Acid-Base 1 Notes PDF 2024
Document Details
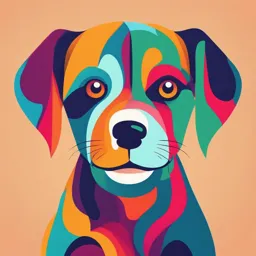
Uploaded by FruitfulIntegral
WSUSOM
2024
Tags
Summary
These notes cover acid-base physiology, focusing on body fluid buffering and titration. Learning objectives, types of buffers, and the Davenport diagram are discussed. It also details acid production, the CO2/HCO3 system, and the Henderson-Hasselbalch equation.
Full Transcript
WSUSOM Medical Physiology Rossi-Acid Base Physiology Page 1 of 15 Body fluid buffering and titration Acid Base 1: Body Fluid Buffering and Titration Learning Objectives: 1. General terms A. Define what is meant by the acid-base state of an organi...
WSUSOM Medical Physiology Rossi-Acid Base Physiology Page 1 of 15 Body fluid buffering and titration Acid Base 1: Body Fluid Buffering and Titration Learning Objectives: 1. General terms A. Define what is meant by the acid-base state of an organism and why pH is regulated B. Distinguish between volatile and nonvolatile acids bases and how the composition of diet determines which are generated C. Define and apply the concepts of partial pressure, equilibrium, and buffering, buffer value D. Distinguish buffering from compensation 2. Types of buffers A. Identify the body fluid compartments that are in acid base equilibrium and how they relate B. Know the role of hemoglobin in buffering systemic pH C. Understand the operation of the HCO3-/CO2 system and its pivotal role in acid base balance D. Learn the modification of the Henderson Hasselbalch equation as it relates to body pH E. Be aware of the role of other acid base buffers in regulating systemic pH 3. Using the Davenport Diagram for buffering in the body A. Become familiar with and be able to apply the acid base map to conditions B. Be able to show how the body titrates acids and bases at a fixed pCO2 along the isobar C. Distinguish between how body fluids are titrated with carbonic acid vs with non- carbonic acid buffers D. Be able to identify and quantitate base deficit and base excess WSUSOM Medical Physiology Rossi-Acid Base Physiology Page 2 of 15 Body fluid buffering and titration Acid Base and its Regulation This figure on the right is the Davenport diagram. It is the acid-base “map.” This diagram tries to represent FOUR dimensions in TWO dimensional graphic. - pH on the X-axis - HCO3- on the Y-axis - pCO2 on the isobars (curved lines) - hemoglobin titration curve (slanted line) By the end of the acid-base lectures you should be able to “find your way around the map” to solve the single acid-base problems. Body Acid-Base State What is meant by the term “body acid-base state”? The fluids in the body can be titrated with acid or base and the pH altered just like a solution in a beaker. 1. The body is divided into separate but interacting fluid or semi-fluid compartments, such as bone, plasma, interstitial fluid, intracellular fluid (other than RBCs) and intracellular fluid in RBCs. The final acid-base state of the body is a composite of the acid-base states of the various compartments. These may change over time (minutes to weeks). 2. For practical purposes, the term acid base state of the “body” is usually taken to mean the acid base state of “arterial blood” or of “extracellular fluid” (ECF). It is assumed that the body is in equilibrium and that the pH of ECF reflects the pH of the other compartments (this is not necessarily true, e.g., cerebrospinal fluid tends to have lower pH than other ECF). For our purposes we will use the overall simplification that pH of ECF or arterial blood reflects body pH. Importance of pH H+ + enzyme1(inactive) « enzyme1H+ (active) or H+ + enzyme2(active) « enzyme2H+ (inactive) WSUSOM Medical Physiology Rossi-Acid Base Physiology Page 3 of 15 Body fluid buffering and titration The functional activities of proteins (enzymes, transport molecules, contractile molecules, etc.) depend on the pH. This is represented in the equations above. pH needs to be regulated to provide optimum environment for the numerous protein-mediated processes occurring in the body. Biochemistry in vivo!!! Acid