6-Bone-2324.pptx
Document Details
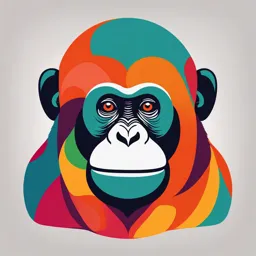
Uploaded by UserFriendlyLepidolite13
Full Transcript
BOTWEEFSEL Gemineraliseerd weefsel met mechanische en metabole functies (30% collagene fibrillen en 60% kalkzouten en cellen, bloedvaten en water) Sterk maar toch dynamisch met constante remodellering Functies: - Protectie van vitale organen - Ondersteunen weke delen - vormt de mergholtes met beenm...
BOTWEEFSEL Gemineraliseerd weefsel met mechanische en metabole functies (30% collagene fibrillen en 60% kalkzouten en cellen, bloedvaten en water) Sterk maar toch dynamisch met constante remodellering Functies: - Protectie van vitale organen - Ondersteunen weke delen - vormt de mergholtes met beenmerg (aanmaak bloedcellen) - reservoir voor calcium, fosfaat -hefbomen systeem: overbrengen van krachten gegenereerd in de spieren, omzetten naar beweging Gespecialiseerd bindweefsel: >Intercellulair verkalkt materiaal (botmatrix) 3 types cellen > osteocyten (in de matrix) (8-1) > osteoblasten (synthetiseren de organische matrix) > osteoclasten (multinucleaire cellen, resorberen en remodelleren) Osteogenische cellen liggen aan de rand van het botweefsel Verbindingen tussen osteocyten en bloedcapillairen: canaliculi > perforeren de matrix (8-2) Bindweefsellaag aan binnen en buitenzijde van alle beenderen > aan het interne oppervlakte: endost > aan het externe oppervlakte: periost Figure 8–3. Events that occur during intramembranous ossification. Osteoblasts are synthesizing collagen, which forms a strand of matrix that traps cells. As this occurs, the osteoblasts gradually differentiate to become osteocytes. The lower part of the drawing shows an osteoblast being trapped in newly formed bone matrix. Bone tissue. Newly formed bone tissue decalcified for sectioning and stained with trichrome in which the collagen-rich ECM appears bright blue. The tissue is a combination of mesenchymal regions (M) containing capillaries, fibroblasts, and osteoprogenitor stem cells and regions of normally calcified matrix with varying amounts of collagen and the three major cell types found in all bone tissue. Bone-forming osteoblasts (Ob) differentiate from osteoprogenitor cells in the periosteum and endosteum, and cover the surfaces of existing bone matrix. Osteoblasts secrete osteoid rich in collagen type I, but also containing proteoglycans and other molecules. As osteoid undergoes calcification and hardens, it entraps some osteoblasts that then differentiate further as osteocytes (Oc) occupying lacunae surrounded by bony matrix. The much less numerous large, multinuclear osteoclasts (Ocl), produced by the fusion of blood monocytes, reside on bony surfaces and erode the matrix during bone remodeling. (400X; Mallory trichrome) Osteoblasts, osteocytes, and osteoclasts. (a)Diagram showing the relationship of osteoblasts to the newly formed matrix called “osteoid,” bone matrix, and osteocytes. Osteoblasts and most of the larger osteoclasts are part of the endosteum covering the bony trabeculae. (b)The photomicrograph of developing bone shows the location and morphologic differences between active osteoblasts (Ob) and osteocytes (Oc). Rounded osteoblasts, derived from progenitor cells in the adjacent mesenchyme (M), cover a thin layer of lightly stained osteoid (Os) on the surface of the more heavily stained bony matrix (B). Most osteoblasts that are no longer actively secreting osteoid will undergo apoptosis; others differentiate either as flattened bone lining cells on the trabeculae of bony matrix or as osteocytes located within lacunae surrounded by bony matrix. (X300; H&E) BOT CELLEN Osteoblasten (8-3) Ontstaan uit osteoprogenitorcellen die voorkomen in het endost en periost > synthetiseren de organische componenten van de matrix >> type I collageen >> proteoglycanen >> glycoproteïnen > rol in de dispositie van inorganische componenten??? > exclusief aan het oppervlak van botweefsel >> aaneengesloten rijen (epitheliumachtige organisatie, osteoblastenzoom) > actieve osteoblasts >> cuboidale tot cylindrische vorm >> synthese van export proteïnen >> gepolariseerde cellen >>> secretie van matrix componenten aan het celoppervlak in contact met de botmatrix = osteoïd (nog onverkalkt - heldere zone) >>>> afzetten van calcium zouten > passieve osteoblasten >> afgeplat uiterlijk – botrandcellen of grensvlakcellen Contact met osteocyten en grensvlakcellen door cytoplasmatische uitlopers Osteocyten - Ontstaan uit osteoblasten (volwassen botcellen) > in lacunae van de verkalkte matrix(1 cel/lacuna) >> tussen lamellae - cytoplasmatische uitlopers in de canaliculi > gap junctions tussen uitlopers van verschillende cellen >> molecule transport -Kleine hoeveelheid extracellulair substantie tussen de osteocyt en de matrix - osteocyten: > relatief weinig RER > klein GC > meer gecondenseerd nucleaire chromatine (afgeplatte kern) - Functie: > onderhoud van de botmatrix >remodellering via gewijzigde vloeistofstroom