Metal Finishing Processes PDF
Document Details
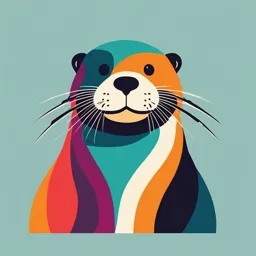
Uploaded by SteadfastRhodium
Tags
Summary
This document provides an overview of metal finishing techniques, specifically emphasizing electroplating principles and processes. It explains the theoretical background, practical considerations, and process parameters involved in this field, providing useful formulas and examples. The text covers various types of metal layers and finishes, including alloys and composites, highlighting the importance of both substrate and coating materials.
Full Transcript
8 Metal finishing Metal finishing is the name given to a wide range of processes carried out in order to modify the surface properties of a metal, e.g. by the deposition of a layer of another metal or a polymer, or by formation of an oxide film. The origins of the industry lay in the desire to enha...
8 Metal finishing Metal finishing is the name given to a wide range of processes carried out in order to modify the surface properties of a metal, e.g. by the deposition of a layer of another metal or a polymer, or by formation of an oxide film. The origins of the industry lay in the desire to enhance the value of metal articles by improving their appearance, but in modern times the importance of metal finishing for purely decorative reasons has decreased. The trend is now towards surface treatments which will impart corrosion resistance or particular physical or mechanical properties to the surface (e.g. electrical conductivity, heat or wear resistance, lubrication or solderability) and, hence, to make possible the use of cheaper substrate metals or plastics covered to give them essential metallic surface properties. A large fraction of the metal objects which we see in everyday life (e.g. car components, kitchen utensils, cans for food, screws and metal window frames) have undergone a metal-finishing process. Moreover, similar processes are an essential part of modern engineering and of the manufacture of electronic components, e.g. printed circuit boards, capacitors and contacts. As a result, the practice of metal finishing extends to many electronics, engineering and metal- processing companies as well as to both large and small specialist firms, resulting in a particularly diverse industry. In general, however, the industry tends to be labour-intensive and dependent on personal experience; the majority of pro- cesses have developed empirically and operator skill is often paramount. It should be emphasized that not all surface finishing is carried out using electrochemical methods, but electroplating, anodizing and other conversion- coating processes, together with electrophoretic painting, represent a large portion of the,industry. This chapter will consider five techniques: (1) electroplating metals, alloys and composites; (2) electroless plating of metals, alloys and composites; (3) immersion plating of metals; (4) chemical conversion coatings based on metal compounds; and (5) electrophoretic painting. 386 Metal finishing 8.1 ELECTROPLATING Electroplating is a very widely used and diverse technology. In principle, the choice of substrate and coating metals may encompass a large number of single metals and alloys; in fact, the substrate may also be a polymer, a ceramic or a composite. The coating may be a single metal, an alloy or, indeed, a metal-polymer or metal-ceramic composite. In practice, the choice is restricted severely by the technical constraints, the possibilities for electrodeposition and the desired deposit properties as well as economic factors. An obvious trend may be noted towards the use of relatively cheap substrates as bulk constructional materials. The more expensive metals are usually encountered as thin coatings. Intermediate metals, such as nickel, may be used either as substrates or coatings. It is noteworthy that aluminium-coated steel would offer a formidable combina- tion of properties, but the development of electroplated aluminium surfaces is severely restricted by the need to utilize fused salts or non-aqueous solvents as electrolytes. 8.1.1 Fundamentals of electroplating The objective of an electroplating process is to prepare a deposit which adheres well to the substrate and which has the required mechanical, chemical and physical properties (section 8.1.5). Moreover, it is of overriding importance that the deposit properties meet their specification on all occasions, i.e. the process is both predictable and reproducible. On the other hand, many metals may (by modification of the bath and plating conditions) be deposited with different properties. It is for this reason that it is not possible to define a single set of conditions for electroplating each metal; the bath, current density, temperature, etc. will depend to some extent on the deposit properties required. It is important that the plating bath is stable for a long period of time because of the importance of reproducibility of the deposit. It is also necessary that the quality of deposit is maintained over a range of operating conditions, since some variations in concentrations and current density are bound to occur, particularly when different objects are to be plated. Tolerance of the bath to carryover from previous process liquors or mishandling during operation on the factory floor is an additional advantage. The principle components of an electroplating process are shown schematically in Fig. 8.1. The essential components include: 1. An electroplating bath containing a conducting salt and the metal to be plated in a soluble form, as well as perhaps a buffer and additives. 2. The electronically conducting cathode, i.e. the workpiece to be plated. 3. The anode (also electronically conducting) which may be soluble or insoluble. 4. An inert vessel to contain (1) --> (3), typically, e.g. steel, rubber-lined steel, polypropylene or polyvinylchloride. 5. A d.c. electrical power source, usually a regulated transformer/rectifier. Electroplating 387 d.c. power supply ne - ne - ~ ~ 0 '-' - - - - - - - --..-- ---- -- Electroplated metal layer Cathode - Anode Ionic transport (workpiece) - - - - - - --c:- - - - - -. - Electroplating tank - t I Electrolyte, i.e. plahng bath (H 2 0 + source of M n ++ conducting salts + buffer + addihves) Fig. 8.1 Principle of electroplating. Electroplating is the process of electrolytically depositing a layer of metal onto a surface. The object to be plated is made the cathode in an electrolyte bath containing a metal ion M n + so that the simplest reaction at the cathode is: (8.1) In the general case, Mn+ may be a simple aquo ion such as hydrated Cu 2 + or it may represent a metal complex such as [Au(CNhr. Where possible, the preferred anode reaction is the dissolution of the same metal to its precursor in solution. M - ne - ------+ M n+ (8.2) and, ideally, the electrolysis conditions are controlled in such a way that the current efficiencies of reactions (8.1) and (8.2) are the same and, hence, the concentration of Mn + in the bath remains constant. In a few cases, the metal ion has to be added as a solid salt and then an inert anode t is employed; the main anode reaction is oxygen evolution. For a successful electroplating process, the correct pretreatment ofthe cathode and careful selection of the anode material, plating bath, current density and t The choice of insoluble anode is very dependent upon the process, e.g. platinized titanium is sometimes used in acid electrolytes, while stainless steel is stable in certain alkaline solutions. 388 Metal finishing other electrolysis conditions, are essential. All these factors will be discussed below. The following types of layer may be electroplated: 1. Single metals: the most important are Sn, Cu, Ni, Cr, Zn, Cd, Pb, Ag, Au and Pt. 2. Alloys: including Cu-Zn, Cu-Sn, Pb-Sn, Sn-Ni, Ni-Co, Ni-Cr and Ni-Fe. 3. Composites: i.e. metals containing dispersed solids such as PTFE, A1 20 3, WC, diamond, SiC, Cr 3C2 and graphite. The mass of electroplated metal w may be expressed in terms of Faraday's laws of electrolysis as follows cpMq W=-- (8.3) nF where M is the molar mass of metal, q is the electrical charge and cp (~ I) is the cathode current efficiency for metal deposition. The majority of electroplating processes are carried out batchwise, at a constant current density I for a measured time t. The averaged rate of mass deposition per unit area is then given by: w ¢/M (8.4) At nF where the factor M/nF is traditionally called the 'electrochemical equivalent'. This expression can also be written in terms of the useful current density. (8.5) At nF Thus, the rate of deposition depends upon the molar mass of the metal M, the number of electrons n per mole of Mn+ and the prevailing current efficiency cp. For some metals, choice of different soluble metal species gives a choice of n, e.g. in the case of copper solutions, in an uncomplexed acid solution, the copper is present as the cupric ion: Cu 2 + +2e- ----->Cu (8.6) while in alkaline cyanide liquors it is present as a copper (I) complex: [CU(CN)2J - + e - -----> Cu + 2CN- (8.7) Obviously, the choice of a low oxidation state source of metal provides a higher deposition rate for a given useful current (or alternatively a given deposition rate can be achieved at a lower current). The current efficiency depends greatly upon the actual process. The majority Electroplating 389 of electroplating baths operate in the range 0.9 < ¢ < 1. Chromium deposition from chromic acid is an important exception, yielding very low values, typically 0.05 < ¢ < 0.20. The most common secondary reaction at the cathode is hydrogen evolution (which may present a safety hazard via spray/mist formation or the build-up of explosive gas mixtures). The averaged rate of deposition may be estimated on a thickness x basis by a consideration of equation (S.4) and assumption (or measurement) of a mass density p value for the plated metal: x/t= ¢iM = ¢IM (S.S) pAnF pnF The quality of an electroplated finish is dependent upon a wide range of process variables, including electrolyte composition and purity, addition agent level, pH, temperature, current density, electrode~anode~cell geometry and flow