Production CO2 oxidation of carbohydrates and lipids – Metabolic production » 13,000 mmol/day – Removed by exhaling 300 L CO2/day – Class 1 (volatile) Oxidation of P and S in proteins and nucleotides – Formation of H3PO4 and H2SO4 (~ 70-100 mmol/day) – Excreted by the kidney – Class 2 (nonvolatile) How is pH altered? - The body is constantly producing ACID as a result of metabolism. - Typical daily amounts of acid generated are listed above. Note the difference in amounts. Much more volatile acid is produced than nonvolatile acid. Class 1 acids: volatile (carbonic acid) Class 2 acids: non-volatile (all other acids) Excess acid is removed from the body by excretion 1. When carbonic acid is dehydrated to CO2, it becomes volatile and is excreted by the lungs. 2. Other non-carbonic acids are excreted by the kidneys. The CO2/HCO3 System CO2 + H2O « H2CO3 « H+ + HCO3- pCO2 = partial pressure of CO2 H2CO3 is an acid Body is an open system – Volatile acid, CO2, is controlled by intake/output by the lungs – Nonvolatile acid excretion is controlled by the kidneys – Why is pCO2 (partial pressure of CO2) regulated in body fluids? 1. The pCO2 is a measure of the concentration of dissolved CO2 in a fluid. 2. CO2 in its hydrated form, H2CO3 (carbonic acid), is an acid. Its concentration is regulated as part of the pH regulatory process. WSUSOM Medical Physiology Rossi-Acid Base Physiology Page 4 of 15 Body fluid buffering and titration H2CO3 Regulation How are pH and pCO2 regulated in body fluids? The essential concept is that the body is an open system, exchanging materials and energy with the external environment. The amounts of carbonic and non-carbonic acid in the body are regulated by controlling the intake and/or output of CO2 and nonvolatile acids. * Carbonic acid regulation: pCO2 or ¯pH ® alveolar ventilation – The rate of CO2 excretion is proportional to alveolar ventilation – Either an increase in pCO2 or a decrease in pH have a stimulatory effect on the brain respiratory control center. – An increase in alveolar ventilation decreases alveolar pCO2 and, by rapid equilibration, also decreases arterial pCO2. The loss of carbonic acid thus increases pH toward normal – A decrease in alveolar ventilation would have the opposite effect. Non-carbonic Acid Regulation Non-volatile acid regulation: H+ or ¯pH ® acid excretion by kidneys Non-volatile acid content of the body is determined by (1) rate of intake of acid in food (protein) (2) the rate of production by metabolism (catabolism) (3) rate of excretion of H+ ions by the kidneys. WSUSOM Medical Physiology Rossi-Acid Base Physiology Page 5 of 15 Body fluid buffering and titration An increase in volatile acid will also result in acid excretion by kidneys (but this is in “compensation” which will be discussed in the next lecture). The rate of acid secretion by the kidney is controlled by the pCO2 and pH. Either an increase in pCO2 or a decrease in pH will increase the rate of non- volatile (non-carbonic) acid secretion and excretion by the kidneys. Acid Base Unbalance COPD (chronic obstructive pulmonary disease) – impaired gas exchange in lungs leading to accumulation of carbonic acid in body fluids Diabetic ketoacidosis – addition of b-hydroxybutyrate and acetoacetate leading to surplus of non- carbonic acid in body – The above are examples of common causes of acid base unbalance. COPD causes accumulation of CO2 and hence carbonic acid, volatile acid Uncontrolled diabetes mellitus with ketoacidosis causes addition of non-carbonic acids (non-volatile acids) to the body. Buffering: the concept That property of a dissolved substance to reduce the change in pH that results from the addition of a strong acid or strong base to that solution. Definitions 1. Strong electrolytes - completely ionize in solution e.g., NaCl, HCl 2. Weak electrolytes - partially ionize in solution. E.g., lactic acid, ketoacids, etc. 3. Acid - a substance that can supply H+ 4. Base - a substance that can take up H+ ions E.g, dissociation of acetic acid: HAc « H+ + Ac- acetic acid hydrogen ion acetate Acetate is