in de canaliculi Osteoclasten (8-4) (8-5) > grote, onregelmatige gevormde, beweeglijke cellen >meerkerning (5 tot 50!). Fusie o.i.v.van stoffen uitgescheiden door osteoblasten of osteocyten Produceren uithollingen in de bot matrix: Howship’s lacunae Actieve osteoclast: > ruffled border: onregelmatig en geplooid >> naar het oppervlak van de botmatrix gericht > heldere zone: rond de ruffled border >> organelvrije zone >> rijk aan actine filamenten (hechting aan het bot via integrines) >>>> vorming van een micro-omgeving voor bot resorptie >Secretie van collagenase in het subosteoclastencompartiment >> afbraak van collageen > pompt protonen in de subcellulaire ruimte >>oplossen van calciumkristallen > activiteit geregeld door >> cytokines (gesecreteerd door osteoblasten) >> hormonen (calcitoninne) Figure 8–1. Components of bone. The diagram shows an overview of the basic features of bone, including the three key cell types osteocytes, osteoblasts, and osteoclasts; their usual locations; and the typical lamellar organizations of bone. Osteoblasts secrete the matrix which then hardens by calcification, trapping the differentiating cells now called osteocytes in individual lacunae. Osteocytes maintain the calcified matrix and receive nutrients from blood vessels via very small channels through the matrix called canaliculi. Osteoclasts are monocyte—derived cells in bone which are important in bone remodeling. Figure 8–3. Mineralization in bone matrix. From their ends adjacent to the matrix, osteoblasts secrete type I collagen, several glycoproteins, and proteoglycans. Some of these factors, notably osteocalcin and certain glycoproteins, bind Ca2+ with high affinity, thus raising the local concentration of these ions. Osteoblasts also release very small membrane—enclosed matrix vesicles with which alkaline phosphatase and other enzymes are associated. These enzymes hydrolyze PO—4 ions from various macromolecules, creating a high concentration of these ions locally. The high ion concentrations cause crystals of CaPO4 to form on the matrix vesicles. The crystals grow and mineralize further with formation of small growing masses of hydroxyapatite [Ca10(PO4)6(OH)2] which surround the collagen fibers and all other macromolecules. Eventually the masses of hydroxyapatite merge as a confluent solid bony matrix as calcification of the matrix is completed. Figure 8–9. An osteon. In preparations of dried, ground bone osteons can be seen with lacunae (L) situated between concentric lamellae and interconnected by fine canaliculi (C). Although it is not apparent by light microscopy, each lamella consists of multiple parallel arrays of collagen fibers. In adjacent lamellae, the collagen fibers are oriented in different directions. The presence of large numbers of lamellae with differing fiber orientations provides the bone with great strength, despite its light weight. Only remnants of the osteocytes (O) in some lacunae and of the osteonic canal’s contents are seen in ground bone. In living tissue osteocytic processes connected via gap junctions are present in successive canaliculi, making cells in all the lamellae in communication with the blood vessels in the central canal. X500. Figure 8–5. Osteoclasts and their activity. The osteoclast is a large cell with several nuclei derived by the fusion in bone of several blood—derived monocytes. (a): Microscopic section showing two osteoclasts (arrows) digesting or resorbing bone matrix in resorption bays on the matrix surface. X400. H&E Figure 8–1. Section of bone tissue showing an osteocyte with its cytoplasmic processes surrounded by matrix. The ultrastructure of the cell nucleus and cytoplasm is compatible with a low level of protein synthesis. Figure 8–2. Photomicrograph of dried bone ground very thin. The lacunae and canaliculi filled with air deflect the light and appear dark, showing the communication between these structures through which nutrients derived from blood vessels flow. Medium magnification. Osteocytes in lacunae. (a)TEM showing an osteocyte in a lacuna and two dendritic processes in canaliculi (C) surrounded by bony matrix. Many such processes are extended from each cell as osteoid is being secreted; this material then undergoes calcification around the processes, giving rise to canaliculi. (X30,000) (b)Photomicrograph of bone, not decalcified or sectioned, but ground very thin to demonstrate lacunae and canaliculi. The lacunae and canaliculi (C) appear dark and show the communication between these structures through which nutrients derived from blood vessels diffuse and are passed from cell to cell in living bone. (X400; Ground bone) (c)SEM of nondecalcified, sectioned, and acid-etched bone showing lacunae and canaliculi (C). (X400) (Figure 8–5c, used with permission from Dr Matt Allen, Indiana University School of Medicine, Indianapolis.) Figure 8–4. Section showing 3 osteoclasts (arrows) digesting bone tissue. The osteoclast is a large cell with