conditions. Thus, quality-control procedures must consider the track record of both the electrolyte and the deposit. One of the most important parameters is the acceptable or optimum current density range; in this range, the electroplated layer must show satisfactory properties such as brightness, freedom from rough deposits, uniform texture, etc. In order to assess the behaviour of a plating bath, comparative tests may be made in a number of available test cells, the Hull cell (Fig. S.2) being the most common. The inclined cathode of the Hull cell enables a controlled variation of the current density over the cathode surface to be achieved in a single experiment. Typically, a constant cell current in the range 2 to 5 A is used; for chromium plating or high-speed processes, lOA may be preferred. A nomogram is often used to estimate the local current density Ix, which varies with the distance x along the cathode (measured from the end nearest the anode) in the following manner: t (S.9) where a and b are constants depending on the cell size. With an applied current of 2 A, the variation in cathode current density is from approximately 2.5 to S5 mA cm - 2 which covers the working range of most electroplating baths. The Hull cell is also useful as an empirical development tool, e.g. for rapid screening tests on addition agents. The fundamental cell has been modified, for special purposes, in numerous ways, e.g. a bottomless cell may t In the case of the 267 cm 3 cell, equation (8.9) takes the empirical form: Ix= IOi(5.1-5.24Iog1ox) where i is in A, x is in em and Ix is in rnA cm- 2 R.O. Hull (1939) Proc. Amer. Electropl. Soc., 27,52. H. M. Heiling (1956) 'Die Hullzelle', Jahr. Oberflaechentech., 12, 805. 390 Metal finishing 1 % inch a (27'2 in deep )... 5 in ch (a) Applied Current density / Adm-2 current fA Posit ion of Hull cell panel e f ; 0 b c d 9 h i j k I m n 1 5 4 3 2 1.5 1.25 1. 50.5 0.4 0.3 0.2 0.1 0.05 07 2 10 8 6 4 3 2.5 2 1.5 1 0.8 0.6 0.4 0.2 0.1 3 15 12 9 6 4.5 3.75 3 2.25 1.5 1.2 0.9 0.6 0.3 0.15 5 25 20 15 10 7.5 6.25 5 3.75 2.5 2.0 1.5 1.0 0.5 0. 25.. ]]] ] ]]] II ]111 ] ob c d efgh ijklmn (b) Fig. 8.2 The Hull cell. Various standard sizes are available, a common version being the 267 cm 3 cell; a 2 g addition of chemicals corresponds to 1 oz / US gallon in the process bath. (a) Plan view showing the internal dimensions of the American version. (b) Current density ranges along the cathode. Electroplating 391 be immersed directly into the plating tank, or the cathode may be segmented to facilitate d.c. density-distribution measurements. In practice, the device is normally used on a laboratory bench with a simple magnetic stirrer or air agitation. The Hull cell is perhaps best utilized empirically as a simple tool allowing comparative checks to be made on plating baths in order to aid process control (e.g. additive replenishment) and troubleshooting operations. In practical electroplating systems, it is clearly desirable that the deposit should have an even thickness over the whole of the surface to be electroplated. This requires the potential to be the same at all points over the surface of the cathode; this is impossible to attain when the object has a complex shape. To some extent the evenness of the deposit can be improved by introducing auxiliary anodes (usually platinized titanium electrodes where the reaction is oxygen evolution) at various positions in the electrolyte, the objective being to increase the cathode current density at points where it would otherwise be very low (i.e. at points on the cathode furthest from the normal anodes, e.g. in holes or recesses in the object being plated). Areas prone to excess deposition or high current density 'burning' may be treated by the use of auxiliary, dummy cathodes (,robbers' or 'burners') or the use of current shields to increase the solution path between anode and cathode. This approach is still limited, being very dependent on the skill and experience of the operator, involving a specific exercise for a particular workpiece-plating bath combination. Hence, in general, we are dependent on the properties of the plating bath to give a good, even deposit of metal. The ability of a plating bath to give an even deposit is measured by its throwing power which may be determined in the Haring-Blum cell (Fig. 8.3). Two cathodes are placed at markedly different distances from a single anode and electroplating is carried out. The weight of metal plated on the two cathodes WI and W z and the thickness of electroplate on them, XI and X z will be different because the iR drops in the two electrode gaps are not the same; electrode 1 must be a lower overpotential and, hence, less metal will deposit at this cathode. Several formulae have been Cathode 1 Cathode 2 backed with backed with insulator Anode insulator Fig. 8.3 The Haring-Blum cell for the determination of the throwing power of a plating bath (plan view). 392 Metal finishing used to express throwing power but that proposed by Field has some general acceptance t. The Field * formula is:. 100(K -B) % throwmg power = - - - - (S.lO) K+B-2 where K=xdxl and B=W1/W l. This equation is designed to give throwing powers between + 100 (very good) and -100 (very poor). If the cathodes both have a current efficiency near 100%, the deposit weights, W l and W 1 , may be replaced convenie~tly by the corresponding currents to each cathode, i land i1. Factors which determine the throwing power of an electroplating bath include: 1. Conductivity of the electrolyte: a high conductivity will minimize the iR drops in the bath which cause differences in potential over a complex cathode surface and therefore cause the rate of deposition to be more uniform at a given temperature and dissolved-metal concentration. 2. The Tafel slopefor the deposition reaction: it will be seen from the curves in Fig. S.4(a) that for any variation of potential the difference in deposition rate will be smaller when the Tafel slope is high (curve (ii)). Experimentally it is generally found that Tafel slopes are higher when complexing agents and additives, which adsorb on the cathode, are present in solution. Some additives known as 'levellers' and 'brighteners' have the role of ensuring an even deposit on a micro scale; their mode of action will be discussed later. 3. Competing electrode reactions: although gas evolution may be a problem (Chapter 2) the throwing power may be enhanced by the occurrence of side reactions, e.g. hydrogen evolution. In the situation shown in Fig. 8.4(b), hydrogen evolution will occur only at points on the surface where the potential is high. The current for hydrogen evolution will then contribute to the iR drop without leading to metal deposition. Hence, it causes a reduction in the local overpotential, and leads to a more even deposit. It can be seen that the main process parameters which affect the throwing power are the composition of the plating bath (i.e. total electrolyte concentration, complexing agents, pH, additives), temperature and current density. From the viewpoint of energy efficiency, it is attractive for the electro- deposition process to have a high current efficiency and the plating cell to be designed to have a low anode-cathode voltage. In practice, the energy used in the electrolysis itself is commonly low compared with that required to heat the bath and to prepare the surface prior to plating. Moreover, the charge consumed * S. Field, Metal Ind. (London) 1934,44,614. t A useful alternative formula due to D~ A. Luke (Trans. Inst. Metal Finish, 61,64, (1983)) gives throwing power values in the range 0 to 100% and when K = B, the throwing power approaches 50% for reasonably high K values: lOOK % throwing power K+B-l Electroplating 393 log I I -~--- Plating....--- Plating only + (a) (b) H2 evolution Fig.8.4 (a) Tafel plots showing the common effects of the addition of a complexing agent to a plating bath on the reduction of the metal ion. (b) J-E plots for a metal ion and proton reduction in a plating bath. during plating is low (1-10 C cm - 2 for a 10 11m layer) and the value added during the process may be very high. Hence, energy efficiency is not as important as in the large-scale electrolytic processes and this (together with convenience in processing and versatility) accounts for the relatively simple cells employed. It should be noted that the major requirement of any electroplating plant is that satisfactory deposit quality should be achieved, with due regard for costs and convenience in processing. It is, of course, unavoidable that electroplating processes employ solutions containing high concentrations of heavy-metal and transition-metal ions. There is, however, an increasing awareness that the environment must be protected and that particularly toxic solutions should be avoided. Thus, all large electroplating facilities will have a plant for the removal of metal ions and other toxic species from effluent water before it is discharged. Moreover, the trend is towards recovery of the metal in a useful form (Chapter 7) and it is a long-term aim of the industry to employ plating baths which avoid the most toxic chemicals such as chromium (VI), cadmium metal and cyanide ion; some concern is also expressed about the toxicity of certain of the organic additives used to improve the product quality. 