called the conjugate base. WSUSOM Medical Physiology Rossi-Acid Base Physiology Page 6 of 15 Body fluid buffering and titration Dissociation Constant Ka’ of a Weak Acid We will use the imidazole solution as our example. The dissociation reaction is shown. Im + stands for imidazole in our equations here. All H+ ion buffers in the body are weak acids or weak bases. A weak acid or weak base acts as a buffer against the changes in pH over that range of pH values where the concentrations of the acid and the conjugate base forms are comparable (When the concentrations are equal, this is the pK). A solution containing weak acid and its conjugate base acts as a buffer against both added acid or added base, because there are molecules that can either supply H+ or absorb H+. The ONLY substances in the body occurring in sufficient concentration and having a pK in the range of normal body pH value (7.40) possess the imidazole ring of the amino acid histidine or are phosphates. K’a is the equilibrium coefficient characteristic of this reaction. The prime sign (K’a rather than Ka) indicates that concentrations rather than activities are used and that the temperature is body temperature. K’a values are assumed to be constant in the body due to constant temperature and ionic strength of body fluids. pH = pKa’ + log __[Im]___ [B] - [Im] Note the resemblance of the equation above to Henderson – Hasselbach Equation! (The derivation is provided in the slides for those who like to know how this equation comes to be.) Imidazole ring of Histidine Notice that imidazole has acidic and basic forms. The pKa of »7.0 is very close to the physiologic pH (may vary slightly depending on the adjacent amino acids on the protein) Body fluids are normally at 7.40. WSUSOM Medical Physiology Rossi-Acid Base Physiology Page 7 of 15 Body fluid buffering and titration Titration Curve A titration curve is the plot of the concentration of added strong acid (or base) against the pH of the solution. 1 mEq acid = 1 mmol H+; 1 mEq base = 1 mmol base- (note single charge) (1 mEq reflect 1 mmol of charge; if a compound has two charges, like Ca2+ then 1 mmol Ca2+ is actually 2 mEq; chemistry texts do not use mEq much anymore but the medical texts still do?!) Buffer value is the slope of the titration curve at any given pH. We can calculate the titration curve for Imidazole. Assume that all added acid or base combines with the buffer. Solve for [H+], pH = pK’a + log __[Im]___ [B] - [Im] Titration Curve for Imidazole Two points: 1. The curve is steepest in the neighborhood of pH = 7.0 where pH = pK. Corollary: When the pH is closest to the pK there is the least change in pH for each mEq of added acid (or base). 2. The titration curve is approximately linear between pH 6.5 and 7.5. Since the range of body fluid pH values compatible with life is less than one pH unit, titration curves for body fluids can be accurately approximated by straight lines. You can also represent the titration “curve” as a Table. Table 1: Titration of a 20 mEq solution of Imidazole Equilibrium condition: Him+ = H+ + Im. Ka = 10 -7 M = [H+]*[Im]/[Him+] Titration reaction: IM + HCl = Him+ + Cl-; HIM+ + NaOH = Na+ + Im + H2O Initial conditions: [Him ] = 10 mEq; [Im] = 10 mEq; H+ = 100 nEq, pH = 7.00 + Conc of added acid [Him+] log [Im] mEq [Im] [Him+] [Im] H+ ………..[Him+] pH -9.5 19.5 0.5 0.026 2.56 1.59 8.59 -9.0 19.0 1.0 0.053 5.26 1.28 8.28 -8.0 18.0 2.0 0.111 11.1 0.95 7.95 -6.0 16.0 4.0 0.250 25.0 0.60 7.60 -4.0 14.0 6.0 0.429 42.9 0.37 7.37 -2.0 12.0 8.0 0.667 66.7 0.18 7.18 0.0 10.0 10.0 1.000 100 0.00 7.00 2.0 8.0 12.0 1.500 150 -0.18 6.82 4.0 6.0 14.0 2.330 233 -0.37 6.63 6.0 4.0 16.0 4.000 400 -0.60 6.40 8.0 2.0 18.0 9.000 900 -0.95 6.05 9.0 1.0 19.0 19.000 1900 -1.28 5.72 9.5 0.5 19.5 39.000 3900 -1.59 5.41 Concentration of added acid = number of milliequivalents of strong acid added divided by the volume of the solution. Negative values refer to addition of strong base. For simplicity, volume of added acid and base is assumed to be negligible. WSUSOM Medical Physiology Rossi-Acid Base Physiology