Neuroscience Past Paper PDF - University of Central Lancashire
Document Details
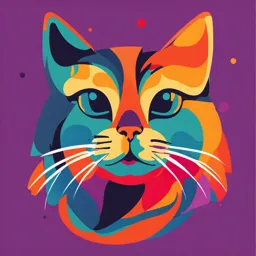
Uploaded by WinningHoneysuckle
University of Central Lancashire
2025
Constantin-Iulian Chiță
Tags
Summary
This is a presentation on neuroscience, focusing on neuroplasticity and relating learning objectives with the notion of plasticity. It also includes discussion on critical periods and factors affecting brain plasticity.
Full Transcript
27th January 2025 XY3291 - Neuroscience Constantin-Iulian Chiță Where opportunity creates success Neuroplasticity Where opportunity creates success Learning objectives Understand the notion of plasticity and its implications for understanding how experience continuously shape our brain...
27th January 2025 XY3291 - Neuroscience Constantin-Iulian Chiță Where opportunity creates success Neuroplasticity Where opportunity creates success Learning objectives Understand the notion of plasticity and its implications for understanding how experience continuously shape our brain Demonstrate a comprehensive understanding of the molecular mechanisms of LTP and LTD Describe key features of central nervous system damage and the potential of repair mechanism Christmas, D., Morrison, C., Eljamel, M. S., & Matthews, K. (2004). Neurosurgery for mental disorder. Advances in Psychiatric Treatment, 10(3), 189-199. Christmas, D., Morrison, C., Eljamel, M. S., & Matthews, K. (2004). Neurosurgery for mental disorder. Advances in Psychiatric Treatment, 10(3), 189-199. Nowadays, only 4 neurosurgical procedures are still being used - Anterior Capsulotomy - Anterior Cingulotomy - Stereotactic Subcaudate Tractotomy - Limbic Leucotomy Only the first two are performed in the UK. Statement on Neurosurgery for Mental Disorder (NMD) by rcpsych.ac.uk - https://www.rcpsych.ac.uk/docs/default-source/about-us/who-we-are/ectcommittee-vns-dbs-ablative- neurosurgery-statement-feb17.pdf?sfvrsn=eba0287a_4 Critical periods Animals have a behavioural repertoire for survival→ imprinting (Konrad Lorenz, 1973) Critical periods: when sensory experience and neural activity have maximal effect on the acquisition of a particular behaviour (temporal windows). Both need to coincide for the critical period to open properly Critical periods vs sensitive periods (as more extended Periods, learning is still possible) Deprivation experiments→ how the experience can affect the innate characteristics Competitive interaction→ leading to the Hebbian theory – if two neurons both have the potential to make a connection with a cell, the neuron that fires more will make the connection. Hebb’s Postulate Donald Hebb (1949) – theory of synaptic plasticity: “When an axon of cell A is near enough to excite a cell B and repeatedly or persistently takes part in firing it, some growth process or metabolic change takes place in one or both cells such that A's efficiency, as one of the cells firing B, is increased.” Or, put simply, “cells that fire together wire together”. = associativity Neuroplasticity Ability of the brain to change continuously throughout an individual's life Optimize the neural networks during phylogenesis, ontogeny and physiological learning, as well as after a brain injury Behaviour, environmental stimuli, thought, and emotions may also cause neuroplastic change Types of neuroplasticity? 1. Functional (experience-dependent plasticity) - adapt the functional properties of neurons. Related with memory and learning (synaptic plasticity) 2. Structural - change its neuronal connections and the brain’s anatomical reorganization. Related with brain repair and regeneration General principles 1. Changes in the brain can be shown at many levels of analysis 2. Different measures of neuronal morphology change independently of each other and sometimes in opposite directions 3. Experience-dependent changes tend to be focal 4. Plastic changes are time-dependent 5. Plastic changes are age-dependent 6. Experience-dependent changes interact 7. Not all plasticity is good General principles 1. Changes in the brain can be shown at many levels of analysis 2. Different measures of neuronal morphology change independently of each other and sometimes in opposite directions 3. Experience-dependent changes tend to be focal 4. Plastic changes are time-dependent 5. Plastic changes are age-dependent 6. Experience-dependent changes interact 7. Not all plasticity is good Factors influencing the brain plasticity Sensory and motor experience Psychoactive drugs Gonadal hormones Parent-child relationships Peer relationships Stress Intestinal flora Diet Synaptic plasticity Strength of synaptic connections consider as dynamic entity This changes alters the synaptic transmission: 1. Short-term – from milliseconds to minutes - Facilitation (↑) → → is a rapid increase in synaptic strength that occurs when 2 or more action potentials invade the presynaptic terminal within few milliseconds - Augmentation (↑) and Potentiation (↑)→ elicited by repeated synaptic transmission and serve to increase the amount of neurotransmitter released from the presynaptic terminal - Depression (↓) → cause neurotransmitter release to decline during synaptic activity 2. Long-term – from minutes to hours - Long term potentiation (↑) - Long-term depression (↓) How does the synapse become stronger? Think about the steps of synaptic transmission: 1) Presynaptic excitability More excitable 2) Release of neurotransmitter More NT release 3) Activation of postsynaptic receptors: Sensitivity More conductance Quantity of receptors More receptors 4) Number of synapses More synapses Long-term potentiation (LTP) Long-term potentiation is an increase in the postsynaptic response to presynaptic activity. The synapse becomes stronger Excitatory (glutamatergic) – depolarisation of postsynaptic cell LTP EPSP Larger EPSP LTP discovery: Bliss & Lomo (1973) Landmark paper: first experimental demonstration of LTP Field recording of perforant path to dentate gyrus HFS either 100Hz (3-4 seconds) or 10-20Hz (10-15s) Long-lasting: up to 10 hours The results suggest that two independent mechanisms are responsible for long-lasting potentiation: ▪ an increase in the efficiency of synaptic transmission at the perforant path synapses; ▪ an increase in the excitability of the granule cell population. Properties of LTP Coincidence detector - LTP require strong activity in the presynaptic and postsynaptic neuron. This requirement is basically the Hebb’s theory (coordinated activity between the pre and post synaptic neuron) Specificity - LTP is input specific. When LTP is induced by activation of one synapse, it does not occur in the other inactive synapse. LTP is restricted to activate synapse rather than to all the synapses in the cell. Associativity - when the pairing of one weak pathway with a neighbouring strong activated pathway the cell undergo LTP. This property is often considering the molecular basis of the associate learning , where 2 stimuli are required for learning. Molecular mechanism of LTP NMDA-receptor mediated LTP Best characterised form of LTP Found across multiple brain regions Best characterised at Schaffer collaterals between CA3 and CA1 pyramidal cells (hippocampus) NMDA receptor functions as a coincidence detector NMDA-receptor Mg2+ pore block gives this receptor unique characteristic: Binds glutamate – senses presynaptic activity Mg2+ block of pore – senses postsynaptic depolarisation = coincidence detection But remember: AMPA receptors depolarise the membrane LTP protocol Recording Stimulating electrode electrode 1s @ 100Hz LTP induction Protocol: 1) Stimulate at 0.1 Hz, record stable baseline 2) Apply high frequency stimulus (HFS; tetanus) 3) Return to 0.1Hz stimulation Why HFS? LTP has a threshold Vm Must depolarise postsynaptic cell sufficiently Threshold Individual EPSPs too weak Summation of EPSPs -70mV Time Presynaptic release of glutamate Activation of postsynaptic AMPARs Depolarisation (HFS summating to threshold) Activation of NMDARs: coincidence detection Calcium entry through NMDARs Signalling mechanism underlying LTP Glu release→ the NMDA receptor channel opens only if the postsynaptic cell is sufficiently depolarized Ca2+ ions → activate CaMKII and PKC→ phosphorylation reactions → regulate trafficking of postsynaptic AMPA receptors through recycling endosomes → leading to insertion of new AMPA receptors→ increase in the spine’s sensitivity to glutamate, which causes LTP Mechanism for long-lasting changes in synaptic transmission during LTP Changes in gene expression and new proteins Initiated by PKA activating the transcriptional regulator CREB Construction of new synaptic contacts LTP induces creation of “perforated synapses” – synapses with multiple, separated, active zones Perforated synapse LTP pre- / post- synaptic densities active zones Perforated synapses grow and develop Eventually the dendritic spine branches and separates into two new spines / synapses Requires extensive cytoskeletal remodelling and gene transcription Silent synapses Silent synapses Especially prevalent in development NMDAR AMPAR Weak or silent synapses incapable of sufficient depolarisation (NDMAR and Mg blocking) Depolarisation may spread from nearby strong synapses → Co- operativity in action When the synapse mature → AMPA receptors are recruited Long-term depression Removal of unwanted or unnecessary connections – free up processing power for re-use. Homeostasis – prevent overactivity? Homeostasis – maintain dynamic range of synaptic activity LTD at the Schaffer collateral-CA1 LTD is also NMDAR and Ca2+ dependent LTP induced by strong, associative activity: high Ca2+ for short periods → activation kinases LTD induced by weak (1Hz) for long periods, non- associative activity → modest Ca2+ concentrations for a sustained period → activation pf phosphatases PP1 and PP2B (calcineurin) Cerebellar