8.1.2 The mechanism of electrodeposition of metals In discussing the mechanism by which an electroplate is formed, it must first be recognized that the deposition process has two distinct phases. At the start of the plating process, the deposition of the metal will occur on an electrode surface of a different material, that of the object to be plated. Hence, the first step will be the formation of nuclei of the new phase and their growth into crystals with the characteristic lattice of the electroplating metal. Then, once the electrode is fully covered by a few atomic layers of this metal,one is dealing with a M/M"+ 394 Metal finishing electrode and the thickening of the layer into a macroscopic deposit. In terms of time or charge, the plating process consists almost entirely of the latter process, but the initial stages are also important, since they determine the structure of the primary layer (such factors as the shape and number of growing crystallites) and thereby influence adhesion together with the structure of the final electroplate and its properties. Nucleation and the early stages of phase growth were mentioned briefly in Chapter 1. It may be noted that nucleation is always an improbable event and is achieved at an electrode surface by the application of a large overpotential. The density of nucleation is dependent on the plating bath and is extremely sensitive to the electrode potential (therefore also, of course, the current density). The nuclei, once formed, grow quite rapidly at comparatively low overpotentials, and in a constant-current situation the potential will decrease substantially once nucleation has occurred. The growth of the crystals occurs by incorporation of the individual metal atoms into the crystal lattice. The newly incorporated metal atom is only likely to be stable when it enters the lattice at a site where it interacts with several other atoms already in the lattice. Of the sites which are possible in a perfect crystal lattice (Fig. 8.5), incorporation will be most favourable at a kink site (where the atom can interact with three neighbours) although some incorporation will also take place at edge sites (two neighbours); adatoms (one neighbour) are likely to diffuse to more favourable sites or to redissolve, although a very small number will expand into new nuclei. Clearly, the overall phase growth is a sequence of at least three steps: 1. Mass transport in solution (this may be diffusion, convection or migration) of the metal-bearing species to the electrode surface. 2. Electron transfer to form an adatom. 3. Diffusion of the adatom across the surface into a position in the lattice. r----~ Expanding nucleus ~~ Fig. 8.5 Types of surface site on a perfect crystal. Kink sites are denoted by (1), edge sites by (2) and adatoms by (3). Electroplating 395 The structure of the growing layer will be determined largely by the relative rates of processes (2) and (3) and, in a plating process, the electrolysis conditions, induding the current density. At low current density, the surface diffusion is fast compared with electron transfer and the adatom is likely to end up in a favoured position in the lattice. At higher current densities, surface diffusion is no longer fast compared with electron transfer and further nuclei must form; the layer will be less ordered. Moreover, the presence of suitable bath additives may radically modify the growth process. During most of the electroplating process, the primary metal layer is thickening from a few atomic layers to the desired thickness, perhaps 1-1 0 ~m. In this period the metal deposition reaction occurs effectively at an electrode of the electrodeposited metal. The rate ofthis process may generally be estimated by an equation of the type: _ (rxnF ) 1= nFk CMn+ exp - RT 1] (8.11 ) although this does not necessarily infer that the electrode reaction is simple. In some cases, the electroplating reaction will involve the reduction of a complexed metal species and then the electrode reaction may involve chemical steps, e.g.: [Zn(CN)4]2- +40H- -----+ [Zn(OH)4]2- +4CN- (8.12) [Zn(OH)4]2 - -----+ [Zn(OH)2] + 20H- (8.13) [Zn(OH)2] +2e- -----+Zn+20H- (8.14) Electrochemical experiments tell us little about the thickening process. Instead, information about thick deposits comes from empirical studies and from techniques such as optical microscopy, scanning and transmission electron microscopy (Fig. 8.6) and electron diffraction. On the other hand, the structure and properties of the deposit are totally determined by electrochemical para- meters combined with the properties of the plating bath. Electrodeposits are almost invariably poly crystalline with many grain boundaries and imperfections such as screw dislocations (Fig. 8.7). Screw dislocations provide an edge which does not grow out; rather it rotates around the centre to form a pyramid of stacked rows of edges, the radius of curvature of the emerging dislocation controlling the step spacing on the pyramid. Again, during the thickening stage a key parameter is the current density. At low current densities, the surface diffusion process is fast compared with electron transfer and both the crystal lattice and structures such as screw dislocations can be well formed and may be observed by electron microscopy. The predominant orientations of surface planes can also be determined using electron diffraction. As the current density is increased, surface diffusion is no longer fast compared with the discharge process and adatoms no longer reach their equilibrium position in the lattice. Furthermore, at the increased overpotential, nucleation of additional growth centres remains a more frequent event. Hence, the lattice 396 Metal finishing (a) (b) (c) (d) Fig. 8.6 Scanning electron micrographs of a copper electrodeposit from an acid sulphate solution at various times, illustrating the development of nodular growth at the limiting current. Nominal deposit thicknesses are as follows. (a) 10 Ilm. (b) 15 Ilm. (c) 20 Ilm. (d) 30 Ilm. (0.07 mol dm ~ 3 CuS0 4 ; I mol dm ~ 3 H 2 S0 4 ; 22°C and with a rotating-cylinder cathode.) (Courtesy: Dr D. R. Gabe, Loughborough University of Technology.) formed will be less ordered and macroscale features, steps, ridges and poly- crystalline block growth (Fig. 8.8) become more likely. With further increase in current density, outward growth of the layer becomes of increasing importance and problems arising from mass transport control in solution can arise. For example, dendrite (Fig. 8.9) (or whisker growth) can occur and once this form of growth commences, it predominates totally because ofthe enhanced rate of mass Electroplating 397 (b) Fig. 8.7 A screw dislocation (a) and its propagation (b) leading to a spiral feature. Note that, as the screw dislocation extends, the kink site is never lost. (b) (d[) o (d) (c) (e) Fig. 8.8 Representation of various growth forms observed in metal deposition. (a) Layer growth. (b) Ridge growth. (c) Block growth. (d) Pyramidal growth. (e) Dendritic growth. 398 Metal finishing Fig. 8.9 Scanning electron micrograph showing dendritic growth of tin during its electrodeposition from a phenol sulphonate solution. (Courtesy: Dr. D. R. Gabe, Lough- borough University of Technology.) transport to the tip (spherical diffusion to the microscale tip) and because the iR drop to the tip is also a minimum as this is the closest point to the anode. In the completely mass transport controlled potential region, the deposit often exhibits a powdered texture. The formation of a roughened deposit is generally cata- strophic for metal-finishing operations. The way in which the structure depends on current density is summarized in Fig. 8.10. The above discussion should not, however, be taken to imply that the best electrodeposits are necessarily formed at the lowest current density; the correct conditions must be sought experimentally. Even when a perfect lattice is Electroplating 399 t H++e- - '/2H2 1 Dispersed powders with increasing oxide or hydroxide content 1·0 Powders (poorly adherent to surface) n+ _ Nodules, dendrites and whiskers M + ne..... M ~--Bunched layers, ridges o L__~~~====~S~pi~ra~ls~,~la~y~e~rs~'Eb~lo~ck~s~(~w~e~lI~fo~r~m~e~dL)~-E Fig. 8.10 The variation of characteristic growth modes with normalized current density f / fL' grown on a substrate with similar lattice dimensions (epitaxial growth), a good adhesive deposit is not always obtained. In many applications, the most durable deposits are often the even, polycrystalline type. The vast majority of electroplating processes are carried out using relatively smooth, direct current (d.c.) power supplies to achieve a constant current flow to the cathode. The useful range of current density which produces satisfactory deposits is much lower than the limiting current density but related to it. Therefore, for a given electrolyte, higher rates of deposition are favoured by increasing I L by the use of, for example: (l) high dissolved-metal concentrations; (2) high temperatures; and (3) relative movement between the cathode and the electrolyte. (1) and (2) are limited in practice. The metal concentration is restricted by solubility, cost (for precious metals) and disposal/effluent treatment considerations. Elevated temperatures can aggravate problems associated with corrosion of process equipment, evaporative losses, chemical decomposition (e.g. of addition agents), prolonged start-up times from cold, not to mention increased power costs for heating. Thus, in practice, there is often considerable scope for considering the cathode-electrolyte flow environment. Quite aside from allow- ing the use of an increased current density and, hence, increased production rate, improved flow may aid the removal of entrapped air or generated hydrogen gas from the workpiece or provide a more stable, local cathode pH and temperature environment. The common methods of introducing electrolyte-electrode movement are: 1. Cathode movement: e.g. reciprocation of a cathode busbar (either horizon- tally, vertically or in both directions), rotation (particularly in the case of cylindrical objects when the current density distribution is time-averaged), or the use of barrel plating (section 8.1.7). A special case is provided by 400 Metal finishing continuous, reel-to-reel plating of wire or strip. This technique is assuming increasing importance in the electronics industry, where rapid and automated processing of components is facilitated by mounting them on a strip. 