several nuclei and a ruffled border close to the bone matrix. Note the clear compartment where the process of bone erosion occurs. This compartment is acidified by a proton pump localized in the osteoclast membrane. It is the place of decalcification and matrix digestion and can be compared to a giant extracellular lysosome. Chondroclasts found in eroded regions of epiphyseal calcified cartilage are similar in shape to osteoclasts. Figure 8–5. Bone resorption. Lysosomal enzymes packaged in the Golgi complex and hydrogen ions produced are released into the confined microenvironment created by the attachment between bone matrix and the osteoclast’s peripheral clear zone. The acidification of this confined space facilitates the dissolution of calcium phosphate from bone and is the optimal pH for the activity of lysosomal hydrolases. Bone matrix is thus removed and the products of bone resorption are taken up by the osteoclast’s cytoplasm, probably digested further, and transferred to blood capillaries. Osteoclasts and their activity. Osteoclasts are large multinucleated cells that are derived by the fusion in bone of several blood-derived monocytes. (a) Photo of bone showing two osteoclasts (Ocl) digesting and resorbing bone matrix (B) in relatively large resorption cavities (or Howship lacunae) on the matrix surface. An osteocyte (Oc) in its smaller lacuna is also shown. (X400; H&E) (b)Diagram showing an osteoclast’s circumferential sealing zone where integrins tightly bind the cell to the bone matrix. The sealing zone surrounds a ruffled border of microvilli and other cytoplasmic projections close to this matrix. The sealed space between the cell and the matrix is acidified to ~pH 4.5 by proton pumps in the ruffled part of the cell membrane and receives secreted matrix metalloproteases and other hydrolytic enzymes. Acidification of the sealed space promotes dissolution of hydroxyapatite from bone and stimulates activity of the protein hydrolases, producing localized matrix resorption. The breakdown products of collagen fibers and other polypeptides are endocytosed by the osteoclast and further degraded in lysosomes, while Ca2+ and other ions are released directly and taken up by the blood. (c)SEM showing an active osteoclast cultured on a flat substrate of bone. A trench is formed on the bone surface by the slowly migrating BOTMATRIX (1) Inorganische bestanddelen: > calcium > fosfor > bicarbonaat > citraat > magnesium > kalium > natrium Ca en P vormen hydroxy-apatietkristallen Ca10(PO4)6(OH)2 Ook Amorf calciumfosfaat De kristallen liggen als platen langs de collagene vezels en zijn omgeven door grondsubstantie. De oppervlakkige ionen van de kristallen zijn gehydrateerd (2) Organic bestanddelen > collageen type 1 > grond substantie:>>glycosaminoglycanen >> proteoglycaan aggregaten >> structurele glycoproteïnen Combinatie van mineralen en collageen => hardheid en stevigheid PERIOST & ENDOST (8-6) Bindweefselvlies Periost: vezelige bindweefsel laag (vooral buitenzijde) > Vezels van Sharpey: collageenvezels die de botmatrix binnendringen >> binden het periost op het bot Debinnenste laag bevat osteoprogenitor cellen > fibroblastachtige cellen >> delen door mitosen >> differentiëren in osteoblasten Endost: begrenst de holtes in het bot > één enkele laag van platte osteoprogenitor cellen > heel weinig bindweefsel Periost en endost bevatten veel bloedvaten die via kanalen van Volkmann het bot binnendringen Figure 8–6. Periosteum and endosteum. Section through a thin portion of the wall of a long—bone diaphysis showing both periosteum (P) and endosteum (E). The periosteum covers bone and provides a supply of osteoprogenitor cells which become osteoblasts for new bone formation. These cells are located in the inner, more cellular layers of the periosteum, next to the bone matrix. Externally the periosteum consists of a thick layer of more fibrous, dense connective tissue, which merges with ligaments and other connective tissues. Perforating fibers fastening the periosteum to the bone matrix are not seen in routine light microscope preparations. The periosteum has a good blood supply, but the cavities lined by endosteum, the marrow cavities, are very rich in blood sinuses and blood—forming tissue. X100. H&E. SOORTEN BOTWEEFSEL (8-7) Compact bot (aaneengesloten zonder holtes)> < spongieus bot Microscopisch hebben beide vormen dezelfde lamellaire opbouw Microscopisch kunnen echter 2 types onderscheiden worden primair > <secundair onrijp > <rijp gevlochten > <lamellair Figure 8–1. Components of bone. The diagram shows an overview of the basic features of bone, including the three key cell types osteocytes, osteoblasts, and osteoclasts; their usual locations; and the typical lamellar organizations of bone. Osteoblasts secrete the matrix which then hardens by calcification, trapping the differentiating cells now called osteocytes in individual lacunae. Osteocytes maintain the calcified matrix and receive