Enzyme Mechanisms PDF
Document Details
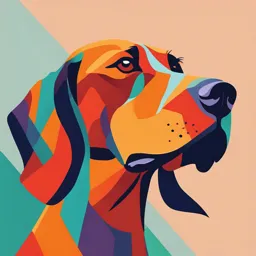
Uploaded by StunningParadox9359
null
null
null
Tags
Summary
These lecture notes provide a summary of enzyme mechanisms, covering various catalytic strategies, including covalent catalysis, general acid-base catalysis, metal ion catalysis, and catalysis by approximation.
Full Transcript
Enzyme mechanisms All figures not specifically referenced either come from: J. M. Berg et al., Biochemistry 7 th edition. W. H. Freeman and Company, 2010. J. M. Berg et al., Biochemistry 8 th edition. W. H. Freeman and Company, 2018. Figures from: J. M. Berg et al., Biochemistry 10 th edition,...
Enzyme mechanisms All figures not specifically referenced either come from: J. M. Berg et al., Biochemistry 7 th edition. W. H. Freeman and Company, 2010. J. M. Berg et al., Biochemistry 8 th edition. W. H. Freeman and Company, 2018. Figures from: J. M. Berg et al., Biochemistry 10 th edition, W.H. Freeman MacMillan Learning, 2023 are specified Many figures come from A.L. Lehninger, Principles of Biochemistry, 6 th edition. W.H. Freeman, 2013, Permission granted to use all these figures Due to copyright laws, you CANNOT POST NOR DISTRIBUTE these lecture notes or any course material. They can only be used for personal educational purposes pertaining to this course. 1 Enzymes use core catalytic strategies to catalyze a specific reaction Binding energy: The free energy released in the formation of a large number of weak interactions between the enzyme and the substrate facilitates the formation of the transition state (lowers its energy, stabilizes it) Induced fit: The binding energy promotes the structural changes that occur upon substrate binding into the active site. These changes facilitate the formation of the transition state and promote catalysis. Purpose of binding energy: 1. establishes substrate specificity 2. increases catalytic efficiency Text adapted on Aug. 20, 2024: Credit: "Enzymes" by Bio-OER, CC BY-NC-SA, via LibreTexts: https://bio.libretexts.org/Bookshelves/Biotechnology/Bio- 2 In addition to binging energy, enzymes use one or more of the following catalytic strategies 1. Covalent catalysis: The active site contains a reactive group (usually a nucleophile) that is briefly covalently modified (attached to the substrate) during catalysis. Example: Chymotrypsin 2. General acid-base catalysis: Proton transfers mediated by weak acid/weak base OTHER THAN WATER Usually Asp, Glu, His, Arg or Lys. The pKa’s of the active site residues are often optimized for a specific protonation or deprotonation event. Example: Chymotrypsin Nucleophile: - attracted to a positively charged centre - it donates electrons Electrophile: - attracted to a negatively charged centre - it accepts electrons 3 In addition to binging energy, enzymes use one or more of the following catalytic strategies 3. Metal ion catalysis: Metal ions function in a number of ways including i. Facilitating the formation of the nucleophile by direct coordination ii. Stabilizing a negative charge in an intermediate (acts as an electrophile) iii. Acting as a bridge between substrate and enzyme holding the substrate in a conformation necessary for catalysis Example: Carbonic anhydrase 4. Catalysis by approximation: The enzyme brings two substrates together along a single binding surface of the enzyme in an orientation that facilitates catalysis. Example: Carbonic anhydrase 4 Proteases Proteases are enzymes that cleave peptide bonds in a protein (or protein-like substrate) by hydrolysis. Although protein hydrolysis is exergonic (thermodynamically favorable), it is kinetically very slow. The planarity of the peptide bond accounts for the resistance to hydrolysis. Enzymes speed up the process by reducing ΔG‡ protease t½ up to 1000 years for uncatalyzed reaction at neutral pH! 5 Specificity nomenclature: Protease/substrate interactions The bond