2. Forced electrolyte flow via air sparging, pumping or jet flow: in certain cases, a special closed cell may be used or the workpiece itself may function as a f1ow- through cell (as in the case of plating the internal surface of cylinders). In a very few, specialized, applications, ultrasonic stimulation of the electrolyte is used. Another specialized method is the use of various alternating current (a. c.) power supplies, the wide spectrum of possible waveforms being referred to as 'pulse' or 'period current reverse' - plating which has been commercially practised since 1965, particularly for certain gold-plating applications in the electronics industry. A general version might include the following sequence: (a) a forward (cathodic) current for a certain 'on' time; (b) a reverse (anodic) current for a certain 'on' time; (c) an 'off' time when no external current is applied; (d) repetition of this sequence. The majority of practical power supplies are (nominally) square wave in form, the technique being applied in a largely empirical fashion. It is clear, however, that periodic current reversal may generally affect charge transfer, mass transport and surface electrocrystallization processes. The effects of pulsed waveforms are extremely complex and poorly under- stood, but the following effects are generally accepted. During the 'off' period of a pulse, no net electron transfer can take place and the cathode surface is refreshed with metal cations as a result of convective diffusion. During the 'on' period, the surface metal ion concentration will initially approach the bulk solution value but will decay with time, i.e. the technique involves non-steady state diffusion. A limiting case is a surface metal ion concentration of zero, i.e. complete mass transport control. The reverse (anodic) current may lead to selective dissolution of high points on the deposit due to their enhanced current density, producing a more compact or smooth surface. The following favourable effects of pulsed current on the deposit quality are generally accepted: higher density, lower porosity, reduced stress, better adhe- sion to the substrate, improved ductility and reduced incidence of H2 embrittle- ment. However, the wider adoption of the technique is hindered by a lack of fundamental knowledge, inconsistent results, and the need for more complex and expensive power supplies. 8.1.3 Anodes The performance of the anode is of considerable importance in determining the success of an electroplating process. Where possible the anode is made from the same metal as that being plated at the cathode and the conditions are selected so Electroplating 401 that the concentration of the metal ion in solution remains constant. This requires the anode and cathode current efficiencies to be matched (usually with values approaching 100%) over the whole service life of the anode. In particular, the electrolytes in the plating bath must be chosen so that the anode does not passivate. A common type of polarization curve for the dissolution of a metal is shown in Fig. 8.11. In the active region, the electrode reaction is the desired metal dissolution but, on increasing the current density or the overpotential above a critical value, the surface becomes covered with an oxide film which passivates the anode, i.e. causes the current for the corrosion reaction to drop to a low level. In a constant-current electrolysis the potential of the anode will shift into the transpassive region where oxygen evolution is a major component of the current. Hence, in a plating process, it is essential to maintain the anode potential in the active region to ensure smooth and uniform dissolution. This may be achieved by using a large anode surface area and, hence, maintaining a suitably low current density at the anode (the anode is frequently in the form of small pellets to increase surface area). Additions of complexing agents, which prevent passiva- tion, or halide ions, which generally cause sufficient pitting of the oxide film for dissolution to continue, are made to many baths. The metal must also dissolve to form the correct oxidation state. Thus, in acid solution, tin may dissolve as tin(n) or tin(lv) but only the former may be reduced to the metal at a potential positive to the hydrogen evolution reaction and therefore the anode current density must be controlled to form only tin(n). The anodes are designed to produce the minimum of anode sludge. This is insoluble solid material which leaves the anode surface and can contain the metal as oxide or hydroxide or even the metal itself if physical disintegration of the anode accompanies dissolution. In addition, impurity elements in the anode which do not dissolve, collect in the sludge. The mode of fabrication and the purity of the anode metal affect its performance, particularly with regard to physical disintegration - rolled metal is frequently better than case metal. For I Active Oxygen dissolution... Passivation evolution ,.. E Fig. 8.11 Typical current-potential curve for an anode in an electroplating bath. 402 Metal finishing several metals, particular forms have been developed for electroplating anodes; examples are 'nickel carbonyl' and 'S-nickel' pellets which combine a high surface area with smooth dissolution and a minimum of sludge. Chromium passivates strongly in acid sulphate media. Hence, an inert anode is always employed in chromium plating. It is generally a lead alloy which immediately covers with lead dioxide on positive polarization in the electrolyte. The alloying elements are tin, antimony and silver which are added to the lead to improve its mechanical properties and to reduce the overpotential for oxygen evolution. In addition to the main anodes, auxiliary anodes of platinized Ti are sometimes also used to improve the current distribution at the cathode. 8.1.4 The plating bath The plating bath is normally a complex mixture of soluble species of the metal being plated, electrolytes and various additives to ensure that the electroplate has the desired properties and quality. In this section we shall consider the role of each of the components. (a) The metal ion The metal to be plated is present in solution either as the simple hydrated ion or as a complex but normally in high concentration, typically 1-3 mol dm - 3. t This high concentration is essential because, while it is necessary to use a reasonable current density, the quality of the plate suffers badly if the deposition occurs under conditions where the electrode reaction is even partially mass-transfer- controlled (Fig. 8.10). A non-complexing medium is frequently used for rapid plating on objects of simple shape while complexing media are employed when a high throwing power is important. (b) Electrolytes Various electrolytes are also added in high concentration to give the bath maximum conductivity. They may also have the role of controlling the pH and buffering the solution particularly if hydrogen or oxygen is evolved at the cathode or anode respectively, since these reactions will tend to change the pH. An increase in pH may lead to deposition of metal hydroxides. This is a particularly difficult problem when the metal depositing is a good H2 evolution catalyst and also easily hydrolysed (e.g. Ni). Hence a Watts bath contains boric acid. A pH above 2 may be essential to decrease H2 evolution, with a consequently reduced tendency for H2 embrittlement or hydride formation in certain metals. t An important exception is in precious-metal baths, where the inventory of metal is kept as low as possible to lower initial investment costs and losses via carryover of process liquor. For example, some gold baths operate at gold levels ~ 10 g dm - 3 Electroplating 403 (c) Complexing agents Complexing agents are used to make the deposition potential more negative when it is necessary to prevent a spontaneous chemical reaction between the cathode and the plating ion, e.g. plating copper onto iron or steel: (8.15) This chemical reaction would lead to a very poor copper deposit, both porous and badly adherent, so a complexing agent is added to make the potential of the Cu2+ jCu couple negative to that for the Fe 2 + jFe couple; reaction (8.15) is then no longer thermodynamically favourable. Complexing agents are also used to modify the Tafel slope for the metal ion reduction and, hence, to improve the throwing power of the bath. The most common complexing agents in electro- plating are cyanide, hydroxide and, more recently, sulphamate ion. Complexing agents also have a role at dissolving anodes; they can prevent passivation and therefore loss of current efficiency in the corrosion reaction and it is for this reason that a low concentration of chloride ion is a common constituent of many baths. (d) Organic additives A wide range of organic molecules are added in relatively low concentration to the electroplating bath to modify the structure, morphology and properties of the cathode deposit. Their development has been almost totally empirical and details of their mode of operation are seldom known. Indeed, it is not always clear whether their effect is due to the additive itself or to decomposition products formed in electrode reactions. Several generalizations concerning their operation are, however, possible. Certainly, additives are usually capable of adsorption on the cathode surface, and in some cases organic matter is occluded into the deposit, especially when the plated metal has a high surface energy (high melting point). Many additives also increase the deposition overpotential and change the Tafel slope. This may be due to the need for electron transfer to occur through the adsorbed layer or due to complex formation at the electrode surface. While the additives may affect more than one property of the deposit ~ and there is clear evidence that when several additives are present in the electrolyte their effect is synergistic ~ they are often considered in the following classifi- cation: 1. Brighteners. For a deposit to be bright, the microscopic roughness of the deposit must be low compared with the wavelength of the incident light so that it is reflected rather than scattered. Brighteners are commonly used in relatively high concentration (several g dm - 3) and may result in substantial organic matter in the deposit. They usually cause the formation of an even, fine-grained deposit and, hence, may act by modification of the nucleation process. 404 Metal finishing For nickel deposition from a Watts bath (a weakly acidic bath containing sulphate and chloride), two types of brightener are recognized: (1) aromatic sulphones or sulphonates leading to bright deposits without lustre and reduced stress; (2) molecules containing -C == N, -N = C = S or )C = 0 entities (e.g. thiourea and coumarin), which produce deposits with a high lustre but also raise the stress and brittleness in the metal. In practice both types are used in combination. The aromatic sui phonates are also brighteners for tin or copper. 