nutrients from blood vessels via very small channels through the matrix called canaliculi. Osteoclasts are monocyte—derived cells in bone which are important in bone remodeling. Primair bot: Onrijpe vorm van botweefsel dat embryonaal eerst aangelegd wordt maar ook bij herstel van fracturen > meestal tijdelijk (wordt vervangen door > uitzonderingen: >> tandalveolen >> de aanhechtingen van bepaalde pezen secundair) > collagene vezels in allerlei richtingen (gevlochten) > Bevat minder mineralen > Bevat veel osteocyten Secundair bot: (8-6), (8-8) Georganiseerd in systemen van Havers of osteonkanalen > collageen gerangschikt in lamellen >> parallele >> concentrische >>> rond een kanaal met: bloedvaten, zenuwen, en losmazig bindweefsel > Lacunae (met osteocyten) tussen en in de lamellen >In één lamel: collageen vezels parallel aan elkander Kitlaag (8-8) > amorf materiaal rond het osteon >> gemineraliseerde matrix >> weinig collageen >> voornamelijk glycoproteïnen In compact bot: typische organisatie Binnenste en buitenste generale lamellen Evenwijdig aan het botoppervlak > Buitenste generale lamellae >> onmiddellijk onder het periost > Binnenste generale lamellae: >>tegen het endost Meer buitenste dan binnenste generale lamellae > Systemen van Havers >> liggen tussen de buitenste en binnenste lamellen >interstitiële (intermediare) lamellae (8-10), tussen de systemen van Havers >> veelhoekige vorm van parallele lamellae Figure 8–6. Schematic drawing of the wall of a long-bone diaphysis showing 3 types of lamellar bone: haversian system and outer and inner circumferential lamellae. (For interstitial lamellae, see Figure 8–10.) The protruding haversian system on the left shows the orientation of collagen fibers in each lamella. At the right is a haversian system showing lamellae, a central blood capillary (there are also small nerves, not shown), and many osteocytes with their processes. Systeem van Havers : lang, parallel aan de diafyse as centraal kanaal omgeven door 4-20 > concentrische lamellae communicatie door kanalen van Volkmann >> tussen mergholte, periost en , systemen van Havers >> perforeren de lamellae In de systemen van Havers: >collagene vezels parallel binnenin de lamellen => helicale organisatie (8-6) > Verschillende schroef tussen de lamellae! Botweefsel wordt constant geremodelleerd => grote variabiliteit in de diameter van de kanalen van Havers > elk systeem wordt gevormd door opeenvolgende afzettingen van lamellae > start aan de periferie >> jonge systemen = groot kanaal Lamellar bone: Perforating canals and interstitial lamellae. (a)Transverse perforating (Volkmann) canals (P) connecting adjacent osteons are shown in this micrograph of compact lamellar bone. Such canals “perforate” lamellae and provide another source of microvasculature for the central canals of osteons. Among the intact osteons are also found remnants of eroded osteons, seen as irregular interstitial lamellae (I). (Ground bone; X100) (b)Diagram showing the remodeling of compact lamellar bone with three generations of osteons and their successive contributions to the formation of interstitial lamellae. The shading indicates that successive generations of osteons have different degrees of mineralization, with the most newly formed being the least mineralized. Remodeling is a continuous process that involves the coordinated activity of osteoblasts and osteoclasts, and is responsible for adaptation of bone to changes in stress, especially during the body’s growth. Development of an osteon. During remodeling of compact bone, osteoclasts act as a cutting cone that tunnels into existing bone matrix. Behind the osteoclasts, a population of osteoblast progenitors enters the newly formed tunnel and lines its walls. The osteoblasts secrete osteoid in a cyclic manner, producing layers of new matrix (lamellae), and trapping some cells (future osteocytes) in lacunae. The tunnel becomes constricted with multiple concentric layers of new matrix, and its lumen finally exists as only a narrow central canal with small blood vessels. The dashed lines in (a) indicate the levels of the structures shown in cross section (b). An x-ray image (c) shows the different Figure 8–7. A: Thick section of bone illustrating the cortical compact bone and the lattice of trabeculae of cancellous bone. (Courtesy of DW Fawcett.) B: Section of cancellous (spongy) bone with its characteristic random disposition of collagen fibers. Picrosirius–polarized light (PSP) stain. Low magnification. Figure 8–8. Schematic drawing of 2 osteocytes and part of a haversian system. Collagen fibers of contiguous lamellae are sectioned at different angles. Note the numerous canaliculi that permit communication between lacunae and with the haversian canals. Although it is not apparent in this simplified diagram, each lamella consists of multiple parallel arrays of collagen fibers. In adjacent lamellae, the collagen fibers are oriented in different directions. The presence