cleaved is called the scissile bond Residues are numbered from the scissile bound outward Peptide substrate: ― Residues on the N-terminus side of the scissile bond are labelled P1, P2, P3… ― Residues on the C-terminus side of the scissile bond are labelled P1’, P2’, P3’… Corresponding binding Sites on the enzyme are labelled S and S’. ←N-terminus C-terminus→ Unprimed sites Scissile bond Primed sites N-terminal product of cleavage C-terminal product of cleavage 6 Four classes of proteases Although there are four classes of proteases, they all display a similar catalytic strategy: To generate a powerful nucleophile that attacks the carbonyl moiety in a peptide bond. 1. Serine Proteases: Have a Ser residue in the active site whose hydroxyl group acts as a nucleophile 2. Cysteine Proteases: Have a Cys residue in the active site whose thiol group acts as a nucleophile 3. Aspartyl Proteases: Use an Asp residue to activate a water molecule 4. Metalloproteases: Contain a metal ion which activates a complexed water molecule 7 Chymotrypsin: Serine protease How do you know it is a serine protease (i.e. a serine residue acts as a nucleophile)? React it with the irreversible inhibitor DIPF (Diisopropyl fluorophosphate, a group specific reagent) − Chymotrypsin has 28 serine residues but only Ser 195 is modified − It loses all activity Thus Ser 195 must play a role in catalysis! 8 Specificity of Chymotrypsin Specificity of cleavage arises from the deep pocket of S1 being lined with hydrophobic residues which favor the binding of large hydrophobic side chains in P1. prefers to hydrolyze peptide bonds on the carbonyl side of aromatic or large hydrophobic amino acids (e.g. mainly Phe, Tyr, Trp, Met) Ser195 is positioned to cleave directly after the residue located in the S1 pocket (Phe). Chymotrypsin OH + H2O P1 S1 Lehninger, Principles of Biochemistry 6th edition. Chymotrypsin: Proof of covalent catalysis e.g. Hydrolysis of N-Acetyl-L-phenylalanine p-nitrophenyl ester at pH 7 chymotrypsin + 2H+ + O- p-nitrophenolate N-Ac-Lcolorless! -Phe-p-NO2-phenyl ester Yellow in color! Kinetics of catalysis Two phases: 1. Burst phase (pre-steady state) 2. Steady-State Phase The fact that we see two phases, means an intermediate enzymatic species must accumulate which is relatively stable (thus must be covalent) 10 Chymotrypsin: Acyl-enzyme intermediate R’ is a generic chain starting with a C Acylation Deacylation RO R’ ROH R’ R’ Burst phase: Steady-state phase: Acylation reaction occurs to form the De-acylation reaction occurs to acyl-enzyme intermediate: regenerate the free enzyme: ― Acyl group becomes covalently ― Acyl enzyme is hydrolyzed which attached to E releases the carboxylic acid ― Briefly, E is covalently modified component of S and free E ― A product ROH is released that ― Slow step absorbs color ― Fast step Note: Double-displacement (Ping-pong) reaction with enzyme-intermediate, covalent catalysis 11 Catalytic triad Active site of Chymotrypsin Ser195 is part of the active site of chymotrypsin. But what makes Ser195 so reactive? CATALYTIC TRIAD composed of Asp102, His57 and Ser195 Note: The aromatic side chain of the substrate sits in a deep hydrophobic pocket Lehninger, Principles of Biochemistry 6th edition. 12 Catalytic triad: Converts Serine 195 to a potent nucleophile Serine has an hydroxyl in its side chain, and hydroxyl groups are poor nucleophiles! So why is serine 195 a reactive species? ‒ Ser195 is H-bonded to His57 Hydrogen bonds stabilize the catalytic triad ‒ His57 is H-bonded to Asp102 ‒ His57 is placed ‘just right’ within the active site to polarize the hydroxyl side chain of Ser. ‒ In the presence of the substrate, His57 accepts the proton of Ser195 generating an alkoxide ion (excellent nucleophile that attacks the peptide bond of the substrate) His activates Ser General-base catalysis ‒ Asp102 orients His57 to make it a better proton acceptor through H-bonding. Asp