2. Levellers. These produce a level deposit on a more macroscopic scale and act by adsorption at points where otherwise there would be rapid deposition of metal. Thus, adsorption of additives occurs preferentially at dislocations because of a higher free energy of adsorption and at peaks because the rate of their diffusion to such points is enhanced; the adsorbed additive will reduce the rate of electron transfer. In practice, many addition agents act as both brighteners and levellers. 3. Structure modifiers. These additives change the structure of the deposit and maybe even the preferred orientation or the type of lattice. Some are used to optimize particular deposit properties, and others to adjust the stress in the deposit (stress is due to lattice misfit). The latter are often called 'stress relievers'. 4. Wetting agents. These are added to accelerate the release of hydrogen gas bubbles from the surface. In their absence, the hydrogen which is often evolved in a parallel reaction to metal deposition can become occluded in the deposit causing, for example, hydrogen embrittlement. 8.1.5 Typical application areas for electroplating Electroplated coatings may confer one or more of' the following surface properties: 1. Corrosion protection. 2. Build-up of material or restoration. 3. Decorative appeal. 4. Wear resistance. 5. Hardness. 6. Optical or thermal reflectivity. 7. Electrical conductivity. 8. Ease of cleaning. 9. Oil retention. 10. Solderability. 11. Thermal conductivity. The usual compromise in choosing the thickness of electroplate is that of obtaining acceptable properties at a minimum, guaranteed thickness. Electro- plating is rarely used for coatings thicker than 75 11m as competitive processes Electroplating 405 (a) (b) Fig. 8.1 2 Examples of 'hard chromium' electroplating for engineering applications. (a) A printing roll; following grinding and polishing, a smooth and highly reflecting surface finish is possible. (b) An injection moulding tool used to produce plastic water tanks (Photographs courtesy: Ionic Surface Treatments; Plating by Hilton and Tuck Division, Manchester.) Table 8.1 Some typical electroplating conditions Current Electrolyte efficiency Metal composition/g dm - 3 TrC l/mA cm- 2 /% Additives Anode Applications eu (a) CuS0 4 (200-250) 20-40 20-50 95-99 Dextrin, gelatin, P-containing Not suitable for iron and H 2S0 4 (25-50) S-containing rolled Cu iron alloy substrates. brighteners, High I but low throwing sulphonic acids power. Additives essential (b) CuCN (40-50) 40-70 10-40 60-90 Na 2S03 02-free, high- Good throwing power, KCN (20-30) conductivity Cu deposits adhere well and K 2C0 3 (10) are bright. Used as undercoat. Cyanide decomposes at anode Ni (a) NiS0 4 (250) 40-70 20-50 95 Coumarin, saccharin, Ni pellets or H2 evolved with de- NiCl 2 (45) benzenesulphonamide pieces position. Additives H 3B0 3 (30) acetylene derivatives depend on purpose pH 4-5 of deposit. Medium throwing power (b) Ni sulphamate 50-60 50-400 98 Not essential. Ni pellets or Good throwing power. (600} naphthalene-1,3,6- pieces Some H2 with additives, NiCl 2 (5) trisulphonic acid mirror finish at H 3 B0 3 (40) 400mAcm- 2 pH 4 Ag KAg(CN)2 (40-60) 20-30 3-10 99 S-containing Ag Good throwing power. KCN (80-100) brighteners Functional or decorative K 2C0 3 (10) Sn (a) SnS0 4 (40-60) 20-30 10-30 90-95 Phenol, cresol Sn Poor throwing power. H 2 S0 4 (100-200) Additives essential (b) Na 2 Sn0 3 (50-100) 60-80 10-20 70 Sn (control I) Good throwing power NaOH (8-18) but uses twice charge (Sn(Iv) rather than Sn(n)) (c) SnCI 2 (75) 65 50-200 90 SCN -, disulphono- Sn Reasonable throwing NaF (37) naphthalinic acid power. More stable KF (50) than (a). Rarely used. NaCl (45) pH 2.5 Zn (a) NH 4 ZnCI 3 25 10-40 98 Dextrin, organic Zn Poor throwing power. (200-300) additives Additives essential NH 4 CI (60-120) pH5 (b) ZnO (20-40) 15-30 10-50 70-90 Glycerin, organic Zn Good throwing power NaCN (60-120) additives NaOH (60-100) (c) ZnO (6-10) 20-40 10-30 60-80 Organic additives Zn Medium throwing NaOH (70-100) power. Non-toxic. Additives essential Cr (a) Cr0 3 (450) 45-60 100-200 8-12 Complex fluorides to Pb-Sb or Pb-Sn Need to reoxidise Cr 3 + H 2 S0 4 (4) increase efficiency coated with formed. Decorative Pb0 2 finish. Poor throwing power (b) Cr0 3 (200) 40-55 100-200 10-15 Pb-Sb or Pb-Sn Hard, wear-resistant H 2 S0 4 (2) coated with deposit Pb0 2 408 Metal finishing may be faster and cheaper. Exceptions do exist however, particularly in heavy engineering; e.g. chromium plating (Fig. 8.12) may be carried out up to perhaps 500 or even 1000 11m thickness because alternative methods of coating are not available. Such heavy deposits require considerable skill and effective post- plating operations such as grinding and honing in order to improve the surface finish. The likelihood of an unacceptable porosity increases markedly for thin coatings, and this may be catastrophic for noble coatings (as noted in Chapter 10), such as copper on steel. The upper limit on deposit thickness is influenced by such factors as an excessive process time, too high an internal stress or development of irregular deposit growth, e.g. nodules or dendrites (Figs. 8.8-8.10). A particular application will require its own deposit characteristics (including thickness) and, hence, a different bath formulation and electrolysis conditions. Table 8.1 therefore sets out some typical plating conditions for six metals which are extensively electroplated. For several metals there is a choice of an acidic, non-complexing bath which is used for rapid plating of simple shapes (e.g. flat surfaces and wires) and a complexing medium which gives a lower plating rate but the additional throwing power essential for intricate surfaces. Moreover, only a range is given for several parameters since the value will depend on the deposit properties sought. From Table 8.1 it can be seen that most plating processes operate at a current density between 10 and 70 mA cm - 2, a range which is low compared with that for the preparative electrolytic processes. Since, in general, the thickness of the deposit required will range between 0.01 and 100 11m, depending on the application, the electroplating procedure will last from only a few seconds to perhaps several hours. The current density for silver deposition is particularly low while those for nickel in sulphamate media and tin in the chloride or fluoride bath are markedly higher. The value for chromium is deceptive because of the low current efficiency; most of the current is hydrogen evolution. For most metals (chromium being an exception), the table also shows that the current efficiencies are good and that the use of additives and temperatures slightly above ambient is normal. The plating of some alloys is possible although the limitations are greater and the control of the conditions more critical. It is essential to use a complexing bath selected to make the deposition potentials of the components of the alloy the same, although the J-E curves for the individual metals are not always good guides for suitable baths. The alloy deposited clearly depends on the electrolyte, bath temperature, current density and the ratio of the metal ions in solution. The latter is often critical, the exceptions being deposits which are intermetallic compounds (e.g. SnNi) although the deposit composition is not the same as that of the solution. Also, the current densities are towards the low end of the metal- plating range and many of the alloys do not dissolve anodically so that it is necessary to use anodes of both metals. The conditions for plating four alloys are summarized in Table 8.2. Table 8.2 Typical conditions for plating alloys Current Electrolyte efficiencyI Alloy composition/g dm - 3 TrC limA cm- 2 % Anode Comments 70% Cu-30% Zn K2Cu(CNh (45) 40--50 5-10 60--80 Brass Additives essentiaL Close control (brass) K 2Zn(CN)4 (50) of bath + plating conditions KCN (12) essential Sodium tartrate (60) 40% Sn-60% Cu K 2Cu(CNh (40) 60--70 20--50 70--90 Bronze or mixture Additives essential (bronze) Na 2Sn0 3 (45) of Sn and Cu NaOH (12) anodes KCN (14) 65% Sn-35% Ni NiCl 2 (250) 60--70 10--30 97 Separate Ni and Good throwing power. Deposit SnCl 2 (50) Sn plates composition not dependent on NH 4F· HF (40) electrolysis conditions over a NH 40H (30) wide range 80% Ni-20% Fe NiS0 4 (300) 50--70 20--50 90 Ni + Fe as pieces Close control of [Ni2+]/[Fe2+] FeS0 4 (20) in bath essential H 3 B0 3 (45) NaCl (30) pH 3-4 410 Metal finishing In the mechanical engineering sectors of industry, electrodeposition has a well- established position as a coating technique (Table 8.3), the following advantages being important: 1. Operating temperatures generally lie in the range 20-70° C; thus, extreme temperatures (which may lead to distortion or adverse metallurgical changes) are avoided. 2. Under suitable conditions, the coating adhesion is very strong. 3. In many cases, a relatively thin coating produces a radically improved surface, with respect to its engineering performance. 4. Process automation is often possible. Limitations include: 1. The speed of deposition is usually much lower than 75 Ilm h -1 (although forced electrolyte convection may improve this). 2. The physical size of the plating bath may limit dimensions of the workpiece. 