the body at an almost constant temperature (37°C, or 98.6°F, in the case of humans), even when the surrounding air is much colder. You've probably noticed how quickly a crowded room warms up - it's all that released metabolic heat energy! The liberation of heat energy also explains why you feel hot after exercise. Sweating and other cooling mechanisms enable your body to lose the excess heat, much as a car's radiator keeps the engine from overheating. #### Food Calories Read any packaged food label and you'll find the number of calories in each serving of that food. Calories are units of energy. A calorie (cal) is the amount of energy that can raise the temperature of 1 gram (g) of water by 1°C. You could actually measure the caloric content of a peanut by burning it under a container of water to convert all the stored chemical energy to heat and then measuring the temperature increase of the water. Calories are tiny units of energy, so using them to describe the fuel content of foods is not practical. Instead, it's convenient to use kilocalories (kcal), units of 1,000 calories. In fact, the Calories (capital C) on a food package are actually kilocalories. For example, one peanut has about 5 Calories. That's a lot of energy, enough to increase the temperature of 1 kg (a little more than a quart) of water by 5°C. And just a handful of peanuts contains enough Calories, if converted to heat, to boil 1 kg of water. In living organisms, of course, food isn't used to boil water but instead used to fuel the activities of life. Figure 5.3 shows the number of Calories in several foods and how many Calories are burned by some typical activities. *** **Figure 5.2 Energy conversions in a car and a cell.** In both a car and a cell, the chemical energy of organic fuel molecules is harvested using oxygen. This chemical breakdown releases energy stored in the fuel molecules and produces carbon dioxide and water. The released energy can be used to perform work. *** | | | | :----------------------- | :------------------------------------------- | | Fuel rich in chemical energy | Energy conversion | | Waste products | Poor in chemical energy | | Octane (from gasoline) | Heat energy | | Oxygen | Kinetic energy of movement | | Carbon dioxide | Energy conversion in a car | | Water | Cellular respiration | | Glucose (from food) | ATP Energy for cellular work | | Energy conversion in a cell | Water | *** **Figure 5.3 Some caloric accounting.** *** | Food | Food Calories | Activity | Food Calories consumed per hour by a 150-pound person\* | | :------------------------------- | :------------ | :----------------------- | :------------------------------------------------------- | | Cheeseburger | 295 | Running (7 min/mi) | 979 | | Spaghetti with sauce (1 cup) | 241 | Dancing (fast) | 510 | | Baked potato (plain, with skin) | 220 | Bicycling (10 mph) | 490 | | Fried chicken (drumstick) | 193 | Swimming (2 mph) | 408 | | Bean burrito | 189 | Walking( 3mph | 245 | | Pizza with pepperoni (1 slice) | 181 | Dancing (slow) | 204 | | Peanuts (1 ounce) | 166 | Playing the piano | 73 | | Apple | 81 | Driving a car | 61 | | Garden salad (2 cups) | 56 | Sitting (writing) | 28 | | Popcorn (plain, 1 cup) | 31 | \* | Not including energy necessary for basic functions, such as breathing and heartbeat | | Broccoli (1 cup) | 25 | Food Calories | Food Calories we burn | --- #### ATP and Cellular Work The carbohydrates, fats, and other fuel molecules we obtain from food cannot be used directly as fuel for our cells. Instead, the chemical energy released by the breakdown of organic molecules during cellular respiration is used to generate molecules of ATP. These molecules of ATP than power cellular work. ATP acts like an energy shuttle, storing energy obtained from food and then releasing it as needed at a later time. Such energy transformations are essential for all life on Earth. #### The Structure of ATP The abbreviation ATP stands for adenosine triphosphate. ATP consists of an organic molecule called adenosine plus a tail of three phosphate groups (Figure 5.4). The triphosphate tail is the "business" end of ATP, the part that provides energy for cellular work. Each phosphate group is negatively charged. Negative charges repel each other. The crowding of negative charges in triphosphate tail contributes to the potential energy of ATP. It's analogous to storing energy by compressing a spring; if you release the spring, it will relax, and you can use that springiness to do some useful work. For ATP power, it is the release of the phosphate at the tip of the triphosphate tail the makes energy available to working cells. What remains is ADP, adenosine diphosphate (two phosphate groups instead of three, shown on the right side of Figure 5.4). #### Phosphate Transfer When ATP drives work in cells by being converted to