activates His 13 Chymotrypsin: Mechanism for hydrolysis (1/9) Note: Although not shown, in principle as with all enzyme -catalyzed reactions, all steps reversible 14 Let’s look at the mechanism step by step… Chymotrypsin: Mechanism for hydrolysis (2/9) oxyanion hole oxyanion hole 1 Michaelis Complex (ES complex) Non-covalent intermediate Step 1: Substrate binds 15 Chymotrypsin: Mechanism for hydrolysis (3/9) oxyanion hole oxyanion hole 2 ES Complex Tetrahedral Intermediate 1 Step 2: In a concerted fashion, acting as a general base, His57 deprotonates the OH group on the side chain of Ser195. The resulting alkoxide makes a nucleophilic attack on the amide carbonyl group of the Scissile bond of the substrate. Asp helps polarize His through H-bonding, increasing its basicity. The resulting carbon on the substrate is now tetrahedral instead of planar. The tetrahedral intermediate has a formal charge of -1 on the oxygen which is stabilized by H-bonding interactions in the oxyanion hole. 16 Oxyanion hole The oxyanion hole is formed by the backbone (main chain) of the protein. Two H-bonding interactions stabilize the tetrahedral intermediate and the transition state leading to its formation (step 2 and step 6) ‒ Interactions between NH group of Gly193 with the negatively charged ‒ Interactions between NH group of Ser195 oxygen atom of the intermediate 17 Chymotrypsin: Mechanism for hydrolysis (4/9) oxyanion hole 33 Tetrahedral Intermediate 1 Acyl-Enzyme Step 3: To form the acyl-enzyme, the tetrahedral intermediate 1 must break down. In order to do so, the amine must be made into a better leaving group by protonation with the positively charged His57 His57 acts a general acid. Immediately following acyl-enzyme formation, the amine remains bound to the His. 18 Chymotrypsin: Mechanism for hydrolysis (5/9) 4 Acyl-Enzyme Acyl-Enzyme Step 4: The newly formed amine product (H2N-P1’-P2’-P3’…) now departs from the enzyme. Completion of the first stage of hydrolysis: ACYLATION Next stage: DEACYLATION 19 Chymotrypsin: Mechanism for hydrolysis (6/9) 5 Acyl-Enzyme Acyl-Enzyme Step 5: A molecule of water is strategically bound into the active site (at the place where the departed amine used to be). 20 Chymotrypsin: Mechanism for hydrolysis (7/9) oxyanion hole 6 Acyl-Enzyme Tetrahedral Intermediate 2 Step 6: Similar to step 2, in a concerted fashion and acting as a general base catalyst, His57 deprotonates the water which allows the hydroxide to make a nucleophilic attack on the ester carbonyl of the acyl-enzyme. The resulting carbon on the substrate is now tetrahedral instead of planar. The tetrahedral intermediate has a formal charge of -1 on the oxygen. The developing negative charge is stabilized by H-bonding interactions in the oxyanion hole. 21 Chymotrypsin: Mechanism for hydrolysis (8/9) oxyanion hole 7 Tetrahedral Intermediate 2 Step 7: The breakdown of the tetrahedral intermediate 2 involves re-protonation of the Ser195 leaving group by the positively charged His 57. His57 acts a general acid The tetrahedral intermediate collapses to form a carboxylic acid product. The carboxylic acid is still bound within the active site. 22 Chymotrypsin: Mechanism for hydrolysis (9/9) 8 Free Enzyme Step 8: Release of the carboxylic acid product (…P2-P1-COOH) regenerates the free enzyme. 23 Catalytic triads in other hydrolytic enzymes Many other proteases have been identified that are homologs of chymotrypsin (have a common ancestor). These enzyme are the result of divergent evolution. Trypsin, Chymotrypsin and Elastase have very similar structures and share over 40% identical residues in their sequence and share same catalytic triad strategy! Yet they have different specificities. How is this possible? Overlay of the backbones of Chymotrypsin and Trypsin 24 Specificity of Chymotrypsin, Trypsin, and Elastase The active site of these proteases have an S1 pocket (specificity