3. In some cases, the plating bath (or pretreatment liquors) may be toxic and require adequate treatment before discharge as effluent. The plating of composites is a recent development but one which is likely to be of increasing importance because it is found that the inclusion of solid particles into the metal deposit can greatly enhance the wear resistance and friction properties of the deposits. Solids such as A1 2 0 3 , Ti0 2 , Si0 2 , WC, TiC, SiC, Cr 3 N 2 , diamond, graphite, MoS 2 and PTFE, present in solution as particles 0.5-5 Ilm in diameter, are entrapped in a deposit under suitable electroplating conditions. They are usually present in 2-10 vol % and are codeposited by one or both ofthe following mechanisms: 1. Physical dispersion in the electrolyte and adequate agitation facilitates mechanical entrapment; special pumped electrolyte flow and plate plungers have been used. 2. The particles are dispersed in the electrolyte and electrically charged, courtesy of surfactant adsorption; electromigration can then occur towards the cathode; usually a combination of non-ionic and cationic surfactants are used. The applications for hard particle composites lie, for example, in internal combustion engines, textile machinery and gas turbine compressors, where combined properties can include resistance to wear, fretting, scuffing and heat and chemical attack. The softer particles display an advantageously low coefficient of friction and effective self-lubricating properties. 8.1.6 Electroplating in the electronics industry The applications of electroplating are legion within the electronics industry and the technique is used on a scale ranging from the minute, for the lead-in Electroplating 411 Table 8.3 Typical engineering applications for electroplated metals Deposited Typical Characteristics metal hardness/HV of coating Typical applications Cr 800-1000 Hard and wear-resistant; Reclamation of worn or low friction and non-stick; oversize components; rolls corrosion-resistant; brittle for printing or steel rolling; plastic moulds; hydraulic rams Ni 150-450 Softer; more ductile than Reclamation of worn or Cr; corrosion-, heat-, and oversize parts; food pro- wear- resistant; solderable; cessing machinery; electro- can be machined by forming; hydraulics cutting tools Cu 60-150 Softer than Ni; ductile; As a resist for selective case resistant to fretting; good hardening of steel; electro- electrical and thermal forming; surface lubrication in conductivity metal working Fe 150-350 Low cost; tough and easily Anti-scuffing in internal heat treated; poor corrosion combustion engines; resistance soldering iron tips; reclamation of worn parts; electroforming of foil Sn 8-12 Soft and ductile; low melting Overlay coatings for pistons point; resistant to fretting, in internal combustion scuffing and adhesive wear engines; corrosion protection Ag 60-120 Excellent electrical Specialized anti-seizure of conductivity; resistant threaded parts at high to fretting temperature 65 Sn- 650-700 Hard; low friction, retains Hydraulic braking systems 35 Ni oil film, corrosion resistant; in automobile engineering brittle; temperature limit ="= 360 C 0 conductors in large-scale integration (LSI) chips, to a wide variety of printed circuit boards used in the majority of electronic hardware. Alternative methods of metal deposition (including vacuum deposition or conductive paints and lacquers) have their niche but electroplating has provided the most favourable route, especially for large-scale processing. In general, the electrolytes and electroplating conditions used are similar to those for decorative or engineering 412 Metal finishing plating. The critical and ever-increasing performance of both printed circuit boards and components demanded by the electronics industry means that cleanliness and process control are much more stringent. The choice of metal depends on the nature of the component or device, service conditions, cost and required lifetime. Copper, silver and gold are primarily selected for their high electrical conductivity. Palladium and rhodium find use where hardness and enhanced corrosion resistance are important. Tin and tin-lead are utilized for their low melting points and solderability (tin-nickel deposits are also becoming increasingly important in this area). Typical application areas of the metals are given in Table 8.4. In order to illustrate the utilization of electroplating in the electronics industry, two areas may be briefly described: (1) fabrication of printed circuit boards; and (2) electri- cal contacts. (a) Printed circuit boards The major technique used to manufacture printed circuit boards is the 'sub- tractive' method, where the desired pattern of copper tracking is formed by etching away unwanted copper (Chapter 9) from the glass fibre/epoxy (or phenolic) base. The base ranges from thick « 5 mm), rigid boards for heavy power circuitry and components down to thin ( < 0.15 mm) flexible materials. The adoption of double-sided, copper clad laminates together with plated- through-holes permits a much higher packing density of components. A circuit pattern is produced via etching on both sides of the board followed by interconnection of the two sides via plated-through-hole fabrication. It may be noted that the printed circuit board laminate is produced by using electroformed copper foil, nodular deposition of copper and surface anodic oxidation to Cu 2 0 providing greatly improved bonding to the insulating substrate. More sophisticated circuits employ multiple layers of copper tracking, interconnected by through-hole-plating. Figure 8.13 shows schematically the steps involved in fabrication: etching, electroplating, electroless plating (section 8.2) and, occasionally, immersion plating are all involved, on an integral basis with mechanical, physical and optical techniques. The manufacturer of printed circuit boards requires plating-through-holes; ideally, the electroplated layer should have a uniform thickness within the hole and on the flat laminate 'land' areas; this presents a challenge to the throwing power of the electrolyte (section 8.1), the racking of the printed circuit board and effective control over the electroplating process. (b) Electrical contacts and connectors The electronics industry relies upon effective contacts for the connection of: I. Semiconductor wafer-to-chip connection. 2. Chip to package. Electroplating 413 Table 8.4 Typical application areas and special properties of electroplated metals used in the electronics industry Metal Special or alloy characteristics Application areas Electrolyte type Ag High electrical Heavy-current busbars Alkaline cyanide conductivity; and tracks; low contact switchgear; fuse holders; resistance cable Cu Relatively low cost; Printed circuit boards Acid sulphate; good electrical alkaline cyanide; conductivity pyrophosphate Au Corrosion resistance; Printed circuit board Neutral cyanide; high electrical edge connectors; acid cyanide; conductivity; reed relays; sulphite low contact resistance semiconductor lead-out connections Rh Very hard, but can be Open contacts Acid sulphate highly stressed Pd Hard; Heavy-duty open con- Sulphamate; corrosion resistant tacts; halide; diamminodinitrite; ammino Sn Solderability; reason- Pretinning of contacts; Acid flu oro borate; able contact resistance electrical contacts acid sulphate 60:40 Solderability Printed circuit board Acid fluoroborate Sn-Pb tracks 65:35 Solderability superior Printed circuit Acid chloride Sn-Ni to Sn-Pb, especially board tracks after prolonged storage 80:20 Resistance to Sliding wear contacts Ammine sulphate Pd-Ni sliding wear; lower cost than Au Ru Good wear resistance; Reed switches Nitrosyl sulphamate oxide is electrically conducting 414 Metal finishing :;;;;;;;::;;:;:;;:;;::;:;::;:;::;:;:;:;:;;;._ Copper foil /:/// h--)// / / / / / / / / / / / / //._ Insularing base /:///////////////////// (GRP/epoxy or phenolic) ' - Copper foil ~ Erching of copper ro produce rracking t Drilling of t"hrough-holes I Adival"ion of surfaces rhen elecrroless t copper plating ro metallize rhe rhrough hole I Copper elect-roplahng ro build up deposit" t rhickness, followed in some cases by Sn-Pb plating Fig. 8.13 The steps involved in the subtractive technique for the manufacture of printed circuit boards. A very simplified schedule is shown to obtain a double-sided board, i.e. one which has copper tracks on each side interconnected by 'plated-through' holes. 3. Package to substrate or printed circuit board. 4. One board to another or to the rest of the circuitry. 5. Switches, which may be of open or sealed construction. In the case of printed circuit boards, edge connectors are required at the extremities of the panel for interboard connections. The connectors are of the mechanical pressure type, due to the need for intermittant board replacement. Gold is deposited either directly onto the copper pad or an intermediate, electroplated nickel layer (which provides a diffusion barrier). 'Acid' gold cyanide is a common electrolyte, the deposit containing small amounts of cobalt to provide hardness. For contact applications, the surface preparation is often critical and the co- depositions of other materials are often of paramount importance to the reliability and service life. For many applications, especially those involving high currents, Electroplating 415 the bulk contact is fabricated from copper or a copper alloy. In an open atmosphere, corrosion protection, wear/spark resistance and effective electrical conductivity are provided by a number of precious-metal deposits. Its high electrical conductivity finds silver numerous low-noise or high-current appli- cations, particularly in plug and socket arrangements, switchgear, fuseholders, and copper cable. Gold and its alloys (pure gold is soft and costly) have been traditional choices, due to their favourable combinations of electrical conduc- tivity together with corrosion and wear resistance. The selection and adoption of alternative methods have proved difficult but attract increasing attention. Palladium and rhodium layers provide considerably enhanced wear resistance, while palladium-nickel has introduced a cheaper alternative to gold. A common form of closed contact is the reed relay, where the sealed capsule consists of two Ni-Fe blades, coated in the contact area with a precious metal and sealed into a glass envelope filled with, for example, a N 2 -H 2 mixture. The capsule is positioned within, or close to, a coil which induces the blades to contact magnetically when energized. The blades are usually gold-plated using a continuous belt-feed system, the cathode contact area being limited by judicious control of electrolyte level. A wide range of applications are found in semiconductor manufacture, e.g.: 1. In transistors, the base ofthe encapsulating can and lead wires are gold plated. 