of large numbers of lamellae with differing fiber orientations provides the bone with great strength, despite its light weight. (Redrawn and reproduced, with permission, from Leeson TS, Leeson CR: Histology, 2nd ed. Saunders, 1970.) Figure 8–9. Lamellar (secondary) bone in which the collagen fibers can be parallel to each other (at left) or organized concentrically around neurovascular channels, to constitute the haversian systems, or osteons (in most of the figure). Among the numerous haversian systems are some interstitial lamellae. PSP stain. Low magnification. Lamellar bone. Two photographs of the same area of an unstained section of compact bone, showing osteons with concentric lamellae around central canals. Lamellae are seen only faintly by bright-field microscopy (a), but they appear as alternating bright and dark bands under the polarizing light microscope (b). Bright bands are due to birefringence from the highly ordered collagen fibers in a lamella. Alternating bright and dark bands indicate that fibers in successive lamellae have different orientations, an organization that makes lamellar bone very strong. (Both X100) (Used with permission from Dr Matt Allen, Indiana University School of Medicine, Indianapolis.) Figure 8–11. Section of a haversian system, or osteon. Note the alternation of clear and dark circles resulting from the alternation in the direction of the collagen fibers. The collagen fibers appear bright when cut longitudinally and dark when cross-sectioned. In the center of the osteon is a channel. PSP stain. Medium magnification. HISTOGENESE 2 manieren (1)Endesmaal (desmaal): botweefsel direct uit bindweefsel gevormd (botafzetting in de vorm van een band): mineralisatie van matrix gesecreteerd door osteoblasten (2)Enchondraal: indirecte vorming doordat kraakbeen (chondros) vervangen wordt door bot Telkens vorming van primair bot dat vervangen wordt door secundair bot Ifv plaats van de botvorming Intramembraneus versus chondrale (perichondrale en enchondrale) INTRAMEMBRANEUZE botvorming Bij sommige schedelbeenderen. Start in een bindweefselgebied dat zich voordoet als vlies of membraan Bindweefsel wordt omgezet in botweefsel (soort endesmale botvorming) Cellen differentiëren tot osteoblasten Osteoblasten vormen osteoïd dat verkalkt tot botmatrix Ingesloten osteoblasten differentiëren tot osteocyten Trabekels groeien en vormen een 3 dimensioneel netwerk CHONDRALE Botvorming Perichondraal (om het kraakbeen) >< enchondraal (in het kraakbeen) Opbouwfasen: 1. Vorming van een kraakbenig skelet 2. Perichondrale botvorming rond de diafyse van het kraakbenige pijpbeen 3. Enchondrale botvorming centraal in de kraakbenige diafyse (primaire botvormingscentrum) 4. Enchondrale botvorming in beide epifysen (secundaire botvormingscentra) 5. Enchondrale botvorming in beide epifysaire schijven Figure 8–13. Formation of a long bone on a model made of cartilage. Hyaline cartilage is stippled; calcified cartilage is black, and bone tissue is indicated by oblique lines. The 5 small drawings in the middle row represent cross sections through the middle regions of the figures shown in the upper row. Note the formation of the bone collar and primary and secondary ossification centers. Epiphyseal fusion with diaphysis, with disappearance of the epiphyseal cartilage, occurs at different times in the same bone. (Redrawn and reproduced, with permission, from Bloom W, Fawcett DW: A Textbook of Histology, 9th ed. Saunders, 1968.) 1. Vorming van het kraakbenig skelet Hyalien kraakbeenstuk wordt aangelegd (verkleinde versie van het te vormen pijpbeen) 2 epifysen (verdikkingen op het uiteinde) 1 diafyse (kraakbeenschacht) 2. Perichondrale botvorming (diafyse) >Uit bindweefsel (perichondrium) dat de diafyse omgeeft wordt botweefsel gevormd >> binnenste cellen van het perichondrium differentiëren tot een zoom van osteoblasten >>>zetten bot af tegen de kraakbenige diafyse Vorming van de botmanchet Omzetting van bindweefsel in bot = endesmale botvorming 3. Enchondrale botvorming in de kraakbenige diafyse: het primaire botvormingscentrum Hypertrofie van de kraakbeencellen in de diafyse (resorbtie van omringde matrix) Verkalking van de kraakbeenmatrix: destructie van kraakbeencellen met vorming van lacunes gescheiden door tussenschotten (septa) van verkalkte kraakbeentussenstof Vorming van de botvormingsknop (periostknop) : Osteoclasten doorbreken het perichondrale bot en delen van het kraakbeen worden afgebroken (chondroclasten). Bloedvaten en osteoprogenitorcellen kraakbeenmatrix in de Osteoblasten (uit osteoprogenitorcellen) zetten nieuw bot af tegen de resten van de verkalkte kraakbeenmatrix. De kraakbeenresten liggen in Figure 8–15. Schematic drawings showing the 3-dimensional shape of bone in the epiphyseal plate area. Hyaline cartilage is stippled; calcified cartilage is black, and bone tissue is shown as yellow hatched areas. The upper drawing shows the region represented 3-dimensionally in the lower drawing. (Redrawn and reproduced, with permission, from Ham AW: Histology, 6th ed. Lippincott, 1969.) 