Page 8 of 15 Body fluid buffering and titration For example, if 4 mmol 4 mEq) of a strong acid, HCl is added to 1 liter of the imidazole solution in a beaker, what will be the pH of the resulting solution? (move from orange to blue box) Assume that no change in volume and that all the added base combines with HIm+ and converts it to Im + water. Since the initial solution contains 10 mmol Im and 10 mmol HIm+, the basic form Im will decrease by 4 mmol to 6 mmol and the conjugate acid HIm+ will increase by 4 mmol to 14 mmol. The [H+] concentration can be estimated from the table to be 233 nEq, giving a pH = 6.63. Note that the table does not go beyond 9.5 mEq of acid or base added. This is because the approximation that all added acid (or base) combines with buffer is grossly inaccurate when the total concentration of added acid (or base) exceeds the initial concentration of buffer in the acid (or base) form…in this case 10 mEq (10 mmol). If we add a strong base instead…. For example, if 4 mmol of a strong base, NaOH is added to 1 liter of the imidazole solution in a beaker, what will be the pH of the resulting solution? Assume that no change in volume and that all the added base combines with HIm+ and converts it to Im + water. Since the initial solution contains 10 mmol Im and 10 mmol HIm, the acid form HIm+ will decrease by 4 mmol to 6 mmol and the conjugate base Im will increase by 4 mmol to 14 mmol. [H+] concentration can be estimated from the table to be 42.9 nEq, giving a pH = 7.37. This figure shows the titration curve in the table on the previous slide in graphic form. Note that the rectangle shows the area of typical physiological values. This area will be represented in what is called the Davenport diagram. WSUSOM Medical Physiology Rossi-Acid Base Physiology Page 9 of 15 Body fluid buffering and titration Body Acid-Base State: Review Rather large amounts of acid or base can be added to the body without producing lethal changes in pH because the body contains large amounts of buffer substances. Most of the buffers are located intracellularly; but this process is relatively slow. Intracellular buffers are composed mostly of proteins and phosphates. Hemoglobin in red cells, though intracellular, quickly buffers acids or bases added to ECF. Hemoglobin has a high histidine content (36 histidines per hemoglobin tetramer). Histidine has the imidazole group. Thus, hemoglobin accounts for nearly 90% of the buffer value of blood. Hemoglobin as a Buffer High histidine content = 36 histidines in a tetramer Most of CO2 carried in blood is as HCO3- with Most of the H+ is in the form of HIm+ in RBCs All measurements of pH in blood are corrected to 100% saturation of Hgb with O2 ECF also includes buffers such as plasma proteins and phosphates. Intracellularly, proteins and phosphates also act as buffers of the intracellular pH. Bone is a large sink for acids/bases, very slow buffer and participates more in conditions of chronic acidosis (e.g., kidney failure). Most of the CO2 carried by blood is in the form of HCO3- with the H+ ions being carried in the form of HIm+ by the histidines in hemoglobin. When we discuss acid/base balance, we assume that extracellular blood pH reflects intracellular pH, and that blood pH reflects pH in all parts of the body. These are very big assumptions but they are what we have to work with as physicians. The HCO3-/CO2 buffer system (carbonic acid) is very important even though the pK=6.1 (not that close to physiologic). This buffer pair is important because this system is under the dual control by the lungs and the kidneys. It is useful to know where the numbers we use here come from (but do NOT memorize this derivation). WSUSOM Medical Physiology Rossi-Acid Base Physiology Page 10 of 15 Body fluid buffering and titration CO2 System Concentration of dissolved CO2 = [CO2]dis [CO2]dis µ pCO2 [CO2]dis = 0.03 mM/mmHg * pCO2 pCO2 = 40 mmHg [CO2]dis = 0.03 * 40 = 1.2 mM CO2/HCO3- system 1. Dissolved CO2: all gases dissolve to some extent in water. The concentration of dissolved CO2 is plasma [CO2]dis is