ADP, the released phosphate groups don't just fly off into space. ATP energizes other molecules in cells by transferring phosphate groups to those molecules. When a target molecule accepts the third phosphate group, it becomes energized and can then perform work in the cell Imagine a bicyclist pedaling up a hill. In the muscle cells of the rider's legs, ATP transfers phosphate groups to motor proteins. The proteins than change shape, causing the muscle cells to contract (Figure 5.5a). This contraction provides the mechanical energy needed to propel the rider. ATP also enables the transport of ions and other dissolved substances across the membranes of the rider's nerve cells (Figure 5.5b), helping them send signals to her legs. *** **Figure 5.4 ATP power.** $$adenosine-P-P-P + H_2O \implies adenosine - P - P + P_i$$. *** *** **Figure 5.5 How ATP drives cellular work.** The first part shows **Motor** protein performing mechanical work. The second parts shows **Transport** protein performing transport work. The third part demostrates **Chemical** reaction performing chemical work. *** --- ### The ATP Cycle Your cells spend ATP continuously. Fortunately, it is a renewable resource. ATP can be restored by adding a phosphate group back to ADP. That takes energy, like recompressing a spring. And that's where food enters the picture. The chemical energy that cellular respiration harvests from sugars and other organic fuels used to work regenerating a cell's supply of ATP. Cellular work spends ACP, which is recycled when ADP and phosphate are combined using energy released cellular respiration (Figure 5.6). Thus, energy from processes that yield energy, such as the breakdown of orange fuels, is transferred to processes that consume energy, such as muscle contraction and other cellular work. The ATP cycle can run at an astonishing place: Up to 10 million ATPs are consumed and recycled each second in a working muscle cell. ### Enzymes A living organism contains a vast collection of chemicals, and countless chemical reactions constantly change the organism's molecular makeup. In a sense, a living organism is a complex "chemical square dance" with the molecular "dancers" continually changing partners via chemical reactions. The total of all the chemical reactions in an organism is called metabolism. But almost no metabolic reaction occur without help. Most require the assistance of enzymes, proteins that speed up chemical reactions without being consumed be those reactions. All living cells contain thousands of different enzymes, each promoting a different chemical reaction. #### Activation Energy For a chemical to begin, chemical bonds in the reactant molecules must be broken. (The first step in swapping partners during a square dance to let go of your current partner's hand.) This process requires that the molecules absorb energy from their surrounding. In other words, for most chemical reactions, a cell has to spend a Little energy to make more. You can easily relate this concept to your owe life: it takes to clean your room, but this will save you in the long run because you won't have to hunt you belongines. The energy that must be invested to start a reaction activation energy because the actors and triggers the chemical reaction. Enzymes enable metabolism to occur the amount of activation energy to break the bonds of reactant molecules. If you think activation energy as the amount of a barrier to a chemical reaction, is to lower that barrier (Figure 5.7). It does so by bind to reactant molecules and putting them under physical or physical Stress, making it easier to break the bonds and start a reaction Activation Activation energy barrier. *** | | | | :-------------------------------- | :----------------------------------- | | energy barrier without enzyme | Reduced by enzyme | | | | | Reactant | Activaton | Activation energy barrier with enzyme without enzyme | | Products | Enzymes with reduced product | *** **Figure 5.6 The ATP cycle.** Illustration of the ATP Cycle. * Cellular respiration: chemical energy harvested from fuel molecules goes to ATP * ATP goes to ADP + P * And gives energy for cellular work **Figure 5.7 Enzymes and activation energy.** Illustration of the Activation Energy. ### Nanotechnology The Process of Science Can Enzymes Be Engineered? Like proteins, enzymes are by genes. of suggest ancestral gene and the copies over genetic changes, becoming distinct different functions. scientific experiments. Their experiment used one of this copies of the ### Directed of an During several rounds, The start lactase Enzyme Randomly. The research enzymes resulting determination which The copy for these enzymes that new activity. After rounds, the with researchers Methods properties, one That 1.3 ### Nanotechnology and Can Genes Evolution evolution: ancestral different.