pocket) whose size/shape/charge determines which polypeptide sequence will be cleaved. P1 must be complementary to S1. Thus the specificity differences between Chymotrypsin, Trypsin, and Elastase are due to small structural differences in the binding pocket. S1 S1 S1 Chymotrypsin Trypsin Elastase S1 is deep and lined S1 is deep with -ve S1 is shallow since the with hydrophobic charged Asp189 at the side chains of Val216 and residues bottom of the pocket Val190 sterically close off It can accept Phe, Tyr, It can accept long +ve the pocket. Trp, Met in P1 to charged side chains in It can accept small side maximize P1 (i.e. Arg, Lys) to chains in P1 (i.e. Ala) hydrophobic maximize electrostatic since they can fit in S1. interactions. interactions. 25 Chymotrypsin vs Subtilisin Both these two enzymes are serine proteases. Their 3⁰ structures look different, yet they still employ the same catalytic triad in nearly identical spatial arrangements. These two enzymes are the result of convergent evolution. Since this catalytic triad appears in different courses of evolution, it must be very effective! 26 Site-directed mutagenesis: Subtilisin Site directed mutagenesis allows a (natural enzyme) single amino acid (or multiple) residue in a protein to be changed in order to determine how important it is in the functioning of the enzyme. Mutating catalytic triad residues did not affect KM but affected kcat residues not needed for binding, but for catalysis Mutating each residue individually showed a dramatic decrease in kcat. (Note the log scale!) This proves that the Asp-His-Ser triad serves to generate a powerful nucleophile that can attack the carbonyl of a peptide bond. Even mutated, the enzyme can hydrolyze the reaction 1000 times faster than uncatalyzed. 27 Site-directed mutagenesis: Subtilisin Asn155Gly Mutating Asn155 to a Gly has little effect on binding the kcat 0.2% of wild type substrate but it decreases the KM Increased by only 2-fold catalytic rate to only 0.2% of its value in the wild-type enzyme. Why? Because the side chain which stabilizes the tetrahedral intermediate has been eliminated. If the rate goes down, the TS leading to the formation of the tetrahedral intermediate must be higher in energy and less stable. Thus the oxyanion hole stabilizes Oxyanion hole of subtilisin involves: the tetrahedral intermediate and Asn155 side chain NH2 the transition state leading to its Ser221 backbone NH formation. Serine proteases use transition state stabilization as a method of catalysis 28 Other proteases use different catalytic strategies Cysteine protease Aspartic protease Metalloprotease Catalytic strategy similar to Two Asp residues act together The active site chymotrypsin in order to activate H2O for contains a bound A Cys residue, activated by attack on the carbonyl carbon metal ion (almost a His residue, acts as the of a peptide bond always Zn2+) that nucleophile and attacks the One Asp is deprotonated and activates a water carbonyl carbon of a abstracts a proton from H2O molecule for attack peptide bond. (general base) on the carbonyl Since sulfur in Cys (pKa 8.3) The other Asp is protonated carbon of a peptide is a better nucleophile than and polarizes the carbonyl so bond. oxygen in Ser (pKa 13), that it becomes susceptible to ‘B’: is a base (often a catalytic design only attack (general acid) glutamate) that requires His-Cys and not helps deprotonate the triad Asp-His-Cys the H2O 29 On your own Summary: Common features of the active site of proteases Serine protease Cysteine protease Aspartyl protease Metalloprotease 1. Activation of a water molecule or other nucleophile 2. Polarization of the peptide carbonyl group (not shown above) 30 3. Stabilization of the tetrahedral intermediate 30 Catalytic strategies by an enzyme different than a protease 31 Carbonic anhydrase: What does it do? Carbonic anhydrase Active site: Carbonic anhydrase II Carbonic anhydrase catalyzes reversible conversion of CO2 to bicarbonate. In tissues, the enzyme in red blood cells (RBC) converts CO2 to bicarbonate. As the blood passes in the lungs, the reverse reaction occurs, the enzyme in RBC dehydrates bicarbonate so that CO2 can be exhaled. Although the reaction is fast without enzyme at room temperature, the enzyme enhances the rate and works at diffusion limits! Active site of carbonic anhydrase II found in RBC: Contains a Zn2+ ion coordinated to the imidazole rings of three Histidine residues and to one water molecule. 