2. Integrated circuits (ICs) are generally packaged as discrete integrated pack- ages (DIPs) or flat packs ('cerpaks'). The basic conductor pattern is a Mo-Mn alloy coating on a Ni-Fe (Kovar) lead frame. Gold is electrodeposited (~1 11m thick) onto this substrate (usually from a neutral cyanide solution) to reinforce the conductor pattern and provide improved conductivity. The brazed ceramic base is gold plated with 0.5 to 111m pure gold, while the external leads may be electroplated with tin or tin-lead to improve solderability. There remain several problematic factors in the development of palladium electrodeposits. Useful current densities (and hence deposition rates) are low, typically < 50 rnA cm - 2; baths have tended to show chemical instability and etching of base metal substrates. Moreover, evolution of hydrogen and its incorporation into the metal lattice, a facile process on active Pd, may result in f3- PdH 2 producing high stress levels in the deposit. Whilst problematic in electroplating, hydrogen absorption in palladium provides an effective method for the sensing of hydrogen gas (Chapter 12). Palladium surfaces are also prone to adsorption of organic contaminants, leading to catalysis of oxidation/reduction and, subsequent polymerization, in service, leading to unreliable contact resistance. 8.1.7 The overall plating process and the importance of component design It is essential for the surface to be correctly prepared in order to obtain a good electrodeposit. A typical simple procedure will have the following steps. Electroplating 417 In barrel plating, the components are usually weighed before loading; deposit uniformity is usually assured due to the random orientation of components with respect to the anode. This technique is carried out on a wide range of scales and levels of automation (Fig. 8.16). The electroplating step is normally carried out in a simple rectangular tank of volume between 20 and 2000 dm 3 , which should be thermally insulated and have provision for heating the electrolyte. It is also frequently advantageous to introduce convection into the plating tank and, where oxygen does not interfere with the metal deposition, bubbling air through the electrode is the cheapest procedure. The jigs are mounted in the tank with anodes, in bags of cotton or plastic to retain anode sludge, placed symmetrically about the cathode jig; the positioning of the jigs, main and auxiliary anodes may be based on experience or a detailed computer optimization depending on the cost of the product and the scale of the operation. In larger facilities, the tanks will have a loop for continuous filtering of the electrolyte and the bath temperature, pH and current will be monitored. The concentration of metal ions will be determined by external analysis on a regular basis. The most modern plants will also have an effluent-control plant to deal with liquids resulting from washing and spillages and sludges removed from the tanks. The heavy metals are still cbmmonly precipitated with caustic soda and the solids then allowed to settle, although the modern trend is towards direct electrolytic metal recovery from the effluent (Chapter 7). The control units for the electroplating baths generally operate at a current between 100 and 10000 A and a voltage of - 8 to -12 v. There are presently very few electroplating processes which utilize a divided cell, primarily due to the increased cell voltage (and, hence, power costs), constructional complexity and increased maintenance requirements. One exam- ple is the 'Envirochrome' process for decorative chromium plating which has been trial-manufactured during the last five years. This utilizes a Cr{m) electrolyte which avoids problems associated with the use and disposal of Cr(vI) solutions. It is important to design the workpiece, wherever possible, to minimize the degree of masking required in those areas which must not be plated. In addition, care should be taken to avoid recesses which may serve to trap cleaning or plating liquors. Another important consideration is electrical conductivity; the workpiece should, when possible, have continuous conductivity across any welds, joins, etc. In general, irregularly shaped objects cause many problems, particularly if they feature sharp edges, abrupt changes in cross-section, per- forations, recesses or box sections. The problems arise particularly from poor current density distribution and gas entrapment. Figure 8.18 illustrates some problems associated with the geometry of the substrate, and low throwing power of, for example, a chromium electrolyte, together with suggested solutions. Whenever feasible, the design engineer should consider likely problems in the electroplating step during the early stages in the 418 Metal finishing design of the component. Failure in this respect inevitably results in a tedious, difficult (even impossible), unsatisfactory or expensive electroplating process. Any special preparation or replating procedures applied by the electroplater undoubtedly appear as increased overheads for the electroplating operation. (a) (b) Fig. 8.14 Jig-rack mounting of electroplated articles. (a) A jig for nickel-plating the interiors and exteriors of kettle bodies. Note the use of platinized titanium auxiliary anodes inside the kettle to provide improved current distribution. (Courtesy: W. Canning Materials Ltd.) (b) A transported-serviced electroplating system, which may be semi- or fully automatic in operation and which utilizes rack- or jig-mounting of components. (Courtesy: Electroloid Ltd.) 420 Metal finishing Fig. 8.15 A facility for manual electroplating. The modular equipment is suitable for both rack- and barrel-plating. Construction materials include corrosion-resistant PVC- clad steel and stainless steel. Applications include precious-metal plating in the electro- nics industry. Power supply and control circuitry are located above the tanks. (Courtesy: PMD Chemicals Ltd.) Of the many modern developments in electroplating technology, several trends may be highlighted. 1. Electrodeposition of alloys having a controlled composition (e.g. Ni-Fe or Pd-Ni) as cheaper alternatives to single metal coatings. 2. Codeposition of metals with either polymers ('polymets') or ceramics (,cermets') to provide unique wear-resistant or 'tribomet' coatings, e.g. Ni-PTFE, Ni-diamond, Co-carbides. 3. Increasing consideration of less toxic plating baths; in particular cyanide solutions for gold and cadmium have partly been replaced by non-cyanide liquors. 4. Electroless (zero current) plating of an increasingly wide range of single metals, alloys and composites, e.g. Ni-PTFE (section 8.2). 5. Continued development of non-aqueous baths, particularly for aluminium t and titanium. (Baths for Ta and Nb have also been developed.) T Commercial aluminium plating processes, based on aluminium triethyl at 80-100 C 0 are currently in operation on a production scale (up to 2200 dm 3 electrolyte volume), e.g. the Sigal process (Hegin Galvano-Aluminium bv). Electroplating 421 (a) (b) Fig. 8.16 Equipment for 'barrel-plating' small components. (a) A range of small electro- plating barrels available in polypropylene (or Perspex) having 2.4 mm perforations as standard. (Courtesy: PMD Chemicals Ltd.) (b) A fully automatic vibrating barrel for bulk plating of numerous small parts such as electrode contacts which are too delicate for barrel plating. (Courtesy: Electroloid Ltd.) 422 Metal finishing 8 Lead anode\ Catholyte Inner perforated Outer perforated baske~ side basket side Ion exchange membrane (a) Cation exchange membrane 8 I I Crill _ I I I ~H+ I I + I H_ I iCcr ill An0.g e + Cathode 2 H2 0-4e - 0 2 + 4H Crm + 3e- ____.Cr 2H++2e--H 2 (b) Fig. 8.17 A divided cell for decorative chromium electroplating (the Envirochrome process). (a) The cell design. (b) The electrode reactions. (Courtesy: W. Canning Materials Ltd and IBM UK.) 6. The use of programmed current waveforms (e.g. periodic current reversal) in order to produce thin, dense, pore-free coatings, particularly for demanding electronic applications (section 8.1.2). 7. Selective, high-speed, automated electroplating processes for reel-to-reel plating (Fig. 8.19) of electronics materials, e.g. by use of laser-stimulated deposition. Electroplating 423 ~ Deposit Workpiece ~ ;?/Conforming (cathode) ~QOOde 'shields' rM ~ Insu Ia h ng _______ (i) c:::=::=J = (ii) ~ liT r ~ Aoooe ~Conducting c:::=::=J (iii) =(iv) 'ro b bers J Fig. 8.18 Modification of extreme current densities to provide a more uniform current distribution. (i) use of a radiused corner to reduce throwing power deficiency in a recess; (ii) use of a conforming anode to overcome poor throwing power in a recess; (iii) use of inert shields to prevent build-up of deposits on edges; and (iv) use of cathodic 'burners' or 'robbers' to minimize edge build-up. (After: Ashby (1982) Electroplating for Engineering Technicians, National Physical Laboratory, London; and Courtesy: Ionic Plating Ltd.) Fig. 8.19 A continuous reel-to-reel electroplating line. The machine is a four-strand device which incorporates pretreatment sections prior to electroplating. A typical application is the selective electroplating of gold spots onto nickel~iron lead frames, which is an important stage in the manufacture of integrated-circuit packages. (Courtesy: S. G. Owen (Northampton) Ltd.) 424 Metal finishing Table 8.5 Pros and cons of electrode position as a coating technique Advantages Disadvantages Control of deposit thickness good Only some metals may be deposited from aqueous solution Control of deposit distribution fair Rate of deposition limited to 20-100 J..lmh- 1 Variety of finishes possible Thickness ranges limited in practice, e.g. < 1 J..lm, too porous; > 70 J..lm, too expensive Technology readily available at reason- Some substrates require special pretreat- able cost and well-understood ment Most metals depositable Throwing power variable with, for ex- ample, metal, electrolyte and process conditions Most substrates coatable Deposits less porous, at equivalent thick- ness, than many competitive techniques In the light of the above, it is possible to summarize some advantages and drawbacks of electrodeposition as a coating technology (Table 8.5). It may be concluded that electroplating is vital in almost every branch of industry. The technique often provides a versatile and economic method of applying a thin (say 1-75 J..lm) metal coating which significantly improves the electrical or decora- tive properties of the substrate. 