LTD First characterised by Masao Ito (1982, 1984) Observed at Purkinje cells → receive information from 2 types of excitatory inputs: climbing fibers and parallel fibers Implicated in the motor learning LTD require depolarisation of the Purkinje cell produced by climbing fiber activation and signals generated by the active parallel fiber at the same time = associate Both PFs and CFs must be activated within certain timeframe LTD most effective with CF activation after PF (peak ~ 80ms latency) LTD is expressed at PF-PC synapses, but not CF-PC. Mechanism underlying cerebellar LTD - Glutamate from PF → activates AMPA-type and metabotropic glutamate receptors (mGLURs) - IP₃ and Ca²⁺ as second messenger (acting together in IP₃ receptors) - PKC activation by DAG and Ca²⁺ - Internalization of AMPA receptor= ↓ response postsynaptic Purkinje cell Brain repair and regeneration PNS vs CNS Brain functional recovery without repair – Doesn’t reflect the regrowth of axons or replacement of damaged neurons – Undamaged brain regions eventually become activated and reorganized 1. “unmasked connections” → connections that might not be active when the system is intact can be unmasked when there is a damage near by 2. LTP and LTD → plastic changes 3. ipsilateral/contralateral pathways Neuronal regeneration or repair A. Regrowth of axons B. Restoration of damaged central nerve cells C. Genesis of new neurons Cellular response to injury in the CNS Damage to the CNS: physical trauma, ischemia, stroke, cardiac arrest, neurodegenerative diseases There are 3 major reasons for the differences between successful peripheral regeneration and the limited regeneration in the CNS 1. Necrotic and apoptotic processes 2. Glia scar formation → combination of glia growth and proliferation along with microglia activity actively inhibits the growth 3. Immune-mediated inflammatory response → Activation of glia cells by pro-inflammatory cytokines 1. Necrotic and apoptotic processes Extended neuronal cell death Apoptosis activated by direct damage into the cell or excitoxicity ↑ activation caspase-3 Autophagy can prevent or lead to neuronal degeneration ❖ Model of the primary mechanism for neuronal apoptosis after injury Apoptosis→ via excess of Glu or binding cytokines or loss of neuronal connections Removal of the anti-apoptotic gene Bcl2→ Cytochrome c is then released from mitochondria→ activating caspase-3 → apoptotic cell 2. Glia scar formation Astrocytes, oligodendrocytes and microglia respond to a brain injury, opposing the neuronal growth Glia precursors or extensive growth of existing glia in or around injury Myelin-associated protein → several inhibitory molecules prevent the axon growth and contribute to the glia scars. Myelin-associated glycoprotein (MAG) as some ECM molecules, cytokines ❖ Cellular response to injury in the CNS. Local changes at or near an injured site → degeneration of myelin and other cellular elements The clearing of this debris by microglia (phagocytic cells in the CNS) Local production of inhibitory factors → limit axon growth 3. Immune-mediated inflammatory response Increase the resistance to neuronal regrowth and repair Damage to the brain tissue→ BBB disruption (by disrupting the tight junctions) → invasion of neutrophils and monocytes → invasion of microglia and astrocytes and finally the invasion of T and B cells Activation of glia cells by pro-inflammatory cytokines→ IL-1 → forms a protective barrier for adjacent healthy brain tissue Neurogenesis in the CNS Process by which the neurons are produced by neural stem cells (different from prenatal neurogenesis) Two regions of the brain 1. For the olfactory bulb are found in the anterior subventricular zone(SVZ) 2. For the hippocampus in the subgranular zone (SGZ) New cells in the CNS: interneurons (granule cells and periglomerular cells) Some these neurons are integrated into functional synaptic circuits but the most of them die before being integrated Neuronal stem cells Characteristics similar to astrocytes Regulated by signalling molecules→ proximity to the blood vessels To generate differentiated neurons and glia → the neural stem cells must give rise to an intermediate precursor cell class→ Transit amplifying cell Transit amplifying cell → Cell division is faster and asymetrically Migration of new neurons Newly generated and still undifferentiated neurons and glia cells → move away from the SGZ and SVZ into the regions of the olfactory bulb or hippocampus 1. SGZ Hippocampus → the distance is relatively small, and the cells undergo to a local movement. 2. SVZ olfactory bulb → the distance is higher → migratory route defined → glia cells facilitates the migration of this newly neurons → rostral migratory pathway RMS no expressed in humans ECM influences migration - NCAM which promote cell to cell interaction - Neuregulin and Integrin receptors which also influence the axon guidance and synapse formation