4. Enchondrale botvorming in beide epifysen: de secundaire botvorming Secundaire botvormingscentra in beide epifysen: >Binnendringen van bloedvaten en osteoprogenitorcellen (die differentiëren tot osteoblasten) > enchondrale bot afzetting op het kraakbenig skelet 5.Enchondrale botvorming in beide epifysaire schijven Twee gebieden met kraakbeen blijven over: > het gewrichtskraakbeen >epifysaire schijven of groeischijven (verbinding epifysen met diafysen). Het kraakbeen in de epifysaire schijven is verantwoordelijk voor de lengtegroei van de beenderen en verdwijnt bij volwassenen. Enchondrale botvorming, gekoppeld aan 5 kenmerkende zones van de epifysaire schijven. (1) Rustzone: normaal hyalien kraakbeen (2) Proliferatiezone: snelle deling van chondrocyten en vorming van isogene groepen (3) Zwellingszone van hypertrofisch kraakbeen: sterk gezwollen kraakbeencellen, bevatten veel glycogeen en resorberen matrixmateriaal. De kraakbeenmatrix wordt teruggebracht tot dunne septa tussen de gezwollen isogene groepen (4) Verkalkingszone: verkalking van de kraakbeensepta en degeneratie van de kraakbeencellen (5) Botvormingszone: verbreking van de septa tussen de lacunes (in de lengterichting van het bot) = vorming van longitudinale tunnels. Bloedcapillairen + osteoprogenitorcellen dringen de tunnels binnen en na differentiatie zetten de osteoblasten enchondraal bot af tegen de septa van verkalkt kraakbeen (kenmerkend voor primair bot). Dit primaire bot wordt snel vervangen door secundair (lamellair) bot. Lengtegroei van lange pijpbeenderen: > Enchondrale botvorming onderaan de epifysaire schijf Diktegroei: >Endesmale botafzetting aan de buitenzijde van de botmanchet door osteoblasten in het periost. Lengte en diktegroei gaan gepaard met botafbraak aan de binnenkant en botafzetting aan de buitenkant waardoor de mergholte steeds groter wordt. Figure 8–16. Epiphyseal growth plate: locations and zones of activity. The large and growing primary ossification center in long bone diaphyses and the secondary ossification centers in epiphyses are separated in each developing bone by a plate of cartilage called the epiphyseal plate. (a): Epiphyseal plates can be identified in an x—ray of a child’s hand as marrow regions of lower density between the denser ossification centers. Cells in epiphyseal growth plates are responsible for continued elongation of bones until the body’s full size is reached. Developmental activities in the epiphyseal growth plate occur in overlapping zones with distinct histological appearances. (b): Moving from the epiphysis to the diaphysis, these zones include cells specialized for the following: (1) normally appearing hyaline cartilage, (2) cartilage with proliferating chondroblasts aligned in lacunae as axial aggregates, (3) degenerating cartilage in which the aligned cells are hypertrophic and the matrix condensed, (4) an area in which the chondrocytes have disappeared and the matrix is undergoing calcification, and (5) a zone in which blood vessels and osteoblasts have invaded the lacunae of the old cartilage, producing marrow cavities and osteoid for new bone. X100. H&E. Appositional bone growth. Bones increase in diameter as new bone tissue is added beneath the periosteum in a process of appositional growth. Also called radial bone growth, such growth in long bones begins with formation of the bone collar early in endochondral ossification. During radial bone growth, formation of new bone at the periosteal surface occurs concurrently with bone removal at the endosteal surface around the large medullary, enlarging this marrow-filled region and not greatly increasing the bone’s weight. Figure 8–14. Osteogenesis of long bones by endochondral ossification. Endochondral ossification forms most bones of the skeleton and occurs in the fetus in models made of hyaline cartilage (1). The process takes many weeks and major developmental stages include: formation of a bone collar around the middle of the cartilage model and degeneration of the underlying cartilage (2), followed by invasion of the resulting ossification center by capillaries and osteoprogenitor cells from the periosteum (3), osteoid deposition by the new osteoblasts, calcification of woven bone, and its remodeling as compact bone (4). This primary ossification center develops in the diaphysis, along the middle of each developing bone. Secondary ossification centers develop somewhat later by a similar process in the epiphyses. The primary and secondary ossification centers are separated by the epiphyseal plate (5) which provides for continued bone elongation. The two ossification centers do not merge until the epiphyseal plate disappears (6) when full stature is achieved. Figure 8–14. A small portion of an epiphyseal plate showing endochondral ossification. Remnants of calcified cartilage matrix (dark purple) appear covered