8.2 ELECTROLESS PLATING 8.2.1 Fundamentals of electrochemical deposition It is important to distinguish between three types of electrochemical process for the deposition of metals: (1) electroplating; (2) immersion plating; and (3) e1ec- troless plating (Table 8.6). They differ in the nature of their anodic reaction. Considering the simple case of discharge of a metal ion, the common cathodic process is given in equation (8.1) as M n + + ne - ----> M. This is supported by a suitable anodic process (taking place at the same rate). 1. In electroplating dissolution of a separate anode, Ma occurs: MA - ne- ----> M~t (8.16) Electroless plating 425 Table 8.6 Comparison of electrochemical methods for metal deposition Electroless Immersion Property Electroplating deposition plating Driving force Power supply Autocatalytic redox Chemical displacement reaction Cathode reaction M" + + ne - -------> M M" + + ne - -------> M M" + + ne - -------> M Anode reaction M" - ne - -------> M R - ne - -------> 0 M 1 - ne - -------> M~ + or n/2 H 2 0 - ne - -------> n/40 2+nH+ Overall cell MA ------> Me or M"+ +R------->M+O M" + + M 1 -------> reaction n/2 H 2 O+M"+-------> M+M~+ n/4 02+nH+ +M Site of cathode Substrate (work- Substrate (work- Substrate (workpiece) reaction piece) piece) which must which must remain have a catalytic partially exposed surface Site of anode Separate anode Substrate (workpiece) Substrate (workpiece) reaction which dissolves Anode reactant M or H 2 O R, reducing agent in M I , dissolving metal solution Nature of Pure metal (or Usually M Pure metal, but may deposit definite alloy) contaminated by be porous and poorly O/R derived species adherent Thickness 1-100 1-100 ~10 limit/J..lm (or oxygen evolution takes place). The overall cell reaction is then: MA----->Me (8.17) In an ideal case, metal is simply transferred from anode to cathode. 2. In immersion deposition, simple displacement occurs as the substrate metal N dissolves: (8.18) and M will deposit onto M l' often in a porous and poorly adherent manner, at 426 Metal finishing a declining rate; the substrate metal, eventually will no longer be exposed to the electrolyte and deposition ceases. 3. In electroless deposition processes, the oxidation of a soluble reducing agent R R-ne- ----->0 (8.19) occurs on some sites on the substrate surface such that the overall process is: Mn+ +R----->M+O (8.20) The deposition of the metal is autocatalytic; once nucleation has occurred, further deposition is a very favourable process which occurs at a fast rate on the growing deposit. The electroless process can be traced back to laboratory observations by Wurtz in 1844. Despite research by other workers in 1911 and 1931, industrial developments did not begin until 1946, when Brenner and Ridell devised an acid electroless nickel bath. Today, the majority of electroplated metals have been deposited successfully from e1ectroless baths, but fundamental knowledge of the underlying chemical and electrochemical reactions is at an early stage. More- over, considerable skill and research work is often necessary to formulate a practical electroless plating bath. Thermodynamically favourable but unwanted dropout of the metal in the bulk electrolyte or the plating tank walls is always a danger; the bath must deposit the metal at a high rate, but selectively onto the substrate! By far the most important examples of electroless deposition in industry are deposition of: 1. Nickel onto steel substrates, largely for engineering applications, but also for uses in the electronics industry. 2. Copper onto printed circuit boards and plastics (section 8.1.6) (following surface activation). These applications will be described in section 8.2.3, but first, the formulation and composition of electro less plating baths will be considered. 8.2.2 Composition of electroless plating baths An electroless plating liquor in general will contain the following components: (1) a source of soluble, electroactive metal; (2) a reducing agent; (3) a complexant; (4) an exaltant, which may help to increase the rate of plating; (5) stabilizers to prevent bath decomposition; (6) other addition agents; and (7) a buffer to control pH. Typical examples of electrolytes and operating conditions are given in Table 8.7. Taking electroless nickel plating as an illustrative example, the reducing agent is often hypo phosphite; the process may be represented by the much simplified Electroless plating 427 Table 8.7 Composition and operating conditions for selected electroless plating processes Bath/Deposit Formulation Acid nickel Nickel chloride 20 gdm- 3 Sodium hypophosphite 20 gdm- 3 Sodium acetate 10 gdm- 3 Sodium succinate 15 gdm- 3 pH 4.5 Temperature 93° C Alkaline nickel Nickel chloride 30 gdm- 3 Sodium hypophosphite lOgdm- 3 Ammonium citrate 65 gdm- 3 Ammonium chloride 50gdm- 3 pH 8-10 Temperature 90° C Alkaline cobalt Cobalt chloride 30 gdm- 3 Sodium hypophosphite 30 gdm- 3 Sodium citrate 50gdm- 3 Ammonium chloride 40gdm- 3 pH 8.5 Temperature 90° C Copper Copper sulphate 12gdm- 3 Formaldehyde 8 gdm- 3 Sodium hydroxide 15 gdm- 3 Rochelle salt 14gdm- 3 EDTA 20 gdm- 3 pH 11 Temperature 25° C reactions: Cathode Ni2+ +2e- ------.Ni E" -0.25 V (S.21) Anode E"= -0.50 V (S.22) Overall Ni2+ +H2POi + H 20------.Ni+H 2P0 3 +2H+ cell LlG= -4S.3kJmol- 1 (S.23) which take place on a single surface. Several general points may be made. 1. The substrate metal (after surface preparation) and the deposited metal must support both reactions (S.21) and (S.22) for the electroless process to occur. 2. Substrates may be active upon immersion, or they may briefly be stimulated cathodically by, for example: (a) contact with a workpiece which is already plating; (b) an impressed current, courtesy of an external battery or power supply. 428 Metal finishing 3. A pH decrease accompanies the overall cell reaction as in equation (8.23). 4. The reducing agent is depleted and its oxidation product accumulates. 5. The source of metal declines in concentration. The exact mechanisms of the chemical and electrochemical reactions occurring during electroless plating are still obscure. In the case of electroless nickel, they are far more complex than those suggested by reactions (8.21) add (8.22). Other reactions are possible besides the formation of a metallic coating. For example, the hypophosphite bath can lead to the formation of phosphorus, resulting in a Ni-P alloy deposit. The amount of phosphorous codeposition varies between 3% and 15%. When borohydride is used as the reducing agent, boron is incorporated in the deposit, while no codeposition is observed when hydrazine or formaldehyde are part of electroless formulations. Hydrazine is not very popular as a reducing agent owing to its potentially explosive nature and recent reports suggest that it may be carCInogenIc. The selection and concentration of the complexant must be considered very carefully, because if the metal is too-heavily complexed, insufficient free metal ions will be available for deposition. For instance EDT A, which has a high stability constant, requires very careful control if plating is not to cease. As reactions in the bath involve the formation of either protons or hydroxyl ions, the pH of the solution will alter during plating and thus affect the rate of deposition and composition of the deposit (section 8.2.3 and 8.2.4). Buffers have to be added to stabilize the pH of the solution, and these include the carboxylic acids in acid media (which also act as complexants) and ammonia/ammonium chloride or organic amines in alkaline solutions. Electroless plating formulations are inherently unstable and the presence of active nuclei such as dust or metallic particles can lead over a period of time to a process analogous to homogeneous reduction. Stabilizers, which adsorb onto these active nuclei and shield them from the solution, are added in small concentrations to overcome this problem. Typical examples are lead, calcium or thallium ions, thiourea and mercaptobenzthiazole. However, if they are used in excess, deposition will be completely prevented even on the substrate itself. The rate of plating may be lowered drastically by the addition of complexants to the formulation. Additives which increase the rate to an acceptable level without causing bath instability are termed 'exaltants'. These are generally anions which are thought to function by catalysing oxidation of the anodic process. Common examples include sodium succinate, glycine and fluoride ions. 8.2.3 Operating conditions The successful operation of an electroless process unfortunately does not end with the selection of the correct ingredients for the bath. The rate of deposition is markedly affected by temperature. This is clearly shown by the acid electroless Electroless plating 429 nickel formulation given in Table 8.7. Plating does not commence until a temperature of 70° C is reached. A further rise in temperature causes a dramatic increase in deposition rate with a value of over 20 11m h - 1 being obtained at 92° C. Temperatures above this value will make the bath very unstable and may cause metal deposition on the jigs and the walls of the tanks, or complete homogeneous reduction of the solution. The practical consequence of this experimental observation is that large objects to be coated have to be preheated to the operating temperature prior to immersion. This necessitates the use of accurate temperature controllers if consistent coating thicknesses are to be achieved without resorting to the use of test coupons in the bath for each batch of components plated. Current research is aimed at reducing the operating temperatures of the baths, and at present electroless copper baths for the printed- circuit-board industry are operated near room temperature. As already stated, the pH of the solution alters the potential difference bet