32 Carbonic anhydrase: Proposed mechanism Increased acidity Coordination bond Hydrophobic Hydrophilic 1. When water binds to Zn2+, its pKa decreases from 15.7 to 7. Zn2+ increases the acidity of the proton on water, making it easier to generate a hydroxide ion (a more potent nucleophile). 2. Adjacent to the zinc site is a hydrophobic patch that allows CO 2 to bind. This positions the CO2 molecule nicely for attack by the Zn-bound OH-. 3. The OH- attacks CO2 converting it to bicarbonate. 4. The active site is regenerated upon release of HCO 3- and binding of H2O. 33 Carbonic anhydrase: Metal ion catalysis Increased acidity Coordination bond By having the Zn2+ ion bind water, the formation of the transition state is favored Metal ion catalysis – pKa of water is lowered, making it easier to release a proton – Via catalysis by approximation the water molecule is positioned to be in close proximity to the CO2 34 Carbonic anhydrase: His proton shuttle Increased acidity 1. The zinc-bound water molecule must lose a proton to begin the hydration reaction. His64 picks up the proton from the zinc-bound water molecule, generating the hydroxide ion and protonated histidine. 2. In order to prevent the reprotonation of the OH− and to regenerate the unprotonated His, a molecule of buffer (B) remove a proton from the histidine. The His serves as a proton shuttle, transferring the proton from water to the buffer. 35 Rate of CO2 hydration affected by buffer concentration Increasing the buffer concentration enhances the rate of CO2 hydration, suggesting that buffers are important in the reaction mechanism. Proton shuttle transfers protons from the zinc-bound water molecule to the protein surface via His 64 and then to the buffer Why? Buffer too large to reach active site, thus needs His to transfer it to the buffer 36 In class problem Which one of the following is the most accurate statement regarding the catalytic mechanism of the enzyme chymotrypsin? A. The catalytic mechanism involves an oxyanion hole that stabilizes the substrate. B. The catalytic mechanism of chymotrypsin involves a catalytic triad composed of an aspartate, a histidine, and a serine residue. C. The catalytic mechanism of chymotrypsin involves the formation of a covalent thioester intermediate. D. The catalytic mechanism of chymotrypsin involves a Zn2+ ion that interacts with a histidine residue in the active site E. The catalytic mechanism of chymotrypsin involves destabilization of the tetrahedral intermediate 37 In class problem In the presence of substrate, the histidine residue of an enzyme accepts a proton from a serine hydroxyl group, generating an alkoxide ion, which is a powerful nucleophile. What kind of catalytic strategy is described? 38 In class problem Fill in the blank with the best option. The specific cleavage of peptide bonds by chymotrypsin ________. A. results in the cleavage at the peptide bond after amino acids with small side chains, such as alanine and serine. B. results because the enzyme contains a specificity pocket lined with hydrophobic residues. C. depends almost entirely on which amino acid is directly on the carboxyl-terminal side of the peptide bond to be cleaved. D. occurs in the active site of the enzyme which is located in the interior of the enzyme. E. occurs when long positively charged side chains fit into the S 1 pocket. 39