by light-stained bone tissue. The newly formed bone is surrounded by osteoblasts. Some osteoblasts that were captured by the osseous matrix become osteocytes (arrowheads). Pararosaniline–toluidine blue (PT) stain. Medium magnification. Figure 8–15. Schematic drawings showing the 3-dimensional shape of bone in the epiphyseal plate area. Hyaline cartilage is stippled; calcified cartilage is black, and bone tissue is shown as yellow hatched areas. The upper drawing shows the region represented 3-dimensionally in the lower drawing. (Redrawn and reproduced, with permission, from Ham AW: Histology, 6th ed. Lippincott, 1969.) Figure 8–16. Photomicrograph of the epiphyseal plate, showing its 5 zones, the changes that take place in the cartilage, and the formation of bone. PT stain. Low magnification. Figure 8–17. Higher magnification of the epiphyseal plate showing details of the endochondral ossification. Cartilage matrix (purple) is covered by recently formed bone tissue (red). Bone marrow and fat cells fill up the space left by the new bone. Picrosirius-hematoxylin (PSH) stain. Medium magnification. Figure 8–18. Photomicrograph of endochondral ossification. In the upper region is a row of osteoblasts with intense cytoplasmic basophilia, a feature to be expected in cells synthesizing a glycoprotein (collagen). Note an osteoblast being captured in the bone matrix (arrow). Between the layer of osteoblasts and the calcified bone matrix is a pale region made of noncalcified bone matrix called osteoid. PT stain. Medium magnification. Figure 8–19. Section of endochondral ossification. The osseous matrix, rich in collagen type I, is specifically stained with picrosirius-hematoxylin. The cartilaginous matrix, containing collagen type II, stains blue with hematoxylin because of its high content of chondroitin sulfate. Medium magnification. Mechanisme van Calcificatie? > Start met afzetting van calcium zouten op collageen fibrilen >> geïnduceerd door proteoglycanen >> geïnduceerd door hoge-affiniteit calcium-binding glycoproteïnen >osteoblasten concentreren calcium zouten in intracytoplasmatische vesikels >> worden vrijgesteld aan het extracellular medium (matrix vesikels) > Betrokkenheid van alkalisch fosfatase >> geproduceerd door osteoblasten >> aanwezig in ossificatie zones BOT GROEI & REMODELLERING Groei: gedeeltelijke resorptie van gevormd weefsel met simultane afzetting van nieuw botweefsel. Meer afzetting dan afbraak Bot turnover is zeer hoog bij jonge kinderen (200X –volwassenen) INTERNE STRUCTUUR VAN BOT Veranderingen in functie van stress (mechanische belasting): Orthodontische remodellering: op de plaats van de druk botresorptie en aan de andere kant botafzetting: heroriënteren van botbalkjes en systemen van Havers ten opzichte van de mechanische belasting. METABOLE ROL VAN BOTWEEFSEL 99% van het totale calcium Bot calcium mobilisatie >transfer van ionen uit de kristallen naar de interstitiële vloeistof >> voornamelijk in spongieus bot >>> meer uit de “jongste “lamellae > Hormonen: >> parathyroïd hormoon (+) osteoclast resorptie via de osteoblasten die receptoren hebben voor PTH >>> productie van een osteoclast stimulerende factor >> calcitonine (schildklier) remt de activiteit van de osteoclasten FRACTUUR GENEZING (8-21) Bij botbreuk is de botmatrix beschadigd > local bloeduitstorting en vorming van bloedstolsel >botcellen in nabijheid van de breuk sterven af Macrofagen verwijderen (1) het bloedstolsel, (2) cellen, (3) de beschadigde matrix Periost en endost rond de fractuur proliferen >vormen weefsel rond de fractuur > Sluiten de fractuur Vorming van primair bot > enchondrale ossificatie > intramembraneuze ossificatie De onregelmatige trabeculae van het primaire bot verbinden tijdelijk de 2 extremiteiten = bot callus Krachten tijdens het herstel helpen de callus te remodelleren Het primaire bot wordt geleidelijk vervangen door secundair bot Figure 8–21. Repair of a fractured bone by formation of new bone tissue through periosteal and endosteal cell proliferation. Figure 8–18. Main features of bone fracture repair. Repair of a fractured bone occurs through several stages, but utilizes mechanisms already in place for bone remodeling. (1): Blood vessels torn within the fracture release blood which clots to produce a large fracture hematoma. (2): This is gradually removed by macrophages and replaced by a soft fibrocartilage—like mass of procallus tissue rich in collagen and fibroblasts. If broken, the periosteum re—establishes continuity over this tissue. (3): This soft procallus is invaded by regrowing blood vessels and osteoblasts. In the next few weeks the fibrocartilage is gradually replaced by trabeculae of primary bone, forming a hard callus throughout the original area of fracture. (4): The primary bone is then remodeled as compact and cancellous bone in continuity with the adjacent uninjured areas and fully functional vasculature is re—established. GEWRICHTEN Aaneensluiting van botten