proportional to the partial pressure of CO2 in the plasma, pCO2 If pCO2 is given in mmHg and [CO2]dis in mM then [CO2]dis = 0.03 * pCO2 Since arterial blood is normally equilibrated with alveolar air, pCO2, is equal to the partial pressure of CO2 in alveolar air, normally 40 mmHg. Thus, [CO2]dis = 0.03 * 40 = 1.2 mM Hydration of CO2 2. Hydration of CO2 CO2 reacts reversibly with water to form carbonic acid (1st equation above). The equilibrium is typically far to the left. At body temp, there are about 500 CO2 molecules for every H2CO3 molecule. The hydration reaction is SLOW. It is catalyzed by carbonic anhydrase in the body. Carbonic anhydrase is found in critical locations involved such as - RBCs - transporting epithelia (renal tubular cells, choroid plexus of the brain) 3. The overall reaction is shown in the 2nd equation above. Once H2CO3 is formed, it rapidly dissociates to H+ and HCO3- Hence, the useful relationship is that among the quantities pCO2, pH, and [HCO3-] Assuming water is constant, the equilibrium condition Ka’ is given in the last equation. WSUSOM Medical Physiology Rossi-Acid Base Physiology Page 11 of 15 Body fluid buffering and titration Henderson-Hasselbach Equation: now filling in the numbers Ka’ for this reaction is 8 x 10-7; so that, pKa’ = 6.1. By taking the logarithm of both sides and solving for pH we get the Henderson-Hasselbach equation (box). The highlighted equation (box) gives the relationship between the three physiologically important quantities pCO2, pH, and [HCO3-]. If any two of these quantities are measured, the third can be calculated! If one of the two values changes, the third will change in a predictable manner. (E,g, at a given HCO3- and pCO2 there can be one and only one pH. If the pCO2 increases and the HCO3- stays the same, pH will decrease.) Henderson Hasselbach is ALWAYS obeyed!!! Common misconception: Henderson Hasselbach does NOT tell us the acid base disorder. Even in severe and complex disorders of acid base balance, the relationship among pCO2, pH, and [HCO3-] remains governed by the chemical equilibrium among these three species. The acid base disturbance and changes in these parameters (pCO2, pH, and [HCO3-]) are governed by physiological processes (and constraints), but they will always related to each other by Henderson Hasselbach. The Davenport diagram is merely the section in the box of the titration curve for imidazole. The diagram can be used to assess buffering WSUSOM Medical Physiology Rossi-Acid Base Physiology Page 12 of 15 Body fluid buffering and titration Several points: 1. The curve is steepest in the neighborhood of pH = 7.0, where pH = pK. Corollary: When the pH is closest to the pK there is the least change in pH for each mEq of added acid (or base). 2. The titration curve for imidazole is approximately linear between pH 6.5 and 7.5. Since the range of body fluid pH values compatible with life is less than one pH unit, titration curves for body Imidazol titration curve fluids can be accurately approximated by straight lines. 3. The HCO3- concentration is given on the Y-axis 4. The pH is given on the X-axis 5. The curved lines are called isobars and are constructed by drawing smooth curves through the points where pCO2 is the constant. Buffering In the Body – Using the Davenport Diagram For any two measured parameters pH, pCO2 and [HCO3-], the third can be calculated. Let’s pretend….. pCO2 isobars: titration curves at fixed pCO2 The figure above shows the titration curves of a carbonic acid/bicarbonate solution (such as ECF or whole blood) at various, fixed pCO2 values. The solution is titrated by adding strong non-carbonic acid or base in increments to the solution. Consider the curve for pCO2 = 40 mmHg. HCl or NaOH is added and the solution allowed to equilibrate. Point O is the normal point for humans at sea level: pCO2 = 40 mmHg WSUSOM Medical Physiology Rossi-Acid Base Physiology Page 13 of 15 Body fluid buffering and titration [HCO3-] = 24 mM pH = 7.40 Point A is reached by adding 5 mEq of strong acid (HCl) to each liter of solution with the