door bindweefselstructuren Synartrosen: bijna geen beweegelijkheid >< diartrosen > 3 types, gebaseerd op het soort weefsel die de botstukken aaneenzet (1) synostose: botten verbonden door botweefsel (schedel) (2) synchondrose: botten verbonden door hyalien kraakbeen (ribben op het sternum) beperkte beweeglijkheid (3) syndesmose: interosseuse ligament van straf bindweefsel (distale tibiofibulaire gewricht Diarthrosen: grote beweeglijkheid (8-22) (8-23) (8-24) (8-25) > ligamenten en een kapsel van bindweefsel verzorgen het contact >Het kapsel vormt een gesloten gewrichtsruimte die synoviale vloeistof bevat >> kleurloos, transparante, viskeuze vloeistof >> een dialysaat van bloedplasma >> bevat een hoge concentratie aan glycoproteïnen >>> geproduceerd door de cellen van de synoviale membraan Gewrichtkapsel bestaat uit een fibreuze laag (buitenzijde – verderzetting van periost – straf bindweefsel) en aan de binnenzijde een synoviale membraan (losmazig bindweefsel met macrofaagachtige cellen en fibroblastachtige cellen): De synoviale vloeistof voorziet nutrienten en zuurstof aan het avascular articulair kraakbeen De collageen vezels van het articulaire oppervlak van kraakbeen zijn gerangschikt in bogen: verdeling van krachten (8-24) Het articulair kraakbeen is veerkrachtig => absorptie van mechanische druk b.v. interverterbrale schijven (8-25) > proteoglycaan moleculen bevatten veel water >> biomechanische veer Druk: >>water uit de kraakbeenmatrix naar het synovial vocht >> na verwijdering van het water: reciproke electrostatische afstoting van de negatief geladen carboxyl en sulfaat groepen in de GAG moleculen >>> maken plaats zodat water opnieuw binnen kan na verdwijnen van de druk > Verplaatsing van water door bewegen van het gewricht >> essentieel voor de voeding van het kraakbeen >>> faciliteert eveneens de uitwisseling van O2 en CO2 tussen het synoviaal vocht en het articulair kraakbeen Figure 8–22. Schematic drawing of a diarthrosis. The capsule is formed by 2 parts: the external fibrous layer and the synovial layer (synovial membrane) that lines the articular cavity except for the cartilaginous areas (blue). Figure 8–19. Diarthroses, or synovial joints. Diarthroses are joints that allow free movement of the attached bones, such as knuckles, knees, and elbows. (a): Diagram shows the components of a diarthrosis that include (1) a capsule continuous with a covering ligament which inserts into the periosteum of both bones, (2) a synovial or joint cavity lined by synovial membrane and containing synovial fluid as a lubricant, and (3) the ends of epiphyses covered by articular cartilage Figure 8–23. Photomicrograph of a diarthrosis. Section of a guinea pig knee. PSH stain. Low magnification. Figure 8–24. Articular surfaces of a diarthrosis are covered by hyaline cartilage that is devoid of perichondrium. The upper drawing shows that in this cartilage, collagen fibers are first perpendicular and then bend gradually, becoming parallel to the cartilage surface. Deeply located chondrocytes are globular and are arranged in vertical rows. Superficially placed chondrocytes are flattened; they are not organized in groups. The lower left drawing shows the organization of collagen fibers in articular cartilage in 3 dimensions. Articular cartilage. (a) Articular surfaces of a diarthrosis are made of hyaline cartilage that lacks the usual perichondrium covering (X40; H&E). ( Het kapsel bestaat uit 2 lagen 8-26) (1) externe fibreuze laag (2) interne synoviale membraan De synoviale membraan bestaat uit 2 types cellen: (8-27) (3)fibroblast achtige cellen (B-Cellen) talrijker, goed ontwikkeld RER, GC. Zij secerneren eiwitten glycoproteïnen en hyaluronzuur) (4)macrofaag achtige cellen (A-Cellen) veel lysosomen, weining RER, goed ontwikkeld GC. De fibreuze laag bestaat uit straf bindweefsel Figure 8–26. Histologic structure of the synovial membrane, with its lining cells in epithelioid arrangement. There is no basal lamina between the lining cells and the underlying connective tissue. This tissue is rich in blood capillaries and contains a variable number of adipose cells (AD). (Reproduced, with permission, from Cossermelli W: Reumatologia Basica, Sarvier, 1971.) Figure 8–27. Schematic representation of the ultrastructure of synovial membrane. The two covering cell types are separated by a small amount of connective tissue ground substance. No basal lamina is seen separating the lining cells from the connective tissue. Blood capillaries are of the fenestrated type, which facilitates exchange of substances between blood and synovial fluid. Figure 8–25. Example of a special type of joint. Section of a rat tail showing in the center the intervertebral disk consisting of concentric layers of fibrocartilage (annulus fibrosus) surrounding the nucleus pulposus (see Chapter 7). The nucleus pulposus is formed by residual cells of the notochord immersed in abundant viscous intercellular matrix. PSH stain. Low magnification.