CO2 held constant at 40 mmHg. Very nearly all the added HCl combines with NaHCO3 to form CO2, H2O, and NaCl: [HCO3-] decreases by 5 mEq to a final value of 19 mM (mEq) the pH calculated from Henderson-Hasselbach is now 7.30. Point B is reached by adding 5 mEq of strong base (NaOH) to 1 liter of the original solution. [HCO3-] increases by 5 mEq to a final value of 29 mM (mEq) the pH is now 7.48 Reaction of carbonic acid with buffers When carbonic acid is added to the solution by increasing dissolved CO2 (thereby increasing pCO2), the main buffering reaction is: H2CO3 + Hgb-Im « Hgb-HIm+ + HCO3- WSUSOM Medical Physiology Rossi-Acid Base Physiology Page 14 of 15 Body fluid buffering and titration where Hgb-Im represents titratable protein buffers, mostly imidazole on histidine in hemoglobin (Hgb) and plasma proteins and HCO3- is the conjugate base of H2CO3 This reaction shows that the titration curve of ECF with carbonic acid can be represented on the pH - [HCO3-] plane: For every H+ ion that is buffered by Hgb-Im, an HCO3- ion appears. (I am using Hgb or Hgb-Im here to emphasize and remind us that the imidazole is in the hemoglobin molecule; but often I will not use the Hgb to save space.) Titration of Carbonic Acid: Representaion on the pH- [HCO3-] Plane The titration curve of body fluids on the pH - [HCO3-] diagram: The straight line drawn through the normal Point O and has a slope = -12 mEq/pH This straight line is a good approximation for the acid base state of normal humans breathing different concentrations of CO2. For whole blood the line is steeper due to a higher [Hgb] than in plasma alone. For example, doubling pCO2 from 40 to 80 mmHg (Point A) increases [HCO3-] from 24 to 27 mEq (line L1), which is 3 mEq, and lowers the pH to 7.15. This 3 mEq represents the increase in [HCO3-] which equals the amount of added carbonic acid. If only bicarbonate buffers were present (no Hgb-Im) the pH would fall to 7.10 rather than 7.15. (This seems like a small difference, but in your clinical years you will find that even 0.05 pH points can make a BIG difference). Titration (buffering) of Body Fluids of Non-Carbonic Acids Non-carbonic (nonvolatile) acid is buffered by both – HCO3- – Proteins Therefore, D [HCO3- ] ¹ total H+ added Titration of body fluids with non-carbonic acids = metabolic disorders Non-carbonic acid is buffered by both HCO3- and by proteins. WSUSOM Medical Physiology Rossi-Acid Base Physiology Page 15 of 15 Body fluid buffering and titration The addition of a small amount of strong non-carbonic acid such as HCl to a buffered solution results in the combination of virtually all added H+ ions with the buffers. For body fluids (e.g, ECF), acid is buffered by the Im form of imidazole and the HCO3- to form carbonic acid. The reactions are shown above. The added non carbonic acid is buffered by both bicarbonate and hemoglobin (Hgb). Therefore, the change in [HCO3-] does NOT represent the total amount of added acid. Titration with Non-carbonic Acid: Base Deficit Determination of the amount of added non-carbonic acid (or base): Point O shows the initial condition. Point A shows the condition after an unknown amount of non-carbonic acid is added. (Note the pCO2 is kept constant in this scenario (move down the isobar = 40 mmHg). Most of the H+ ions combine with HCO3- to form CO2 and H2O. The remainder combine with Hgb and plasma proteins. L2 represents the change in [HCO3-], that is, the amount of acid buffered by HCO3-. L1 represents the amount of non-carbonic acid buffered by Hgb and plasma proteins. The vertical distance from point A up to the body titration line, L2 + L1 = the amount of added non-carbonic acid. This distance is called the base deficit. In the example above, base deficit = L2 + L1 = 11 mEq + 3 mEq = 14 mEq. Assuming that the addition of acid is recent and most of the body buffering is in the ECF, if the ECF has a volume of 10 liters, then the total amount of HCl added to the ECF = base deficit * ECF volume = 14 mEq/L * 10 L = 140 mEq acid. Likewise, points above the body titration line have a base excess.